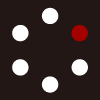
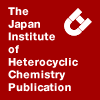
HETEROCYCLES
An International Journal for Reviews and Communications in Heterocyclic ChemistryWeb Edition ISSN: 1881-0942
Published online by The Japan Institute of Heterocyclic Chemistry
e-Journal
Full Text HTML
Received, 16th August, 2013, Accepted, 31st October, 2013, Published online, 12th November, 2013.
DOI: 10.3987/COM-13-S(S)112
■ Introducing the Diels-Alder Reactivity of 2-Furanmethanethiol with Selected Maleic Acid Derivatives
Mohanad Gh. Shkoor, Irena Nikoloska, and Adrian L. Schwan*
Department of Chemistry, University of Guelph, Guelph, Ontario, N1G 2W1, Canada
Abstract
Surprising chemoselectivity is demonstrated in the reaction of 2-furanmethanethiol with maleic anhydride and with N-phenylmaleimide. These maleic acid derivatives demonstrate a predilection for Diels-Alder cycloaddition, forgoing both conjugate addition and initial carbonyl attack. N-Ethylmaleimide showed a preference for conjugate addition, whereas diethyl fumarate or dimethyl maleate proved unreactive. The cycloadduct of 2-furanmethanethiol and maleic anhydride was subjected to dehydration/aromatization conditions to create benzo[c]thiophen-1(3H)-one-7-carboxylic acid. An alkylation attempt also led to the formation of a thiolester.The inhibition of the poly(ADP ribose polymerase) (PARP) family of enzymes has been tied to the treatment of cancer,1 vascular disease2 and the control of PARP overexpression, which can lead to necrotic cell death.3 Many inhibitors of the PARP enzyme possess the benzamide core (1) and particular efficacy is observed when the amide takes the form of a lactam or related structure.2-4 As such we have been pursuing synthetic approaches to the isoindolone ring system (2).5 In addition to the inhibitory activity related to PARP enzymes,4 the isoindolone core is present in compounds exhibiting substantial biological activity for a variety of therapeutic uses6 including antiangiogenic activity,7 inhibition of kinase enzymes,8 detection of schizophrenia9 and action as anti-inflammatory drugs.10
It was thought that a diverse and flexible library of inhibitors containing the compound 2 core could be accessed from compounds possessing the general structure 3, in which the carbonyl groups are undefined carboxylic acid derivatives, possibly in the form of an imide. Specifically, the plan is to attach the sulfur atom of 3 to the proximal carbonyl carbon, while also forming an additional 5-membered ring that incorporates the carbonyl carbons. Chemical adaptation thereafter, by way of sulfur oxidation and eventual fragmentation followed by Diels-Alder chemistry, would allow rapid construction of a library of compounds with diversity on the lower portion of the molecule.
Retrosynthetic analysis indicates that a logical approach to synthesizing 3 could be by Diels-Alder cycloaddition of furan 4a with maleic anhydride and aromatization of the cycloadduct. However, the direct Diels-Alder reaction of maleic anhydride and furan 4a was not expected to proceed, due to the well-established use of thiols and structurally related maleimides as linking agents by way of Michael addition chemistry.11 Hence, a protected form was employed, wherein p-methoxybenzyl (PMB) was chosen for its anticipated involvement in future transformations.12 As a starting point, sulfide 4b (PG = PMB) and maleic anhydride were reacted under a variety of conditions, but there were only traces of product that appeared to form, under selected situations, and the product was not pursued. Presuming that steric factors brought on by the presence of the protecting group were hindering the reaction, on a whim, the unprotected thiol (4a) was reacted with maleic anhydride. Surprisingly, a clean Diels-Alder reaction occurred in ether at room temperature and 5a was obtained as the lone product in 95% yield after recrystallization, and there was no evidence of conjugate addition. The exo nature of 5a was confirmed through analysis of the bridgehead to α-carbonyl 1H-1H coupling constant (J ~ 0 Hz).
