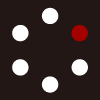
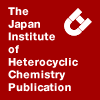
HETEROCYCLES
An International Journal for Reviews and Communications in Heterocyclic ChemistryWeb Edition ISSN: 1881-0942
Published online by The Japan Institute of Heterocyclic Chemistry
e-Journal
Full Text HTML
Received, 1st April, 2013, Accepted, 26th April, 2013, Published online, 2nd May, 2013.
DOI: 10.3987/COM-13-12721
■ Thermal and Photochemical Rearrangements of 3-Arylamino-2-phenyl-1H-inden-1-ones to N-Arylphthalimides
Masayuki Kawai, Eriko Sakanoshita, Motoko Akita, and Keiji Kobayashi*
Department of Chemistry, Faculty of Science, Josai University, 1-1 Keyakidai, Sakado, Saitama 350-0295, Japan
Abstract
Upon heating to above their melting temperatures, 3-arylamino-2-phenyl-1H-inden-1-ones and 2,2’-diphenyl-3,3’-bis(arylimino)- [2,2’-bi-1H-indene]-1,1’-dione undergo a skeletal rearrangement to afford N-arylphthalimides along with benzoic acid in the presence of atmospheric oxygen. The photoreaction of these compounds in acetonitrile also results in the formation of these products. The mechanism of these reactions, including the formation of a peroxyl radical followed by its conversion to a nitrogen-centered radical, is proposed.INTRODUCTION
The homolytic cleavage of carbon(sp3)-carbon(sp3) bonds is a fundamental reaction to generate carbon radicals. The ease of their dissociation is attributed both to steric repulsion around these C-C bonds and to the resonance stabilization of the dissociated carbon radicals, which may exist in thermal equilibrium with their precursors in solution.1 In the solid state, the resulting radical pairs usually undergo recombination because of restricted molecular and atomic displacements in crystalline lattices. Thus, a reversible change in color is induced in the solid state.2 Such homolytic dissociation of a C-C single bond in the solid state is realized thermally3 as well as by mechanical energy.4 To develop novel solid-state thermochromic compounds due to the generation of radical pairs and their recombination, we designed 2,2’-diphenyl-3,3’-bis(arylimino)-[2,2’-biindan]-1,1’-dione (2) as a potential candidate of such compounds by the structural modification of 2,2’-diphenyl-[2,2’-biindan]-1,1’,3,3’-tetrone (4) (Scheme 1), which shows mechanochemical dissociation of its central bond with the application of external pressure.5 In the course of the preparation of 2, its precursor compounds 3-arylimino-2-phenyl-1H-inden-1-ones (1a-d) have been found to show a novel thermal reaction in a melt with the participation of atmospheric oxygen. Furthermore, not only thermal but also photochemical reactions of these compounds were observed. In this paper, we describe novel thermal and photochemical rearrangement reactions of 1, 2 and 3 to afford N-arylphthalimides, which proceed via a stable carbon radical as an intermediate.
RESULTS AND DISCUSSION
1a-1d were readily obtained by the condensation of 2-phenyl-1,3-indandione with corresponding anilines in the presence of TiCl4. On the basis of the results of X-ray crystallographic analyses of 1b and 1c, the resulting condensation products were revealed to take an enamine form rather than an imino form, as represented by the structural forms of 1b and 1c (Figure 1). The NH hydrogen is hydrogen-bonded with the neighboring molecule at the carbonyl oxygen to form an infinite chain. The enamine structure is also retained in solution as proven by the occurrence of the NH proton at 7.2 ppm and by the absence of the proton attached to the tertiary carbon in its NMR spectra. Such isomeric structures were also revealed for the other derivatives 1a and 1d, judging from their NMR spectra.
Upon heating the solid samples of 1a-1d up to 250 °C, they melted gradually over a wide range, indicating decomposition. The products of this thermal decomposition were easily identified on the basis of the 1H NMR spectra of the resulting mixture, which involve signals identical to those of authentic samples of N-arylphthalimide and benzoic acid (Scheme 2). Figure 2 shows the 1H NMR spectrum of the reaction mixture from 1b as an example. The product distribution was estimated from the signal intensities to be approximately 84% N-tolylphthalimide and 75% benzoic acid. Similar reactions were also observed for the other derivatives to afford the oxidative rearrangement product and benzoic acid in an approximately 1:1 ratio and with 70-80% yields.
