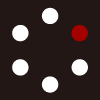
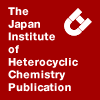
HETEROCYCLES
An International Journal for Reviews and Communications in Heterocyclic ChemistryWeb Edition ISSN: 1881-0942
Published online by The Japan Institute of Heterocyclic Chemistry
e-Journal
Full Text HTML
Received, 2nd July, 2013, Accepted, 26th July, 2013, Published online, 30th July, 2013.
DOI: 10.3987/COM-13-S(S)78
■ Preparation and Spectroscopic Study of Alternate meta-Ethynylpyridine Oligomer Involving 2,4,6-Trisubstituted and 3,5-Disubstituted Pyridine Rings
Hajime Abe,* Daiki Suzuki, Ayako Shimizu, and Masahiko Inouye*
Graduate School of Pharmaceutical Sciences, University of Toyama, Sugitani 2630, Toyama, 930-0194, Japan
Abstract
A new type of ethynylpyridine cooligomer which consists of 3,5- and 2,6-pyridylene units alternately linked with acetylene bonds was prepared by repeating Sonogashira reaction. 1H NMR study suggested that this cooligomer associates with methanol by hydrogen-bonding at the pyridine units. Intramolecular excimer emission was observed around 540 nm by fluorescence measurement, indicating the formation of helical conformation. Weak induced CD was observed by the addition of octyl glucoside as a guest.INTRODUCTION
Helical structures of molecular level have attracted the interests of scientists in various fields. In order to build well-organized helical oligomers and polymers based on a heterocyclic main chain, a number of strategies have been applied.1 For example, heterohelicenes: very rigid structure can force the helical structure;2 helicates: the helical structure is held by coordination with metal centers;3 supramolecules: non-covalent interaction as hydrogen bonding and solvophobic effects can contribute to the helix formation. Intramolecular interaction, intermolecular dimerization (or more), and host−guest interaction can be adopted;4 and conformers: curving conformation suitable for the helix formation can be stabilized by electrostatic effects. In some cases, two kinds of N-heterocycles were alternately oligomerized to form a helix.5 Without any appropriate strategy, the oligomers and polymers usually tend to form a disordered structure such as random-coil.
So far, our group has developed meta-ethynylpyridine oligomers and polymers 1 (Figure 1), which consist of pyridine rings linked with acetylene bonds at 2,6-positions.6−8 These polymers and oligomers prefer transoid conformation, because of the local dipole moment at each pyridine ring. The addition of a saccharide guest stabilized the cisoid conformation by multiple hydrogen-bonding, and the resulting helical complex showed induced CD due to the chirality transfer from the guest to the helix.6a Recently, we reported heterocyclic cooligomers 2, which have 1H-4-pyridone rings in addition to pyridine rings, and these two kinds of rings were linked alternately by acetylene bonds.7 Because the pyridine and pyridone rings have the local dipolar moments in the opposite direction, the cisoid conformation could be stabilized in their own right. This situation made the cooligomers favorable in the helical structures without addition of the saccharide guests. The cooligomers 2 proved to have strong saccharide recognition ability by virtue of the helical conformation. Next we planned to make another kind of alternate ethynylpyridine cooligomer 3 to build a helix. This cooligomer consisted of six 2,6-pyridylene and five 3,5-pyridylene groups linked with acetylene bonds. Figure 2 shows comparison between conformers of model compounds 3a by DFT calculation. The U-shape conformer of 3a was predicted to be 0.61 kJ/mol more stable than N-shape one, therefore the helical structure of 3 was expected to exist more than the case of 1. These prospects encouraged us to develop 3 as a helical device. The 3-butenyloxy groups at 2,6-pyridylene moieties were introduced at the aim of improving solubility and fixing the helix afterward by alkene metathesis reaction.