Given the observed of maleic anhydride and 4a, the reaction of 4a with N-phenylmaleimide was investigated, in order to get more rapid access to target compound 3. Stirring furan 4a with N-phenyl- maleimide also brought about a cycloaddition and no conjugate addition was observed. Attempts to separate the two isomers were challenging as the reaction mixture was only partially tractable; presumably the reversibility of the system caused some problems. Modifications to the reaction conditions, including reactant ratios, solvent and temperature did not make the mixture more manageable. A pair of isomeric cycloadducts was eventually prepared in a total isolated yield of 39%. The difference in reactivity between furans 4a and 4b may be traced to the larger steric substituent on the 2-position of 4b; presumably the tenuous thermodynamic preference for furan-based cycloadduct formation vs. reversion to starting materials13 is negatively affected by the presence of the large group at the 2-position.
The Diels-Alder chemistry of furfuryl alcohol14 and furfuryl amine15 with maleic anhydride has already been established. Furfuryl alcohol can undergo cycloaddition directly with the furan diene without intervention of the alcohol.14 In contrast, furfuryl amines appear to initially open maleic anhydride through carbonyl attack by the nitrogen, thereby facilitating an intramolecular cycloaddition.15b On the other hand, the literature indicates 2-furanmethanethiol and it S-alkylated derivatives have never been employed in simple bimolecular Diels-Alder reactions. The only related Diels-Alder involvement of the 2-furfurylthio backbone has been the IMDA of sulfides derived from 2-furanmethanethiol.16 Moreover, given the vast literature on the use of maleimides for labelling thiols via a conjugate addition reaction11a,b under neutral (aqueous) conditions,17 the tendency of 2-furanmethanethiol to preferentially participate in the Diels-Alder reaction is remarkable.
The isolation of exclusively the exo isomer of 5a is uncommon given that furan cycloadditions usually give a mixture of endo and exo isomers. To investigate this, a low temperature 1H NMR study was performed. Before beginning, it was determined that the cycloadditions have the same single product in CHCl3, permitting an NMR analysis in CDCl3. A reaction vessel containing CDCl3 held at –78 °C was charged with equimolar amounts of maleic anhydride and furan 4a, in concentrations comparable to the reaction conditions. About 0.5 mL of the solution was transferred to an NMR tube and the tube was inserted in the NMR probe which was previously precooled to -10 °C.
In the early stages of the analysis, the NMR acquisitions were of poor resolution because the maleic anhydride was not completely soluble (Figure 2, a & b). As time progressed, the resolution improved and the peaks could be interpreted and assigned. As such, the singlet due to the bridgehead H of exo-5a was recognizable, but was accompanied by a doublet of doublets (J = 1.5, 5.6 Hz) at slightly lower ppm (Figure 2 c), which was assigned to be the bridgehead H of the endo isomer of 5a, based on the larger coupling constant. The relative ratio of the exo:endo isomers was roughly estimated to be 60:40 (Figure 2, a). As time progressed and the temperature rose to rt, the intensity of the minor isomer decreases, indicating the slow conversion to the thermodynamically preferred exo product (Figure 2 d-f). After several more hours at rt, there was exclusive formation of the exo adduct. Clearly, the two isomers form at similar rates, but with warming cycloreversion and formation of the thermodynamically more stable exo isomer occurs.18
The reaction of N-ethylmaleimide with the unprotected thiol (4a) gave both Michael addition and a single Diels-Alder adduct (endo-6b) with the preference of the conjugate addition product 7b. The reaction was then done at cold temperature (-5 to 0 ºC) in attempt to change the ratio of products but no significant change was observed. The literature suggests that N-alkyl and N-phenyl substituents impart similar contributions to the kinetics of maleimide cycloadditions to furans.19 As such, the observed reactivity differences of N-ethyl and N-phenylmaleimides may be attributable to the higher activity of the nitrogen lone electron pair of the N-ethyl group, which may better facilitate some of the proton transfers20 required for the conjugate addition. Finally, to probe other dienophiles, the reaction chemistry of diethyl fumarate and dimethyl maleate with 4a was evaluated but neither Diels-Alder chemistry nor conjugate addition reactions were evident in diethyl ether at room temperature or in refluxing toluene.