To gain further insight into the thermal reactions of 1b, thermal analysis by differential scanning calorimetry (DSC) was carried out within the temperature range from 180 to 330 °C in atmospheric and nitrogen environments. In nitrogen, 1b showed a sharp melting point at 250.8 °C with an endothermic energy of 137.2 J/g. However, in air, the DSC profile changed to a broad endothermic curve with a maximum heat flow at 248 °C. From this DSC profile, it can be seen that the reaction of 1b with oxygen takes place at approximately 245 °C and is completed before reaching its intrinsic melting point, observable in nitrogen. Thus, we assume that a plausible mechanism of these oxidative rearrangements includes successive ring opening and closure, as shown in Scheme 3. The peroxy radical, formed via the initial hydrogen abstraction by molecular oxygen,6 could be intramolecularly added to the C=N double bond to yield a new radical with a free valence on the N atom.7 The conversion of the resulting dioxetane intermediate to diketo compounds has been mentioned in the literature,8 and the intramolecular addition of a N-centered radical to the carbonyl group to afford a lactam ring has also been reported.9 The C2 carbon of the indenone framework is considered to be released as a benzoyl carbon, which can enter the chain reaction by hydrogen abstraction from the starting compound 1.
With the intention of detecting a possible reaction intermediate, we monitored the reaction using temperature-dependent IR spectra (Figure 3). The change in the IR spectral profile with the temperature is in good agreement with that observed in DSC. As noted earlier, the NH hydrogen of 1b in the crystalline state is hydrogen-bonded with the neighboring molecule at the carbonyl oxygen. Therefore, the absorption bands at 3250 and 1657 cm-1 are attributed to absorption of the hydrogen-bonded N-H and C=O, respectively. At the completion of the reaction above 280 °C, the N-H absorption band disappears and the C=O band shifts to a higher wavelength of 1725 cm-1.
Closer examination of Figure 3 indicates the appearance of new absorption bands at 3470 cm-1 in the NH region and at 1670 cm-1 in the carbonyl region, both being at wavenumbers higher than those at the outset. These bands occur transiently in a narrow temperature range at approximately 240 °C and are therefore attributed to molecules of 1 having no intermolecular hydrogen bonds. We can conclude that the relaxation of the crystalline lattice accompanying the cleavage of intermolecular hydrogen bonds occurs prior to hydrogen abstraction by oxygen, which is accelerated with increasing temperature and with the progress of the reaction.
Next, the thermal reactions of 2 and 3 were investigated with the aim of realizing the solid-state cleavage of the central C-C bond and the recombination of the resulting radicals. The dimeric compounds 2 and 3 were prepared by the oxidative dimerization of 1d and 1c, respectively, using iodine. The diastereomeric separation of the meso- and dl-forms of 2 was accomplished by column chromatography on silica gel using benzene as the eluent. The dl isomer was eluted fast, followed by the meso isomer. The isomeric structure of dl-2 was determined unambiguously by X-ray crystallographic analysis (Figure 4).10
The central C-C bond length of dl-2 (1.581(3) Å) is considerably larger than the lengths of common C(sp3)-C(sp3) bonds, indicating steric congestion around the central C-C bond. In accord with this observation, 1H NMR signals assignable to protons at the 2 and 6 positions of the 4-chlorophenyl ring showed remarkably broad signals at 6.3 ppm to show restricted rotation about the aryl-C bond at room temperature. The X-ray crystal structural analysis of the isolated 3 showed that it is the dl form.
Contrary to our expectation at the outset, dl-2, meso-2, and dl-3 did not undergo reactions in the solid state up to 200 °C. Upon further heating these compounds displayed a color change from pale yellow to orange in the solid state and at around 240 °C they melted to become dark brown.
The major products were readily identified as N-arylphthalimide from the NMR and mass spectra of the reaction mixture. Chromatography on silica gel indeed afforded N-arylphthalimide in 43% yield. There was no appreciable difference in the results between the dl and meso forms. The fate of the C2 carbon atom, which was incorporated in benzoic acid in the thermal reaction of 1, could not be identified despite considerable effort. Thus, we assume that the benzoyl radical formed according to Scheme 3 could not enter a chain reaction because of the absence of hydrogen in 2 and 3, therefore, more complex reactions are involved in the fate of the benzoyl radical intermediate.
We also carried out the photoreaction of 2 and 3 to determine whether the C-C bond cleavage could be induced by photoirradiation. The expected photoreactions did not take place in 2 or 3. On the other hand, their monomeric precursors 1a-1d exhibited a photoreaction. An acetonitrile solution of 1a was irradiated for 20 min in an atmospheric environment using a high-pressure mercury lamp through a Pyrex filter. The NMR and mass spectral analyses of the reaction mixture revealed the formation of N-arylphthalimide and benzoic acid in 87% and 81% yields, respectively. Similarly, the photoreaction of 1b-1d under aerobic conditions afforded the corresponding N-(p-substituted-phenyl)phthalimide and benzoic acid, each with approximately 80% yield, the products being identical to those obtained in the thermal reactions. When 1a was irradiated in nitrogen atmosphere for 40 min, no reaction was induced, indicating the intervention of atmospheric oxygen in the photochemical oxidative rearrangement. Thus, for the photoreaction, the same reaction mechanism as that considered for the thermal reaction could also be applicable (Scheme 3). Alternatively, taking into consideration the fact that 2-phenyl-1,3-indandione affords phthalic anhydride upon photolysis (Scheme 4),11 a mechanism including Norrish type 1 bond cleavage followed by ring closure would be possible if the tautomerization from the enamine to imino form via 1,3-hydrogen migration was photochemically induced (Scheme 4). However, this was not the case judging from the lack of photoreactivity of 1a in nitrogen atmosphere, as noted above.