RESULTS AND DISCUSSION
The alternate cooligomer 3 was prepared from three kinds of building blocks 4, 5, and 6 via sequential Sonogashira coupling reaction. Both the 2,4,6-trisubstituted pyridine blocks 4 and 5 were obtained from 2,6-dibromopyridine,8 and 3,5-diiodopyridine 6 was obtained from 3,5-dibromopyridine.9 The Sonogashira reaction using 4 with an excess of 6 gave diiodo-trimer 7. The coupling of 6 with two equivalents of 5 gave trimer 8, and the two tert-butyldimethylsilyl groups were removed by TBAF to give 9. This trimer 9 was further extended to nonamer 10 by the treatment with an excess of diiodide 7. Finally, the two 3-iodopyridyl moieties at the both ends of 10 was coupled with two equivalent of 5 to afford the targeted alternate undecamer 3, which was identified by 1H NMR in CDCl3 and ESI-TOF-HRMS measurements. Chloroform could dissolve 3 moderately, whereas 3 was practically insoluble in more polar acetonitrile or methanol.
Spectroscopic properties were studied for the cooligomer 3. A solvent effect was observed in 1H NMR measurements in CDCl3 and CDCl3/CD3OD (2:5) as shown in Figure 3. It was found that most of aromatic 1H signals shifted downfield in CDCl3/CD3OD compared with that in CDCl3 (Table 1) except the protons at 2,6-positions of 3,5-disubstituted pyridine rings. On the other hand, remarkable downfield shifts were observed for protons at 4-positions of 3,5-disubstituted pyridine rings. Among the aliphatic protons, -OCH2- ones at 3-butenyloxy side chains showed about 0.04 ppm downfield shift in CDCl3/CD3OD, though other aliphatic ones were less affected (< 0.02 ppm shift).
These position-depended downfield shifts could be rationalized by considering hydrogen-bonding of two adjacent pyridine units with methanol. Figure 4 represents hydrogen-bonding of the expected push-pull manner between N-/U-shape model 3a and methanol, of which stability was evaluated by DFT study. There have been supramolecular helical structures in which hydrogen-bonding of C-H hydrogen atoms on N-containing heterocycles are important.1,4 When the hydrogen atom of the hydroxy group in methanol associated with 4-alkoxypyridine unit of 3a, the oxygen atom of the hydroxy group is able to approach the 2- and 4-H atoms of 3,5-disubstituted pyridine unit, according to the conformation. The 1H NMR observation and DFT calculation would suggest the advantage of 3a of U-shape conformation, in which the 4-H atom of 3,5-disubstututed pyridine unit is more affected by hydrogen-bonding.
UV-vis and fluorescence spectra were measured in CH2Cl2 solutions of undecamer 3 and trimer 8 in order to study the length effect. On comparison of UV-vis spectra for 3 and 8 (Figure 5), no remarkable movement of λmax was observed. The difference of absorption strength would be due to the difference of the number of pyridine rings. On the other hand, in fluorescence spectrum of 3 (λex = 320 nm) a structureless broad band was observed around 540 nm (Figure 6). Because the corresponding emission was not observed in the case of 8, this band could be attributed to intramolecular excimer emission. Appearance of the intramolecular excimer emission in 3 indicates the interaction of the both ends of the longer cooligomer at the excited state. A similar emission was also observed in the case of meta-ethynylpyridine oligomers and polymers in our early work,6a and in those cases shorter cooligomers also showed no excimer emission. The much smaller Storks shift for 3 than that for 8 would be due to the rigidity of 3 brought by its higher-order structure.
Finally, saccharide recognition ability10 of 3 was tried by using octyl β-D-glucopyranoside (Glc) as a guest. When an excess of Glc was added to a CH2Cl2 solution of 3, a typical CD band was induced around 335 nm (Figure 7), which indicate the translation of the chirality of the glucoside into the chirality of higher-order structure of 3, as well as meta-ethynylpyridine oligomers and polymers we have reported so far.6−8 The strength of induced CD shown in Figure 7 was much weaker compared to that in the cases of usual poly- and oligo(ethynylpyridines) 1 with a glucoside guest. When 3 makes a helix as expected, the nitrogen atoms of 2,6-pyridylene units direct to inside of the helix and will behave as hydrogen-bonding sites. On the hand, the nitrogen atoms of 3,5-pyridylene units direct to outside and are useless for incorporating a guest. Thus, the alternation in the structure of 3 brought an advantage for forming a helix by a dipole effect, whereas brought a disadvantage for decreasing the number of recognition sites.