Cycloadduct 5a was subjected to conditions to effect aromatization of the [2.2.1]-bicyclic ring.21 Employing 5a, low mass recoveries were observed when established harsh H2SO4 conditions were employed,22 but using the milder two-phase combination of H2SO4 and CH2Cl223 brought about a single, nearly pure product with moderate mass recovery. Spectral analysis of the purified product revealed aromatization, which was also accompanied by thiol attack of the proximal anhydride carbonyl; a heretofore unknown thiophthalide (benzo[c]thiophen-1(3H)-one-7-carboxylic acid, 8) was isolated in 38% purified yield. Compound 8 is the first thiophthalide possessing a carboxylic acid at the 7-position on the aromatic ring. Thiophthalides are garnering increased attention for their value as useful intermediates in organic synthesis.24 As an additional adaptation, were we able to convert acid 8 to its N-benzylamide 9 (45%, two steps, unoptimized), while keeping the thiolester linkage intact (Scheme 2).
Thiophthalides 8 and 9 represent successfully synthesized versions of 3, wherein the R and X’ groups are identified by, and indeed eliminated through, the formation of the thiolester linkage. Nevertheless we sought to see if the thiol functionality could be alkylated to create a different form of 3. As such, 5a was reacted with p-methoxybenzyl chloride (PMBCl) in the presence of DBU, but although the PMB unit was incorporated, 5b was not formed. Instead the product obtained had lost the anhydride functionality (IR, 13C NMR) but the oxabicyclic framework remained in place (1H NMR). The product exhibited two new carbonyl stretches at 1735 and 1698 cm-1 and the new benzyl protons appear at 5.12 ppm, indicative of attachment to an ester oxygen. A 13C NMR resonance was also evident near 204 ppm. The new product was assigned the structure 10, which is consistent with the characterization data. Although base was used for this transformation, the original stereochemistry of the ring substituents appears intact, based on 1H-1H coupling constants of the hydrogens adjacent to the carbonyl groups.14b,25 Thiolactone formation is not surprising in light of the chemistry of the corresponding furfuryl alcohol chemistry. Compound 10 is presumably formed through intermediate 11 which undergoes O-alkylation with in situ PMB-Cl.14 Aromatization attempts on 10 using the milder conditions also led to the isolation of thiophthalide 8 in 47% yield.
To summarize, a Diels-Alder approach has permitted the construction of a 3-thiomethylated 1,2-dicarboxyl benzene derivative. Specifically, thiophthalide 8, a novel benzo[c]thiophen-1(3H)-one, was created in two steps from 2-furanmethanethiol and maleic anhydride. 2-Furanmethanethiol demonstrates surprising chemoselectivity with maleic anhydride and with N-phenylmaleimide, favoring cycloaddition over conjugate addition and carbonyl attack. The current study suggests that 2-furanmethanethiol or its derivatives could be developed into new bifunctional cross-linking reagents possessing thiol vs diene selectivity for various fluorescent maleic acid derivatives.26 Exploration of the chemistry of thiophthalides 8 and 9 is underway for eventual creation of new isoindolones.
EXPERIMENTAL
Preparation of furfuryl p-methoxybenzyl sulfane (4b). 2-Furylmethanethiol (1 eq, 5.68 g, 49.7 mmol) and Et3N (1 eq, 7.0 mL, 49.7 mmol) together with 25 mL of DCM were set to stir at room temperature, under a constant stream of nitrogen gas. p-Methoxybenzyl bromide (1.1 eq, 11 g, 5.50 mmol) was dissolved in 5 mL of DCM and added to the above mixture dropwise. The reaction was stirred at room temperature for 2 h during which time a precipitate formed. The precipitate was redissolved in DCM and 30 mL of 0.1 M HCl was added. The aqueous layer was extracted with DCM and washed with brine, and the combined organics were dried over MgSO4, filtered and concentrated under reduced pressure. The crude material was further concentrated under high vacuum and purified by silica gel flash chromatography (9:1, hexanes/EtOAc) to yield 4b as a white solid (60%). Rf = 0.24 (9:1, hexanes/EtOAc); 1H NMR (400 MHz, CDCl3) δ 7.37 (1H, dd, J = 1.9 & 0.9 Hz, 1H), 7.23 (m, 2H), 6.85 (m, 2H), 6.32 (dd, J = 3.1 & 0.9 Hz, 1H), 6.15 (dd, J = 1.9 & 3.1 Hz, 1H), 3.80 (s, 3H), 3.66 (s, 2H), 3.58 (s, 2H); 13C NMR (100 MHz, CDCl3) δ 158.7, 151.8, 142.1, 130.1, 129.8, 113.9, 110.4, 107.5, 55.3, 35.2, 27.3; IR (neat): υ 2954, 2912, 2834, 1609, 1175 cm-1. Anal. Calcd for C13H14O2S: C, 66.64 H, 6.02. Found: C, 66.71 H, 6.00.
