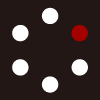
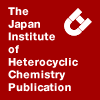
HETEROCYCLES
An International Journal for Reviews and Communications in Heterocyclic ChemistryWeb Edition ISSN: 1881-0942
Published online by The Japan Institute of Heterocyclic Chemistry
e-Journal
Full Text HTML
Received, 15th April, 2013, Accepted, 29th May, 2013, Published online, 31st May, 2013.
DOI: 10.3987/COM-13-S(S)6
■ Cu/HP20-Catalyzed Solvent-Free Huisgen Cycloaddition at Ordinary Temperatures
Yoshiaki Kitamura, Kazumi Taniguchi, Tomohiro Maegawa, Yasunari Monguchi, Yukio Kitade, and Hironao Sajiki*
Laboratory of Organic Chemistry, Department of Pharmaceutical Sciences, Gifu Pharmaceutical University, 1-25-4 Daigakunishi, Gifu 501-1196, Japan
Abstract
We have developed an environmentally friendly and highly efficient solvent-free Cu(I)-catalyzed azide-alkyne cycloaddition (CuAAC) reaction using a polymer-supported copper catalyst (Cu/HP20). Substrates poorly soluble in common organic solvents are also applicable to the present cycloaddition reaction without any solvents and provide the corresponding 1,4-triazole in high yields.INTRODUCTION
In recent years, environmentally-friendly reaction processes have been vigorously studied on the basis of green chemistry. One of the major environmental impacts of organic synthesis is the solvent use, and the reaction systems without a solvent have attracted much attention. The use of heterogeneous catalysts instead of stoichiometric amounts of reagents or homogeneous catalysts in a solvent-free reaction is also highly recommended since only the appropriate and small amount of solvent(s) to dissolve the organic products and residual reagents is required to separate the catalyst from the reaction mixture. We have recently reported the solvent-free and heterogeneous Pd/C-catalyzed hydrogenation and Suzuki-Miyaura coupling reaction.1
The Cu(I)-catalyzed azide-alkyne cycloaddition (CuAAC), independently developed by Sharpless2 and Meldal,3 has become the most remarkable example of click chemistry and has been widely applied in organic syntheses as well as the medicinal and process chemistry fields.4 Although a number of CuAAC methods using homogeneous catalysts have been developed to date,5 most of the procedures have some drawbacks, such as the use of a significant amount of expensive and/or difficult-to-prepare catalysts, and/or organic solvents, etc. Likewise, numerous heterogeneous (supported) copper catalysts have been applied to the CuAAC methods,6 and Kiser7 and Fokin8 independently developed transition-metal-free azide-alkyne cycloadditions, while the use of organic solvents is required for these reactions. A few solvent-free and heterogeneous CuAACs were recently reported, but these methods required elevated temperature conditions9 and special equipment, such as a microwave10 and a ball-mill.11 However, the Amberlyst A21-supported copper (I) catalyst12 is an exception to this requirement.
We have recently reported a newly-developed solvent-free CuAAC using a copper catalyst (Cu/CR11) supported on a polystyrene-divinylbenzene-based chelate resin possessing iminodiacetic acid moieties as a tridentate ligand, DIAION CR11 (Mitsubishi Chemical Corporation).13 Although Cu/CR11 is a highly dispersed heterogeneous catalyst, the application of heat (70 ºC) was required for the efficient reaction progress due to the tight chelation of copper to the resin. On the other hand, we have also previously developed a polystyrene-divinylbenzene-based polymer, DIAION HP20 (Mitsubishi Chemical Corporation)-supported copper catalyst (1% Cu/HP20) and used it as a catalyst for CuAAC under mild conditions with an organic solvent, such as toluene, at room temperature.6v In addition, we have disclosed the preparation of the HP20-supported palladium catalyst (10% Pd/HP20)14 and demonstrated the application to the ligand-free cross-coupling reaction.15 Since HP20 is a polystyrene-divinylbenzene-based polymer possessing a high-specific surface area (ca. 590 m2/g) without chelating functionalities within the molecule, it is anticipated to have a high catalyst activity even under solvent-free conditions due to the loose interaction between the Cu species and HP20. Therefore, we explored the application of Cu/HP20 to the solvent-free CuAAC.