In conclusion, we designed and prepared a new type of ethynylpyridine cooligomer 3 which consists of 3,5- and 2,6-pyridylene units alternately linked with acetylene bonds. 1H NMR experiments of CDCl3 and CDCl3/CD3OD solutions and DFT calculation suggested that cooligomer 3 associates with methanol by hydrogen-bonding in a push-pull manner by adjacent pyridine units. Fluorescence spectra showed an intramolecular excimer emission as a broad band around 540 nm, which supported the formation of helical conformation. Weak glucoside recognition ability was observed by an induced CD measurement, though the CD was much weaker due to the alternation in the structure of 3.
We are now surveying the suitable reaction conditions to bridge the 3-butenyl side chains of 3 by alkene metathesis procedure to fix the helical conformation, which is expected as an saccharide recognition host or a chiral reaction environment.
EXPERIMENTAL
General. NMR spectra were recorded on a 300 MHz NMR spectrometer using tetramethylsilane (TMS) as an internal reference. Melting points were not corrected. THF was freshly distilled from sodium benzophenone ketyl before use.
Starting Materials. 4-(3-Butenyloxy)-2,6-diethynylpyridine (4),8 4-(3-butenyloxy)-2-(tert-butyl- dimethylsilylethynyl)-6-ethynylpyridine (5),8 and 3,5-diiodopyridine (6)9 were prepared as reported in the literature. All reactions were carried out under an argon atmosphere.
4-(3-Butenyloxy)-2,6-bis(5-iodo-3-pyridylethynyl)pyridine (7). To a mixture of 4-(3-butenyloxy)- 2,6-diethynylpyridine (4, 0.10 g, 0.51 mmol), 3,5-diiodopyridine (6, 0.63 g, 2.0 mmol), Pd(PPh3)4 (24 mg, 21 µmol), i-Pr2NH (2.5 mL), and THF (20 mL) was added CuI (3.9 mg, 20 µmol), and the mixture was stirred for 5 h at room temperature. The resulting mixture was treated with a Florisil bed and given a rinse with AcOEt. The filtrate was concentrated by a rotary evaporator and the resulting residue was subjected to silica gel column chromatography (eluent: CHCl3 to CHCl3/MeOH = 50:1) to give 7 as a colorless solid (0.16 g, 52%). Mp 196−198 °C; IR (KBr) ν 3047, 2925, 2222, 1582, 1550 cm−1; 1H NMR (CDCl3) δ 2.57−2.64 (m, 2 H), 4.13 (t, J = 6.6 Hz, 2 H), 5.15−5.23 (m, 2 H), 5.82−5.95 (m, 1 H), 7.07 (s, 2 H), 8.21 (s, 2 H), 8.73 (s, 2 H), 8.81 (s, 2 H); 13C NMR (CDCl3) δ 33.2, 67.8, 84.0, 92.1, 92.4, 113.9, 117.9, 120.8, 133.1, 143.8, 146.6, 150.7, 155.2, 165.1; HRMS (ESI-TOF) calcd for C23H16I2N3O (M + H+): 603.9383; m/z found: 603.9362.
3,5-Bis(4-(3-butenyloxy)-6-(tert-butydimethylsilylethynyl)-2-pyridylethynyl)pyridine (8). To a mixture of 3,5-diiodopyridine (6, 0.28 g, 0.83 mmol), Pd(PPh3)4 (39 mg, 33 µmol), CuI (6.3 mg, 33 µmol), i-Pr2NH (2.5 mL), and THF (10 mL) was added 4-(3-butenyloxy)-2-(tert-butyl- dimethylsilylethynyl)-6-ethynylpyridine (5, 0.52 g, 1.7 mmol), and the mixture was stirred for 4 h at room temperature. The resulting mixture was treated with a Florisil bed and given a rinse with THF. The filtrate was concentrated by a rotary evaporator and the resulting residue was subjected to silica gel column chromatography (eluent: hexane/AcOEt = 8:1 to 4:1) to give 8 as a yellow solid (0.54 g, 93%). Mp 79−81 °C; IR (KBr) ν 3078, 2953, 2928, 2857, 2225, 2164, 1579, 1552 cm−1; 1H NMR (CDCl3) δ 0.20 (s, 12 H), 1.00 (s, 18 H), 2.54−2.61 (m, 4 H), 4.11 (t, J = 6.6 Hz, 4 H), 5.13−5.23 (m, 4 H), 5.81−5.94 (m, 2 H), 6.98 (s, 2 H), 7.03 (s, 2 H), 7.98 (s, 1 H), 8.74 (s, 2 H); 13C NMR (CDCl3) δ −4.7, 16.7, 33.1, 67.6, 84.2, 92.0, 93.6, 103.6, 113.5, 114.0, 117.6, 119.0, 133.2, 141.1, 143.5, 144.5, 151.6, 164.8; HRMS (ESI-TOF) m/z calcd for C43H52N3O2Si2 (M + H+): 698.3598; found: 698.3598.