General procedure for the Diels-Alder cycloaddition of 2-furanmethanethiol (4a) with various dienophiles. The dienophile 1.2 eq was dissolved in 2 mL dry Et2O and 2-furylmethanethiol (1.00 g, 1 eq, 8.8 mmol) were added and the solution stirred overnight at room temperature. The crude was dried and purified by flash column chromatography (hexanes/EtOAc) unless otherwise mentioned.
Reaction of thiol 4a with maleic anhydride. The reaction of maleic anhydride (1.2 eq, 1.03 g, 10.5 mmol) and 4a (1.00 g, 1 eq, 8.8 mmol) provided crude 2.0 g of 5a which was recrystallized from DCM to provide pure 5a (1.76 g, 95%) as white plates. mp 69-71 ºC. 1H NMR (400 MHz, acetone-d6) δ 6.70-6.67 (m, 2H), 5.36 (1H, d, J = 1.5 Hz), 3.62 (1H, d, J = 6.7 Hz), 3.53 (1H, d, J = 6.7 Hz), 3.16 (ABX pattern, JAB = 14.4 Hz, JAB = 9.1 Hz, JAB = 8.4 Hz, 2.22 (dd, J = 9.1 & 8.4 Hz, 1H); 13C NMR (150 MHz Acetone-d6) δ 170.8, 169.8, 138.0, 137.62, 93.1, 82.4, 52.7, 50.5, 23.1; IR (neat): υ 3090, 3006, 2582, 1841, 1775, 1227 cm-1. Anal. Calcd for C9H8O4S: C, 50.94 H, 3.80. Found: C, 50.92 H, 3.90.
Variable temperature 1H NMR study of the reaction of thiol 4a with maleic anhydride. Quantities of maleic anhydride and 4a as employed above were placed in a vessel with CDCl3 (2 mL), precooled to -78 ºC. Approximately 0.5 mL of this solution was transferred to a precooled NMR tube which was then quickly placed in the probe (precooled to -10 ºC) of a Bruker 600 MHz NMR. 1H NMR spectra were acquired hourly for 23 h. The temperature was held at -10 ºC for 17 h and then allowed to warm to +10 ºC and then to rt. Selected data are shown in Figure 2. A final 1H NMR spectrum was obtained after 16 h at rt and showed only the exo isomer of 5a.
Reaction of thiol 4a with N-phenylmaleimide. The reaction of N-phenylmaleimide (1.2 eq, 1.82 g, 10.5 mmol) and 4a (1.00 g, 1eq, 8.8 mmol) provided a mixture which was subjected to flash chromatography to provide exo-6a (0.78 g, 31%) as a white solid and endo-6a (0.21g, 8%) as a pale yellow solid.
Data for exo-6a: mp 130-132 ºC. 1H NMR (400 MHz, CDCl3) δ 7.51-7.42 (m, 3H), 7.30–7.27 (m, 2H), 6.67 (d, J = 5.7 Hz, 1H), 6.62 (d, J = 5.7 Hz, 1H), 5.39 (s, 1H), 3.26-3.17 (m, 3H), 3.08 (dd, J = 14.4 & 9.5 Hz, 1H), 2.20 (dd, J = 9.5 & 8.5 Hz, 1H); 13C NMR (100 MHz, CDCl3) δ 175.0, 174.1, 138.6, 137.2, 131.5, 129.2, 128.9, 126.5, 92.6, 81.7, 50.9, 48.4, 24.0; IR (neat): υ 2567, 1776, 1709, 1499, 1386, 1195 cm-1; HRMS (TOF, ESI) Calcd for [C15H13O3S + H]+ 288.6089. Found: 288.0684.