RESULTS AND DISCUSSION
We investigated the CuAAC using 4-fluorobenzylazide (1a) and 3-methyl-1-butyn-3-ol (2a) as substrates under solvent-free conditions. In the presence of 1% Cu/HP20 (2.0 mol% versus 1a) and Et3N (1.1 equiv versus 1a),16 the cycloaddition was completed within 4 h at room temperature (Table 1, Entry 1). The use of Cu/HP20 and Et3N was then optimized because the reduction of catalysts and reagents is an important issue for an industrial application. The use of 1% Cu/HP20 could be reduced to 1.0 mol% without significant reduction of the reactivity (Table 1, Entries 1 and 2). A further reduction of Cu/HP20 (0.5 mol% and 0.1 mol%) obviously suppressed the reaction progress, and the conversion yields of the Huisgen adduct (3a) decreased with the lower use of Cu/HP20 [83% (0.5 mol%) and 25% (0.1 mol%), respectively] (Entries 3 and 4). The reaction efficiency did not decrease with the reduced use of Et3N down to 0.22 equiv, although the reaction was incomplete with 0.11 equiv of Et3N even after 24 h (Entries 2 and 6–9). The addition of both Cu/HP20 and Et3N was indispensable for the reaction progress, since no cycloaddition took place without Cu/HP20 or Et3N (Entries 5 and 10).16 We then confirmed the substrate applicability of the present solvent-free cycloaddition of alkynes with azides in the presence of 1% Cu/HP20 (1.0 mol%) and 0.22 equiv of Et3N.
As shown in Table 2, a wide range of azides (1a–g) efficiently reacted with 3-methyl-1-butyn-3-ol (2a) even though the reaction was performed at room temperature to give the corresponding Huisgen adducts (3a–g) in nearly quantitative isolated yields (Entries 2–7). It is noteworthy that the cycloaddition of 3,5-dinitrobenzylazide (1c) with 2a also smoothly occurred at room temperature under solvent-free conditions, although the reaction in toluene as a solvent never proceeded at room temperature and could be completed by heating at 60 ºC (Entry 3).6v
Various terminal mono-substituted alkynes (2a–f) were also found to react with 4-fluorobenzylazide (1a) to generate the corresponding 1,4-disubstituted triazoles (3) in moderate to quantitative yields (Table 3). When 3-methyl-1-butyn-3-ol (2a) and 2-ethynylpyridine (2e) were used as substrates, the cyclization proceeded in a very efficient way, since heteroatoms (O and N, respectively) adjacent to the alkynes would presumably facilitate the approach to the Cu species of their C–C triple bonds (Entries 1 and 5). On the other hand, conjugated terminal alkynes of ethynylbenzene (2c) and 1-ethynylcyclohexane (2d)
without the neighboring-group participation indicated lower reactivities (Entries 3 and 4).
Next, the reuse test of 1% Cu/HP20 using 4-fluorobenzylazide (1a) and 3-methyl-1-butyn-3-ol (2a) as substrates was examined. The reaction efficiency significantly decreased with the number of reuses (Table 4). To confirm the reason for the degradation of the catalyst activity, the leaching of the copper species from Cu/HP20 was then measured using inductively coupled plasma atomic emission spectrometry (ICP-AES).17 The amount of leached copper was approximately 19%, thus the leaching of copper species might be the cause of the difficult reuse of Cu/HP20.
CONCLUSIONS
We have developed a solvent-free 1% Cu/HP20-catalyzed Huisgen cycloaddition reaction at room temperature. The cycloaddition between azides (1) and terminal alkynes (2) in the presence of 1 mol% of 1% Cu/HP20 and only 0.22 equiv (versus azides) of Et3N has been regioselectively achieved under totally solvent-free and mild conditions to give the corresponding 1,4-disubstituted thiazoles (3). It is particularly worth noting that the reaction could be easily achieved at room temperature. Although significant amounts of copper species were leached from Cu/HP20, it possesses a significantly strong catalyst activity due to the weak interaction with HP20 as a support compared to the Cu catalyst supported on a chelate resin (Cu/CR1114). The present methodology will find many applications for the synthesis of heat-labile triazole-containing molecules.
