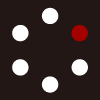
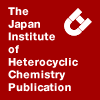
HETEROCYCLES
An International Journal for Reviews and Communications in Heterocyclic ChemistryWeb Edition ISSN: 1881-0942
Published online by The Japan Institute of Heterocyclic Chemistry
e-Journal
Full Text HTML
Received, 18th September, 2013, Accepted, 30th October, 2013, Published online, 7th November, 2013.
DOI: 10.3987/COM-13-S(S)117
■ Synthesis and Properties of Conducting Polymer Nanotubes with Redox-Active Tetrathiafulvalene
Shinya Nambu, Tsukasa Nakahodo, and Hisashi Fujihara*
Department of Applied Chemistry, Faculty of Science and Engineering, Kinki University, 3-4-1, Kowakae, Higashi-Osaka 577-8502, Japan
Abstract
Electropolymerization of a new chiral (S)-binaphthyltetrathiafulvalenyl-terthiophene, 1-TTF, produced the corresponding polymer films on an electrode surface. The polymer nanotubes consisting of the tetrathiafulvalene and polythiophene were synthesized from the template-based electropolymerization of 1-TTF in a nanoporous alumina template.The study of nanostructure materials such as carbon and polymer nanotubes (NTs) and metal nanoparticles (NPs) has been an extremely active area in recent years because of their unique electronic, optical, and catalytic properties.1-3 Specifically, conducting polymer one-dimensional nanomaterials have become a rapidly growing field of research, because they display new properties related to their nanoscale size and have significantly improved the performance of devices.3 Conducting polymers, such as polythiophenes, are indispensable materials for the development of displays, electronic and energy storage devices, actuators, sensors, and neural interfaces. The nanotubular structure of polythiophene (PT) is one of the ideal structures that can enhance the device performance by improving the charge-transport rate as well as increasing the surface area. However, the functionalization of the conducting polymer NTs with organic molecules or metal NPs has received less attention. Recently, we reported the synthesis of hybrid NTs consisting of metal NPs (or magnetite NPs) and polythiophene, and the encapsulation and release of molecules in functional polymer nanotubes.4
The incorporation of electroactive components, tetrathiafulvalene (TTF), into nanostructured materials and polymers is currently a challenge in the fields of nanochemistry and material science.5 TTF is a π-conjugated system that has been exploited thoroughly as a conductive material. TTF can be easily oxidized in two successive one-electron steps into a stable radical cation (TTF .+) and a dication (TTF2+).
Although a number of studies for synthesis of polymers containing TTF have been reported, there is no clear-cut example of the preparation of nanotube consisting of TTF and polythiophene as constituents of nanotubes. The development of a method for the synthesis of chiral conducting polymer nanotubes remains an important and challenging issue. We have now performed the synthesis of a new chiral TTF (1-TTF) containing terthiophene as an electropolymerization site and (S)-binaphthyl, the surface modification of an electrode with the 1-TTF-polymer films by the electropolymerization of 1-TTF, and the preparation of the polymer nanotubes of TTF from the template-based electropolymerization of 1-TTF in a nanoporous alumina membrane as a template. In contrast to 1-TTF, it has been reported that the electropolymerization of 3-thienyl substituted TTF showed no polymer film growing on electrodes.6 This communication describes the synthesis and properties of the TTF-functionalized polythiophene NTs.
The electrochemical behavior of 2-TTF without terthienyl group was investigated by cyclic voltammetry. Figure 1a shows cyclic voltammogram (CV) of 2-TTF in 0.1 M Bu4NPF6-CH2Cl2 at a glassy carbon
electrode. The TTF unit of 2-TTF undergoes two successive reversible one-electron redox reactions, giving two pairs of well-defined redox peaks. The first and second peaks appear at E11/2 = +0.30 V and E21/2 = +0.70 V vs. Ag/0.1 M AgNO3, respectively. The electrochemical stability of 2-TTF on a glassy carbon electrode in CH2Cl2 was investigated by continuously cycling the potential. After 10 scans between +0.15 and +1.25 V, the CVs were not changed (Figure 1b).
In order to fabricate the polymer NTs by electropolymerization, the electropolymerization of the 1-TTF was studied in the absence or in the presence of a porous alumina membrane as a template. Electrochemical polymerization is an elegant strategy for the immobilization of functional materials on a solid surface. The surface modification of electrodes with polymer films has been performed by the electropolymerization of thiophene-based monomers. Accordingly, the electrochemical properties of the 1-TTF were studied by
cyclic voltammetry. The cyclic voltammogram of the 1-TTF in 0.1 M Bu4NPF6-CH2Cl2 at a glassy carbon electrode showed one irreversible oxidation peak at Ep = +0.98 V (vs. Ag/0.1 M AgNO3) due to the oxidation of the terthienyl group, and two redox peaks owing to the TTF group at E11/2 = +0.30 V and E21/2 = +0.77 V vs. Ag/Ag+ (Figure 2). In the following scans, an increase in the anodic/cathodic peaks of the 1-TTF is noted as an indication of the polymer growth. Subsequently, we succeeded in the synthesis of new polymer nanotubes of TTF and polythiophene when a nanoporous alumina membrane as the template was used for the electropolymerization of the 1-TTF.