Data for endo-6a: mp 72-74 ºC. 1H NMR (400 MHz, CDCl3) δ 7.49-7.43 (m, 3H), 7.28–7.12 (m, 2H), 6.62 (dd, J = 1.7, 5.7 Hz, 1H), 6.50 (d, J = 5.7 Hz, 1H), 5.41 (dd, J = 1.6, 5.5 Hz, 1H), 3.87 (dd, J = 7.7 & 5.5 Hz, 1H), 3.66 (d, J = 7.7 Hz, 1H), 3.46 (dd, J = 14.6 & 8.0 Hz, 1H), 3.27 (dd, J = 14.6 & 8.7 Hz, 1H) 1.80 (dd, J = 8.7 & 8.0 Hz, 1H); 13C NMR (100 MHz, CDCl3) δ 174.0, 173.8, 136.1, 135.6, 131.3, 129.3, 128.9, 126.3, 92.2, 79.5, 48.9, 47.9, 26.3; IR (neat): υ 2569, 1776, 1710, 1498, 1383, 1186 cm-1; HRMS (TOF, ESI) Calcd for [C15H13O3S + H]+ 288.0689. Found: 288.0692.
Reaction of thiol 4a with N-ethylmaleimide. The reaction of N-ethylmaleimide (1.2 eq, 1.31 g, 10.5 mmol) and 4a (1.00 g, 1eq, 8.8 mmol) provided a mixture which was subjected to flash chromatography to provide 7b (0.29 g, 14%) as a brown oil and endo-6b (0.20 g, 10%) as a white solid.
Data for 7b: 1H NMR (400 MHz, CDCl3) δ: 7.39 (dd, J = 1.8 & 0.7 Hz, 1H), 6.33 (dd, J = 3.2 & 1.8 Hz, 1H), 6.29 (dd, J = 3.2 & 0.7 Hz, 1H), 4.31 (d, J = 14.9 Hz, 1H), 3.84 (d, J = 14.9 Hz, 1H), 3.66 (dd, J = 9.2 & 4.0 Hz, 1H), 3.56 (q, J = 7.2 Hz, 2H), 3.03 (dd, J = 18.8 & 9.2 Hz, 1H), 2.40 (dd, J = 18.8 & 4.0 Hz, 1H), 1.18 (t, J = 7.2 Hz, 3H); 13C NMR (100 MHz, CDCl3) δ: 174.8, 174.6, 135.8, 135.3, 91.7, 79.1, 48.9, 47.9, 33.5, 26.3, 12.7; IR (neat): υ 3119, 2980, 2879, 1774, 1720, 1591, 1503, 1398, 1351, 1227 cm-1; Anal. Calcd for C11H13NO3S: C, 55.21 H, 5.48. Found: C, 54.99 H, 5.37.
Data for endo-6b: mp 93-95 ºC (EtOAc/hexanes). 1H NMR (400 MHz, CDCl3) δ: 6.36 (dd, J = 5.8 & 1.4 Hz, 1H), 6.25 (d, J = 5.8 Hz, 1H)5.21 (dd, J = 5.4 & 1.4 Hz, 1H), 3.59 (dd, J = 7.6 & 5.4 Hz, 1H), 3.37 (d, J = 8.7 Hz, 1H), 3.34-3.29 (m, 3H), 3.13 (dd, J = 14.4 & 8.7 Hz, 1H), 1.68 (t. J = 8.3 Hz, 1H), 0.97 (t, J = 7.2 Hz, 3H); 13C NMR (100 MHz, CDCl3) δ: 176.5, 174.5, 149.9, 142.7, 110.6, 108.7, 37.8, 35.4, 34.0, 28.1, 12.9; IR (neat): υ 3084, 2979, 2937, 2570, 1768, 1698, 1444, 1399, 1341, 1283, 1225, 1135 cm-1. Anal. Calcd for C11H13NO3S: C, 55.21 H, 5.48. Found: C, 55.40 H, 5.48.