EXPERIMENTAL
General
All reactions were carried out under argon. The deionized water was purchased from Wako Pure Chemical Industries, Ltd. HP20 was a gift from the Mitsubishi Chemical Co. The azides were prepared according to the known procedure.10 The terminal alkynes were purchased from Tokyo Chemical Industry Co., Ltd. Et3N and the solvents were purchased from Nacalai Tasque, Inc. or Wako Pure Chemical Industries, Ltd. The commercial reagents and solvents were used without further purification. Flash column chromatography was performed using Kanto Chemical Co., Inc. silica gel 60N, spherical neutral (63–210 µm).
General Procedure for 1% Cu/HP20-catalyzed CuAAC under solvent-free conditions
A mixture of the azide (1.0 mmol), the terminal alkyne (1.1 mmol), Et3N (30.4 μL, 220 μmol), and 1% Cu/HP20 (63.5 mg, 10 μmol) in a test tube was stirred at room temperature. After a given period, the mixture was diluted with H2O (2 mL) and EtOAc (2 mL), and passed through a filter paper. The filtrate was separated into two layers, and the aqueous layer was extracted with EtOAc (2 × 10 mL). The combined organic layers were washed with brine (10 mL), dried over Na2SO4, and concentrated in vacuo. The residue was purified by flash column chromatography on silica gel to give the corresponding 1,4-triazole, the structure of which was confirmed by comparison to the literature structure.
2-[1-(4-Fluorobenzyl)-1H-1,2,3-triazol-4-yl]propan-2-ol (3a)6v: Pale brown solid; 1H NMR (CDCl3) δ 7.34 (s, 1H), 7.27 (dd, J = 8.7 Hz, 5.3 Hz, 2H), 7.07 (t, J = 8.7 Hz, 2H), 5.47 (s, 2H), 1.61 (s, 6H); 13C NMR (CDCl3) δ 162.5 (d, J = 247.0 Hz), 156.1, 130.4 (d, J = 3.3 Hz), 129.7 (d, J = 8.2 Hz), 119.2, 115.7 (d, J = 21.4 Hz), 68.1, 52.9, 30.2; MS (EI) C12H15N3OF (M+) 236.
2-(1-Benzyl-1H-1,2,3-triazol-4-yl)propan-2-ol (3b)18: Colorless solid; 1H NMR δ 7.40–7.34 (m, 4H), 7.29–7.26 (m, 2H), 5.50 (s, 2H), 1.61 (s, 6H); 13C NMR δ 156.0, 134.6, 129.0, 128.6, 128.0, 119.1, 68.4, 54.0, 30.3; MS (EI) C12H15N3O (M+) 217.
2-[1-(3,5-Dinitrobenzyl)-1H-1,2,3-triazol-4-yl]propan-2-ol (3c)6v: Pale yellow solid; 1H NMR (DMSO-d6) δ 8.79 (s, 1H), 8.65 (s, 2H), 8.09 (s, 1H), 5.87 (s, 2H), 5.13 (s, 1H), 1.44 (s, 6H); 13C NMR (DMSO-d6) δ 156.4, 148.2, 140.1, 128.8, 121.2, 118.4, 67.0, 50.9, 30.6; MS (EI) C12H13N5O5 (M+) 307.
2-[1-(4-Methoxybenzyl)-1H-1,2,3-triazol-4-yl]propan-2-ol (3d)6v: Yellow solid; 1H NMR (CDCl3) δ 7.37 (s, 1H), 7.21 (d, J = 8.8 Hz, 2H), 6.87 (d, J = 8.8 Hz, 2H), 5.39 (s, 2H), 3.78 (s, 3H), 1.58 (s, 6H); 13C NMR (CDCl3) δ 159.6, 129.5, 126.5, 126.5, 118.9, 114.2, 68.2, 55.1, 53.4, 30.3; MS (EI) C13H17N3O2 (M+) 247.