3,5-Bis(4-(3-butenyloxy)-6-ethynyl-2-pyridylethynyl)pyridine (9). A mixture of 8 (0.12 g, 0.17 mmol), tetrabutylammonium fluoride (TBAF, 1.0 M in THF, 0.50 mL, 0.50 mmol), THF (10 mL), and three drops of water was stirred for 1 h at room temperature. The resulting mixture was concentrated by a rotary evaporator and the resulting residue was subjected to silica gel column chromatography (eluent: CHCl3/MeOH = 60:1) to give 9 as a brown solid (70 mg, 90%). Mp 74−77 °C; IR (KBr) ν 3289, 3075, 2936, 2225, 2108, 1581, 1551 cm−1; 1H NMR (CDCl3) δ 2.55−2.62 (m, 4 H), 3.15 (s, 2 H), 4.11 (t, J = 6.6 Hz, 4 H), 5.13−5.23 (m, 4 H), 5.81−5.94 (m, 2 H), 7.01 (s, 2 H), 7.07 (s, 2 H), 7.99 (s, 1 H), 8.75 (s, 2 H); 13C NMR (CDCl3) δ 33.2, 67.7, 82.1, 84.4, 91.8, 113.9, 114.0, 117.8, 119.0, 133.2, 141.1, 143.7, 151.8, 165.0; HRMS (ESI-TOF) m/z calcd for C31H24N3O2 (M + H+): 470.1869; found: 470.1862.
Diiodo-nonamer 10. A mixture of tandem diyne 9 (58 mg, 0.12 mmol), diiodide 7 (0.21 g, 0.34 mmol), PdCl2(PPh3)2 (3.5 mg, 5.0 µmol), CuI (0.9 mg, 5.0 µmol), i-Pr2NH (2.5 mL), and THF (30 mL) was stirred for 5.5 h at 50 °C. The resulting mixture was treated with a Florisil bed and given a rinse with CHCl3. The filtrate was concentrated by a rotary evaporator and the resulting residue was subjected to silica gel column chromatography (eluent: CHCl3/MeOH = 75:1 to 10:1) to give 10 as a red solid (95 mg, 54%). Mp 178−179 °C; IR (KBr) ν 3072, 2923, 2850, 2222, 1581, 1549 cm−1; 1H NMR (CDCl3) δ 2.57−2.64 (m, 8 H), 4.12−4.18 (m, 8 H), 5.15−5.24 (m, 8 H), 5.82−5.95 (m, 4 H), 7.07−7.11 (m, 8 H), 8.01−8.03 (m, 3 H), 8.22 (s, 2 H), 8.73−8.81 (m, 10 H); 13C NMR (CDCl3) δ 33.0, 67.7, 84.5, 91.7, 92.0, 113.8, 117.7, 118.8, 133.0, 141.1, 143.7, 143.8, 146.5, 150.6, 151.7, 155.1, 165.0; HRMS (ESI-TOF) m/z calcd for C77H52I2N9O4 (M + H+): 1420.2232; found: 1420.2231.