Dehydration/aromatization of cycloadduct 5a. Compound 5a (1.00 g, 3.00 mmol) was added portion wise to a cold (-30 ºC) mixture of H2SO4/DCM (1:2 v/v, 10 mL), the solution was allowed to warm to room temperature over 4 h and then was poured in crushed ice. The mixture was extracted with DCM (3 X 10 mL), washed with water and dried over MgSO4. Concentration in vacuo gave thiophthalide 8 (0.22 g, 38%) as a white solid. mp 138-140 °C (CHCl3). 1H NMR (400 MHz, CDCl3) δ: 8.57 (dd, J = 7.3, 1.4 Hz, 1H), 7.86-7.81 (m, 2H), 4.65 (s, 2H); 13C NMR (100 MHz, CDCl3) δ: 205.7, 164.4, 149.7, 133.2, 129.7, 128.0, 126.4, 123.9, 35.3; IR (neat): υ 3200-2500, 2962, 2934, 1721, 1577, 1465, 1439, 1291, cm-1. GS/MS (CI): 194 (M+, 87), 177((M-OH)+, 100); Anal. Calcd for C9H6O3S: C, 55.66 H, 3.11. Found: C, 55.80 H, 3.31.
Conversion of thiophthalide 8 to N-benzylamide 9. Compound 8 (500 mg, 2.6 mmol) was dissolved in dry DCM (10 mL) in a flame dried flask purged with argon. Oxalyl chloride (0.26 ml, 3.1 mmol) and a catalytic amount of DMF were added to the mixture. After stirring at room temperature for 3 h, the solvent and unreacted oxalyl chloride were evaporated under high vacuum. The yellow residue was dissolved in dry DCM (10 mL) and the solution cooled in an ice bath. K2CO3 (400 mg, 2.8 mmol) and a solution of benzylamine (0.31 mL, 2.8 mmol) in DCM (3 mL) were then added. After stirring for 8 h the reaction mixture was extracted with water (20 mL), 5% HCl (20 mL), sat. aq. NaHCO3 (20 mL) and water (20 mL). After drying over MgSO4, the solvent was evaporated the solid remained was rinsed with EtOAc to give 330 mg (45%) of white solid in pure form. A recrystallized sample (DCM) gave white needles, mp 166-168 ºC. 1H NMR (400 MHz, CDCl3) δ 8.27 (br s, 1H), 8.09 (d, J = 7.5 Hz, 1H), 7.71 (t, J = 7.6 Hz, 1H), 7.63 (d, J = 7.6 Hz, 1H), 7.44 (d, J = 7.4 Hz, 2H), 7.38 (t, J = 7.4 Hz, 2H), 7.30 (m, 1H), 4.70 (d, J = 5.5 Hz, 2H), 4.51 (s, 2H). 13C NMR (100 MHz, CDCl3) δ 199.3, 165.7, 148.8, 138.0, 134.0, 133.1, 132.0, 130.5, 128.7, 138.6, 128.1, 127.5, 44.5, 34.2; IR (neat): υ 3271, 1688, 1643, 1546, 1453, 1221, 1170, 996. HRMS (TOF, ESI) Calcd for [C16H13NO2S]+ 284.0740. Found: 284.0749.