2-(1-Phenyl-1H-1,2,3-triazol-4-yl)propan-2-ol (3e)6v: Yellow solid; 1H NMR (CDCl3) δ 8.04 (s, 1H), 7.67 (d, J = 8.1 Hz, 2H), 7.46–7.42 (m, 3H), 1.71 (s, 6H); 13C NMR (CDCl3) δ 156.5, 136.8, 129.4, 128.4, 120.2, 117.8, 68.3, 30.3; MS (EI) C11H13N3O (M+) 203.
2-[1-(3-Phenylpropyl)-1H-1,2,3-triazol-4-yl]propan-2-ol (3f)6v: Yellow oil; 1H NMR (CDCl3) δ 7.45 (s, 1H), 7.28 (t, J = 7.2 Hz, 2H), 7.21–7.14 (m, 3H), 4.29 (t, J = 7.2 Hz, 2H), 2.64 (t, J = 7.2 Hz, 2H), 2.21 (quint, J = 7.2 Hz, 2H), 1.63 (s, 6H); 13C NMR (CDCl3) δ 140.0, 128.4, 128.2, 126.1, 119.2, 68.2, 49.3, 32.4, 31.4, 30.3; MS (EI) C14H19N3O (M+) 245.
{4-[4-(2-Hydroxypropan-2-yl)-1H-1,2,3-triazol-1-yl]phenyl}phenylmethanone (3g)6v: Pale brown solid; 1H NMR (CDCl3) δ 8.05 (s, 1H), 7.96 (d, J = 8.5 Hz, 2H), 7.88 (d, J = 8.5 Hz, 2H), 7.81 (d, J = 7.3 Hz, 2H), 7.63 (t, J = 7.3 Hz, 1H), 7.52 (t, J = 7.3 Hz, 2H), 1.73 (s, 6H); 13C NMR (CDCl3) δ 195.2, 139.7, 137.3, 137.0, 132.8, 131.7, 129.9, 128.5, 119.8, 68.7, 30.5; MS (EI) C18H17N3O2 (M+) 307.
3-[1-(4-Fluorobenzyl)-1H-1,2,3-triazol-4-yl]propan-1-ol (3h)6v: Pale yellow solid; 1H NMR (CDCl3) δ 7.41 (s, 1H), 7.25 (dd, J = 8.7 Hz, 5.3 Hz, 2H), 7.01 (t, J = 8.7 Hz, 2H), 5.43 (s, 2H), 3.97 (s, 1H), 3.85 (t, J = 6.3 Hz, 1H), 2.89 (t, J = 6.3 Hz, 1H); 13C NMR (CDCl3) δ 162.6 (d, J = 248.8 Hz), 145.7, 130.5 (d, J = 3.3 Hz), 129.7 (d, J = 8.2 Hz), 121.6, 115.7 (d, J = 22.0 Hz), 61.0, 53.0, 28.6; MS (EI) C11H12N3OF (M+) 221.
1-(4-Fluorobenzyl)-4-phenyl-1H-1,2,3-triazole (3i)6v: Colorless solid; 1H NMR (CDCl3) δ 7.79 (d, J = 7.2 Hz, 2H), 7.69 (s, 1H), 7.37 (t, J = 7.2 Hz, 2H), 7.31–7.24 (m, 3H), 7.02 (t, J = 8.6 Hz, 2H), 5.48 (s, 2H); 13C NMR (CDCl3) δ 162.1 (d, J = 246.2 Hz), 148.1, 130.5 (d, J = 3.1 Hz), 130.3, 129.8 (d, J = 8.2 Hz), 128.7, 128.1, 125.5, 119.4, 115.9 (d, J = 22.0 Hz), 53.2; MS (EI) C15H12N3F (M+) 253.