The template-based electropolymerization of 1-TTF in a nanoporous alumina membrane (AM) as a template produced the corresponding polythiophene NT-modified alumina membranes, which were converted into the polythiophene NTs (1-TTF-PT-NTs) upon dissolution of the alumina membranes by a sodium hydroxide solution (Figure 3). The UV-vis spectrum of 1-TTF-PT-NTs exhibits the absorption due to the polythiophene at ca. 450 nm (Figure 4a). The polymer nanotubes (1-TTF-PT-NTs) were characterized by scanning electron microscopy (SEM) and transmission electron microscopy (TEM)b)c). The SEM images of 1-TTF-PT-NTs show a good morphology in Figures 5 and 6. Figure 5a shows an SEM image of 1-TTF-PT-NTs, which were grown using the alumina template; most of them have outer diameters of about 200-250 nm. The TEM image of 1-TTF-PT-NTs reveals that the outer diameter of the nanotubes is about 200 nm, with wall thickness of around 50 nm (Figure 6). The elemental composition of 1-TTF-PT-NTs was confirmed by the energy-dispersive X-ray (EDX) analysis of single nanotubes; its EDX spectrum (Figure 5b) shows the presence of O, S and C elements in the nanotubes, which confirms that the nanotubes (1-TTF-PT-NTs) have been successfully prepared from the template-based electropolymerization of the (S)-chiral binaphthyl-terthiophene, 1-TTF, in a nanoporous alumina membrane. The circular dichroism (CD) spectrum of 1-TTF-PT-NTs shows negative Cotton effects (Figure 4b). Studies on the chiral properties of 1-TTF-PT-NTs are in progress.
In summary, the 1-TTF with a polymerizable group produces the polymer films on an electrode. New polymer nanotubes of TTF and polythiophene as constituents of nanotubes are obtained by the template-based electropolymerization of terthienyl-tetrathiafulvalene in a nanoporous alumina template. A study on the encapsulation of electron-acceptor molecules in the inner cavity of the tetrathiafulvalenyl-polythiophene nanotubes is now under way.
EXPERIMENTAL
A typical procedure for the synthesis of polymer nanotubes (1-TTF-PT-NTs) is as follows (Figure 3). The electropolymerization was performed by attaching the alumina membrane (AM) (Whatman Anodisc; pore diameter of about 200-250 nm, thickness of 60 µm) to a Pt electrode, another Pt electrode was used as the counter electrode, and Ag/0.1 M AgNO3 was used as the reference electrode. The electrolysis solution contained 1-TTF (5.0 mM) in 0.1 M Bu4NPF6-CH2Cl2. A voltage of +1.0 V (vs. Ag/0.1 M AgNO3) was applied for 40 min. A 1-TTF-PT-NT-deposited nanopore of AM (1-TTF-PT-NT-AM) was formed. After the electropolymerization, removal of the alumina membrane of 1-TTF-PT-NT-AM with 1 M NaOH led to the release of the 1-TTF-PT-NTs. Scanning and transmission electron microscopies (SEM and TEM), and energy-dispersive X-ray (EDX) spectroscopy were used for the analysis of the synthesized NTs.
ACKNOWLEDGEMENTS
This work was supported by a Grant-in-Aid for Scientific Research on Innovative Areas “New Polymeric Materials Based on Element-Blocks (No. 2401)” (25102544) and a Scientific Research, No. 25410215, from the Ministry of Education, Culture, Sports, Science, and Technology, Japan.
References
1. C. N. R. Rao and A. Govindaraj, Nanotubes and Nanowires, RSC Publishing, 2005; G. A. Ozin, A. C. Arsenault, and L. Cademartiri, Nanochemistry, RSC Publishing, 2009.
2. N. Karousis and N. Tagmatarchis, Chem. Rev., 2010, 110, 5366. CrossRef
3. D. T. Mitchell, S. B. Lee, L. Trofin, N. Li, T. K. Nevanen, H. Soderlund, and C. R. Martin, J. Am. Chem. Soc., 2002, 124, 11864; CrossRef S. I. Cho, W. J. Kwon, S.-J. Choi, P. Kim, S.-A. Park, J. Kim, S. J. Son, R. Xiao, S.-H. Kim, and S. B. Lee, Adv. Mater., 2005, 17, 171; CrossRef S. J. Son and S. B. Lee, J. Am. Chem. Soc., 2006, 128, 15974; CrossRef A. N. Aleshin, Adv. Mater., 2006, 18, 171; CrossRef C. Bae, H. Yoo, S. Kim, K. Lee, J. Kim, M. M. Sung, and H. Shin, Chem. Mater., 2008, 20, 756; CrossRef H. D. Tran, D. Li, and R. B. Kaner, Adv. Mater., 2009, 21, 1487. CrossRef
4. R. Umeda, H. Awaji, T. Nakahodo, and H. Fujihara, J. Am. Chem. Soc., 2008, 130, 3240; CrossRef H. Awaji, T. Nakahodo, and H. Fujihara, Chem. Commun., 2011, 47, 3547; CrossRef K. Sato, T. Nakahodo, and H. Fujihara, Chem. Commun., 2011, 47, 10067; CrossRef M. Mitsumori, T. Nakahodo, and H. Fujihara, Nanoscale, 2012, 4, 117. CrossRef
5. S. Inagi, K. Naka, and Y. Chujo, J. Mater. Chem., 2007, 17, 4122; CrossRef J. R. Heath, Annu. Rev. Mater. Sci., 2009, 39, 1; CrossRef D. Canevet, M. Salle, G. Zhang, D. Zhang, and D. Zhu, Chem. Commun., 2009, 2245; CrossRef H. L. Anderson, Nature Chem., 2010, 2, 12. CrossRef
6. A. Charlton, M. Kalaji, P. J. Murphy, S. Salmaso, A. E. Underhill, G. Williams, M. B. Hursthouse, and K. M. A. Malik, Synth. Metals, 1998, 95, 75. CrossRef
7. The synthesis of 1-TTF and 2-TTF will be reported in a full paper.