Bis(TBDMS-ethynyl)-undecamer 3. A mixture of 5 (40 mg, 0.13 mmol), diiodide 10 (38 g, 27 µmol), Pd(PPh3)4 (6.2 mg, 5.3 µmol), and N-methylmorpholine (NMM, 15 mL) was stirred for 7 h at 55 °C and additionally for 17 h at 65 °C. The resulting mixture was treated with a Florisil bed and given a rinse with CHCl3. The filtrate was concentrated by a rotary evaporator and the resulting residue was subjected to silica gel column chromatography (eluent: CHCl3 to CHCl3/MeOH = 100:1) to give 3 as a pink solid (28 mg, 59%). Mp 139−140 °C; IR (KBr) ν 3074, 2928, 2855, 2224, 2162, 1581, 1550 cm−1; 1H NMR (CDCl3) δ 0.20 (s, 12 H), 1.00 (s, 18 H), 2.55−2.64 (m, 12 H), 4.08−4.18 (m, 12 H), 5.13−5.25 (m, 12 H), 5.81−5.94 (m, 6 H), 6.98 (s, 2 H), 7.01 (s, 8 H), 8.00−8.03 (m, 5 H), 8.73−8.79 (m, 10 H); 13C NMR (CDCl3) δ −4.7, 16.7, 26.2, 33.2, 67.6, 67.8, 84.2, 84.6, 84.7, 91.7, 91.8, 92.0, 93.7, 103.7, 113.6, 113.9, 114.0, 117.7, 117.8, 119.0, 119.1, 133.2, 133.3, 141.2, 143.5, 143.9, 144.6, 151.7, 151.8, 164.9, 165.1; HRMS (ESI-TOF) m/z calcd for C115H100N11O6Si2 (M + H+): 1786.7397; found: 1786.7391.
References
1. Recent reviews for helical foldamers: 'Foldamers,' ed. by S. Hecht and I. Huc, Wiley-VCH, Weinheim, 2007; 'Supramolecular Chirality,' Top. Curr. Chem., Vol. 265, ed. by M. Crego-Calama and D. N. Reinhoudt, Springer-Verlag, Berlin, 2007; D. J. Hill, M. J. Mio, R. B. Price, T. S. Hughes, and J. S. Moore, Chem. Rev., 2001, 101, 3893; CrossRef T. Nakano and Y. Okamoto, Chem. Rev., 2001, 101, 4013; CrossRef C. R. Ray and J. S. Moore, Adv. Polym. Sci., 2005, 177, 91; CrossRef B. Gong, Acc. Chem. Res., 2008, 41, 1376; CrossRef E. Yashima, K. Maeda, H. Iida, Y. Furusho, and K. Nagai, Chem. Rev., 2009, 109, 6102; CrossRef H. Juwarker, J.-M. Suk, and K.-S. Jeong, Chem. Soc. Rev., 2009, 38, 3316; CrossRef B.-B. Ni, Q. Yan, Y. Ma, and D. Zhao, Coord. Chem. Rev., 2010, 254, 954; CrossRef K. Watanabe, K. Suda, and K. Akagi, J. Mater. Chem. C, 2013, 1, 2797. CrossRef
2. For recent reviews involving the topics of heterohelicenes: Y. Shen and C.-F. Chen, Chem. Rev., 2012, 112, 1463; S. K. Collins and M. P. Vachon, Org. Biomol. Chem., 2006, 4, 2518. CrossRef
3. For recent reviews for helicate: M. Albrecht, Chem. Rev., 2001, 101, 3457; CrossRef M. Albrecht, Top. Curr. Chem., 2005, 248, 105; CrossRef M. Albrecht and R. Fröhlich, Bull. Chem. Soc. Jpn., 2007, 80, 797; CrossRef H. Miyake and H. Tsukube, Chem. Soc. Rev., 2012, 41, 6977. CrossRef
4. For recent reviews for helical foldamers based on supramolecular concepts: E. Yashima, K. Maeda, and T. Nishimura, Chem. Eur. J., 2004, 10, 42; CrossRef I. Huc, Eur. J. Org. Chem., 2004, 17; CrossRef M. Albrecht, Angew. Chem. Int. Ed., 2005, 44, 2; CrossRef H.-J. Kim, Y.-B. Lim, and M. Lee, J. Polym. Sci., Part A: Polym. Chem., 2008, 46, 1925; CrossRef H. Juwarker and K.-S. Jeong, Chem. Soc. Rev., 2010, 39, 3664; CrossRef A. Roy, P. Prabhakaran, P. K. Baruah, and G. J. Sanjayan, Chem. Commun., 2011, 47, 11593. CrossRef