Alkylation of cycloadduct 5a. Thiol 5a (0.21 g, 1 eq, 1.0 mmol) was dissolved in dry DCM (3 mL) under Ar. To this solution was added DBU (0.17 g, 1.1 eq, 1.1 mmol) followed by p-methoxybenzyl chloride (0.17 g, 1.1 eq, 1.1 mmol) and the reaction was stirred overnight at room temperature. Water was added and the reaction was extracted with DCM (3 X 10 mL) and the combined organics were dried over MgSO4, filtered and concentrated. The crude product was purified by flash column chromatography (hexanes/EtOAc) to provide 10 (0.15 g, 46%) as a pale yellow solid. mp 110-113 °C (CHCl3). 1H NMR (400 MHz, CDCl3 δ 7.36 (m, 2H), 6.90 (m, 2H), 6.55 (m, 1H), 6.47 (1H, d, J = 5.7 Hz, 1H), 5.16 (s, 1H), 5.12 (AB pattern, J = 12.0 Hz, 2H), 4.03 (d, J = 12.7 Hz, 1H), 3.83 (d, J = 12.7 Hz, 1H), 3.82 (s, 3H), 2.97 (d, J = 8.8 Hz, 1H), 2.83 (d, J = 8.8 Hz, 1H); 13C NMR (150 MHz CDCl3) δ 203.7, 171.4, 159.7, 138.3, 136.5, 130.5, 127.7, 113.9, 92.7, 81.4, 67.1, 58.1, 55.3, 48.8, 33.0; IR (neat) υ 3003, 2954, 2837, 1735, 1697, 1613, 1515, 1250, 1166 cm-1; HRMS (TOF, ESI) Calcd for [C17H16O5S + NH4]+ 350.1057. Found: 350.1046.
Dehydration/aromatization of compound 10. The chemistry was carried out as above using 200 mg (0.60 mmol) to give 55.4 mg (47%) of 8.
ACKNOWLEDGEMENTS
The authors thank the Natural Sciences and Engineering Research Council of Canada for funding. M.Gh.S. thanks the Ontario government for an MRI postdoctoral fellowship.
References
1. a) T. V. T. Le, J. H. Suh, N. Kim, and H.-J. Park, Bioorg. Med. Chem. Lett., 2013, 23, 2642; CrossRef b) C. Cosi, Expert Opin. Ther. Pat., 2002, 12, 1047. CrossRef
2. M. H. Javaid, S. Gomez, X.-L. F. Cockcroft, K. A. Menear, and N. M. B. Martin, WO 144652A2 2007.
3. a) K. S. Putt and P. J. Hergenrother, Anal. Biochem., 2004, 326, 78; CrossRef b) D. R. Martin, A. J. P. Lewington, M. R. Hammerman, and B. J. Padanilam, Am. J. Physiol., 2000, 279, R1834.
4. P. G. Jagtap, G. J. Southan, E. Baloglu, S. Ram, J. G. Mabley, A. Marton, A. Salzman, and C. Szabo, Bioorg. Med. Chem. Lett., 2004, 14, 81. CrossRef
5. a) A. L. Schwan, P. A. Duspara, M. M. Paquette, and A. R. Merrill, Synlett, 2006, 3115; CrossRef b) G. Stajer and F. Csende, Curr. Org. Chem., 2005, 9, 1277. CrossRef
6. a) V. More, R. Rohlmann, O. G. Mancheno, C. Petronzi, L. Palombi, R. A. De, M. A. Di, and A. Massa, RSC Adv., 2012, 2, 3592; b) J. Li and J. Zhang, IDrugs, 2001, 4, 804.
7. W.-W. Lue, Y.-J. Gao, M.-Z. Su, Z. Luo, W. Zhang, G.-B. Shi, and Q.-C. Zhao, Helv. Chim. Acta, 2013, 96, 109. CrossRef
8. R. Boulahjar, A. Ouach, C. Matteo, S. Bourg, M. Ravache, R. l. Guevel, S. Marionneau, T. Oullier, O. Lozach, L. Meijer, C. Guguen-Guillouzo, S. Lazar, M. Akssira, Y. Troin, G. Guillaumet, and S. Routier, J. Med. Chem., 2012, 55, 9589. CrossRef
9. M. Ball, A. Boyd, G. Churchill, M. Cuthbert, M. Drew, M. Fielding, G. Ford, L. Frodsham, M. Golden, K. Leslie, S. Lyons, B. McKeever-Abbas, A. Stark, P. Tomlin, S. Gottschling, A. Hajar, J.-l. Jiang, J. Lo, and B. Suchozak, Org. Process Res. Dev., 2012, 16, 741. CrossRef