References
1. For example: (a) E. Font-Sanchis, C. Aliaga, K. -S. Focsaneanu, and J. C. Scaiano, Chem. Commun., 2002, 1576; CrossRef (b) M. Frenette, C. Aliaga, E. Font-Sanchis, and J. C. Scaiano, Org. Lett., 2004, 6, 2579; CrossRef (c) M. Gomberg, J. Am. Chem. Soc., 1900, 22, 757; CrossRef This is the classical example of recombination of the carbon-radical pair, but was later disproved by the establishment of its correct quinonoid structure. See, (d) H. Lankamp, W. Th. Nauta, and C. MacLean, Tetrahedron Lett., 1968, 9, 249; CrossRef (e) J. M. McBride, Tetrahedron, 1974, 30, 2009; CrossRef (f) H. Voltz, W. Lotsch, and H.-W. Schnell, Tetrahedron, 1970, 26, 5343. CrossRef
2. (a) P. Kiri and G. Hyett, Adv. Mater. Lett., 2010, 1, 86; CrossRef (b) K. Amimoto and T. Kawato, J. Photochem. Photobiol., 2005, 6, 207; CrossRef (c) G. Kaupp, CrystEngComm, 2009, 11, 388; CrossRef (d) E. Hadjoudis and I. M. Mavridis, Chem. Soc. Rev., 2004, 33, 579.
3. R. W. Baldock, P. Hudson, A. R. Katritzky, and F. Soti, J. Chem. Soc., Perkin Trans. 1, 1974, 1422. CrossRef
4. (a) Y. Mori, N. Yamada, M. Kanazawa, Y. Horikoshi, Y. Watanabe, and K. Maeda, Bull. Chem. Soc. Jpn., 1996, 69, 2355; CrossRef (b) K. Maeda and T. Hayashi, Bull. Chem. Soc. Jpn., 1970, 43, 429; CrossRef (c) J. Ohkanda, Y. Mori, K. Maeda, and E. Osawa, J. Chem. Soc., Perkin Trans. 2, 1992, 59. CrossRef
5. (a) A. L. Buchachenko, M. V. Motyakin, and I. I. Aliev, in Reactivity of Molecular Solids, ed by. E. Boldyreva and V. Boldyrev, John Wiley & Sons, Chichester, 1999, pp. 222-239; (b) M. V. Motyakin, L. M. Pisarenko, P. Schuler, and H. B. Stegmann, Magn. Reson. Chem., 1995, 33, 471. CrossRef
6. K. U. Ingold, Acc. Chem. Res., 1969, 2, 1. CrossRef
7. L. M. Postnikov, N. G. Zarubin, E. V. Vichutinskaya, V. Y. Shlyapintokh, N. V. Martynov, and Y. E. Bryuske, Izv. Akad.. Nauk SSSR, Ser. Khim., 1968, 2, 260.
8. (a) J. D. Boyd, C. S. Foote, and D. K. Imagawa, J. Am. Chem. Soc., 1980, 102, 3641; CrossRef (b) H. O. House, R. J. Outcalt, J. L. Haack, and D. VanDerveer, J. Org. Chem., 1983, 48, 1654; CrossRef (c) A. Krebs, H. Schmalstieg, O. Jarchow, and K.-H. Klaska, Tetrahedron Lett., 1980, 21, 3171; CrossRef (d) Y.-Z. Chen, L.-Z. Wu, M.-L. Peng, D. Zhang, L.-P. Zhang, and C.-H. Tung, Tetrahedron, 2006, 62, 10688; CrossRef (e) A. P. Schaap, R. S. Handley, and B. P. Giri, Tetrahedron Lett., 1987, 28, 935; CrossRef (f) M. Balcl, Y. Taskesenliqil, and M. Harnandar, Tetrahedron Lett., 1989, 30, 3339. CrossRef
9. S. Kim, G. H. Joe, and J. Y. Do, J. Am. Chem. Soc., 1993, 115, 3328. CrossRef
10. The crystals of dl-2 and dl-3 were isolated as the 1:1 solvates with acetonitrile. The solvent molecules were readily released thermally or under reduced pressure. The thermal reaction was conducted using the solvent-free solids.
11. J. Rigaudy and P. Derible, Bull. Soc. Chim. Fr., 1965, 3047, 3055, and 3061.
12. This refers to the temperature at which the crystals start to melt with decomposition.