5. For pyridine-pyrimidine systems by Lehn et al.; G. S. Hanan, J.-M. Lehn, N. Kyritsakas, and J. Fischer, J. Chem. Soc., Chem. Commun., 1995, 765; CrossRef D. M. Bassani, J.-M. Lehn, G. Baum, and D. Fenske, Angew. Chem. Int. Ed. Engl., 1997, 36, 1845; CrossRef M. Ohkita, J.-M. Lehn, G. Baum, and D. Fenske, Chem. Eur. J., 1999, 5, 3471; CrossRef M. Ohkita, J.-M. Lehn, G. Baum, and D. Fenske, Heterocycles, 2000, 52, 103; CrossRef K. M. Gardinier, R. G. Khoury, and J.-M. Lehn, Chem. Eur. J., 2000, 6, 4124; CrossRef M. Barboiu and J.-M. Lehn, Proc. Nat. Acad. Sci. U.S.A., 2002, 99, 5201; CrossRef M. Barboiu, G. Vaughan, N. Kyritsakas, and J.-M. Lehn, Chem. Eur. J., 2003, 9, 763; CrossRef for pyridine-pyridazine systems by Lehn et al.; L. A. Cuccia, E. Ruiz, J.-M. Lehn, J.-C. Homo, and M. Schumutz, Chem. Eur. J., 2002, 8, 3448; CrossRef for pyridine-triazole systems by Hecht et al.; R. M. Meudtner and S. Hecht, Angew. Chem., Int. Ed., 2008, 47, 4926. CrossRef
6. (a) M. Inouye, M. Waki, and H. Abe, J. Am. Chem. Soc., 2004, 126, 2022; CrossRef (b) H. Abe, N. Masuda, M. Waki, and M. Inouye, J. Am. Chem. Soc., 2005, 127, 16189; CrossRef (c) M. Waki, H. Abe, and M. Inouye, Chem. Eur. J., 2006, 12, 7839; CrossRef (d) M. Waki, H. Abe, and M. Inouye, Angew. Chem., Int. Ed., 2007, 46, 3059; CrossRef (e) H. Abe, S. Takashima, T. Yamamoto, and M. Inouye, Chem. Commun., 2009, 2121; CrossRef (f) S. Takashima, H. Abe, and M. Inouye, Chem. Commun., 2011, 47, 7455; CrossRef (g) S. Takashima, T. Yamamoto, H. Abe, and M. Inouye, Heterocycles, 2012, 84, 355; CrossRef (h) S. Takashima, H. Abe, and M. Inouye, Chem. Commun., 2011, 47, 7455; CrossRef (i) H. Abe, H. Makida, and M. Inouye, Tetrahedron, 2012, 68, 4353; CrossRef (j) H. Abe, Y. Ohishi, and M. Inouye, J. Org. Chem., 2012, 77, 5209; CrossRef (k) H. Abe, K. Okada, H. Makida, and M. Inouye, Org. Biomol. Chem., 2012, 10, 6930; CrossRef (l) H. Abe, H. Makida, and M. Inouye, Heterocycles, 2012, 86, 955; CrossRef (m) F. Kayamori, H. Abe, and M. Inouye, Eur. J. Org. Chem., 2013, 1677; CrossRef (n) S. Takashima, H. Abe, and M. Inouye, Tetrahedron: Asymmetry, 2013, 24, 527. CrossRef
7. H. Abe, H. Machiguchi, S. Matsumoto, and M. Inouye, J. Org. Chem., 2008, 73, 4650; CrossRef See also: H. Abe, Y. Chida, H. Kurokawa, and M. Inouye, J. Org. Chem., 2011, 76, 3366. CrossRef
8. H. Abe, D. Murayama, F. Kayamori, and M. Inouye, Macromolecules, 2008, 41, 6903. CrossRef
9. M. Peters, M. Trobe, and R. Breinbauer, Chem. Eur., J., 2013, 19, 2450. CrossRef
10. Recent reviews for saccharide recognition by synthetic hosts: Host-Guest Chemistry-Mimetic Approaches to Study Carbohydrate Recognition, in Top. Curr. Chem., ed. by S. Penadés, Springer-Verlag, Berlin, 2002, Vol. 218; A. P. Davis and T. D. James, Functional Synthetic Receptor, Springer-Verlag, Weinheim, 2005; A. P. Davis and R. S. Wareham, Angew. Chem. Int. Ed., 1999, 38, 2978; CrossRef M. Mazik, Chem. Soc. Rev., 2009, 38, 935. CrossRef