10. F. Csende, F. Miklos, and A. Porkolab, ARKIVOC, 2013, ii, 378. CrossRef
11. a) J. L. Meier and M. D. Burkart, Methods Enzymol., 2009, 458, 219; CrossRef b) M. H. Stenzel, ACS Macro Lett., 2013, 2, 14; CrossRef c) S.-Y. Tang, J. Shi, and Q.-X. Guo, Org. Biomol. Chem., 2012, 10, 2673. CrossRef
12. A. L. Schwan, R. R. Strickler, Y. Lear, M. L. Kalin, T. E. Rietveld, T.-J. Xiang, and D. Brillon, J. Org. Chem., 1998, 63, 7825. CrossRef
13. L. Rulíšek, P. Šebek, Z. Havlas, R. Hrabal, P. Čapek, and A. Svatoš, J. Org. Chem., 2005, 70, 6295. CrossRef
14. a) A. Pelter and B. Singaram, Tetrahedron Lett., 1982, 23, 245; CrossRef b) A. Pelter and B. Singaram, J. Chem. Soc., Perkin Trans. 1, 1983, 1383. CrossRef
15. a) J. C. Kondoli, D. Prajapati, J. S. Sandhu, and B. J. Wakefield, J. Chem. Res., (S), 1987, 76; b) V. P. Zaytsev, N. M. Mikhailova, I. K. Airiyan, E. V. Galkina, V. D. Golubev, E. V. Nikitina, F. I. Zubkov, and A. V. Varlamov, Chem. Heterocycl. Compd., 2012, 48, 505. CrossRef
16. a) M. Dadwal, M. K. Kesharwani, V. Danayak, B. Ganguly, S. M. Mobin, R. Muruganantham, and I. N. N. Namboothiri, Eur. J. Org. Chem., 2008, 6106; CrossRef b) M. Karaarslan and A. Demircan, Asian J. Chem., 2007, 19, 2999; c) L. L. Klein, J. Org. Chem., 1985, 50, 1770; CrossRef d) M. Lautens and E. Fillion, J. Org. Chem., 1997, 62, 4418; CrossRef e) I. N. N. Namboothiri, M. Ganesh, S. M. Mobin, and M. Cojocaru, J. Org. Chem., 2005, 70, 2235; CrossRef f) F. Ponten and G. Magnusson, J. Org. Chem., 1997, 62, 7978. CrossRef
17. G. Viault, S. Dautrey, N. Maindron, J. Hardouin, P.-Y. Renard, and A. Romieu, Org. Biomol. Chem., 2013, 11, 2693. CrossRef
18. The NMR study also provided an opportunity to probe possible intermolecular H-bonding of the thiol hydrogen to maleic anhydride prior to cycloaddition. However, no evidence in support of this premise was observed.
19. R. C. Boutelle and B. H. Northrop, J. Org. Chem., 2011, 76, 7994. CrossRef
20. I. V. Koval’, Russ. J. Org. Chem., 2007, 43, 319. CrossRef
21. Aromatization/dehydration of isomers 6a led to intractable mixtures.
22. M. R. Dhananjeyan, Y. P. Milev, M. A. Kron, and M. G. Nair, J. Med. Chem., 2005, 48, 2822. CrossRef
23. Y. Kuninobu, Y. Nishina, and K. Takai, Tetrahedron, 2007, 63, 8463. CrossRef
24. a) P. Mitra, B. Shome, D. S. Ranjan, A. Sarkar, and D. Mal, Org. Biomol. Chem., 2012, 10, 2742; CrossRef b) D. Mal and S. R. De, Org. Lett., 2009, 11, 4398. CrossRef
25. A. Basso, L. Banfi, R. Riva, and G. Guanti, J. Org. Chem., 2004, 70, 575. CrossRef
26. a) H. J. Gruber, G. Kada, B. Pragl, C. Riener, C. D. Hahn, G. S. Harms, W. Ahrer, T. G. Dax, K. Hohenthanner, and H.-G. Knaus, Bioconj. Chem., 2000, 11, 161; CrossRef b) B. Frisch, C. Boeckler, and F. Schuber, Bioconj. Chem., 1996, 7, 180; CrossRef c) C. Boeckler, B. Frisch, S. Muller, and F. Schuber, J. Immunol. Methods, 1996, 191, 1. CrossRef