4-Cyclohexenyl-1-(4-fluorobenzyl)-1H-1,2,3-triazole (3j)6v: Colorless solid; 1H NMR (CDCl3) δ 7.32 (s, 1H), 7.25 (dd, J = 8.5 Hz, 5.4 Hz, 2H), 7.05 (t, J = 8.5 Hz, 2H), 6.50–6.48 (m, 1H), 5.47 (s, 2H), 2.37–2.32 (m, 2H), 2.20–2.15 (m, 2H), 1.76–1.71 (m, 2H), 1.67–1.61 (m, 2H); 13C NMR (CDCl3) δ 162.7 (d, J = 247.0 Hz), 150.0, 130.8 (d, J = 3.3 Hz), 129.7 (d, J = 8.2 Hz), 127.1, 125.1, 118.0, 115.9 (d, J = 21.6 Hz), 53.1, 26.2, 25.1, 22.3, 22.1; MS (EI) C15H16N3F (M+) 257.
2-[1-(4-Fluorobenzyl)-1H-1,2,3-triazol-4-yl]pyridine (3k)6v: Pale brown solid; 1H NMR (CDCl3) δ 8.53 (d, J = 4.8 Hz, 1H), 8.16 (d, J = 7.8 Hz, 1H), 8.08 (s, 1H), 7.74 (dd, J = 7.8 Hz, 6.4 Hz, 1H), 7.33–7.29 (m, 2H), 7.19 (dd, J = 6.4 Hz, 4.8 Hz, 1H), 7.04 (t, J = 8.6 Hz, 2H), 5.54 (s, 2H); 13C NMR (CDCl3) δ 162.7 (d, J = 247.0 Hz), 150.0, 149.2, 148.6, 136.7, 130.2 (d, J = 3.3 Hz), 130.0 (d, J = 8.2 Hz), 122.7, 121.7, 120.0, 115.9 (d, J = 21.6 Hz), 53.3; MS (EI) C14H11N4F (M+) 254.
1-(4-Fluorobenzyl)-4-trimethylsilyl-1H-1,2,3-triazole (3l)6v: Pale yellow solid; 1H NMR (CDCl3) δ 7.47 (s, 1H), 7.27 (dd, J = 8.8 Hz, 5.2 Hz, 2H), 7.04 (t, J = 8.8 Hz, 2H), 5.53 (s, 2H), 0.30 (s, 9H); 13C NMR (CDCl3) δ 162.6 (d, J = 248.6 Hz), 147.1, 130.8 (d, J = 3.3 Hz), 129.8 (d, J = 8.1 Hz), 128.6, 115.8 (d, J = 22.2 Hz), 52.5, -1.3; MS (EI) C12H16N3FSi (M+) 249.
Recycling of 1% Cu/HP20
A mixture of 4-fluorobenzylazide (756 mg, 5.0 mmol), 3-methyl-1-butyn-3-ol (538 μL, 5.5 mmol), Et3N (152 μL, 1.1 mmol), and 1% Cu/HP20 (318 mg, 50 μmol) in a test tube was stirred at room temperature. After 5 h, the mixture was diluted with H2O (10 mL) and EtOAc (10 mL), passed through a filter paper and the catalyst was washed with H2O (2 × 5 mL) and EtOAc (2 × 5 mL). The filtrate was separated into two layers, and the aqueous layer was extracted with EtOAc (2 × 10 mL). The combined organic layers were washed with brine (10 mL), dried over Na2SO4, and concentrated in vacuo. The residue was purified by flash column chromatography on silica gel (CHCl3/MeOH, 100:0~10:1) to give 2-[1-(4-fluorobenzyl)-1H-1,2,3-triazol-4-yl]propan-2-ol (1.17 g, 100%). The recovered the Cu/HP20, which was dried in a desiccator under vacuum for more than 12 h, was used for the 2nd run. The reuse tests were carried out in line with the amount of the recovered catalyst, e.g., for the 2nd run, recovered 1% Cu/HP20 (299 mg, 47 μmol), 4-fluorobenzylazide (710 mg, 4.7 mmol), 3-methyl-1-butyn-3-ol (506 μL, 5.17 mmol), and Et3N (143 μL, 1.03 mmol) were used. The reuse tests were repeated until the 4th run.
Assay of Residual Copper in the Reaction Mixture
A mixture of 4-fluorobenzylazide (756 mg, 5.0 mmol), 3-methyl-1-butyn-3-ol (538 μL, 5.5 mmol), Et3N (152 μL, 1.1 mmol), and 1% Cu/HP20 (318 mg, 50 μmol) in a test tube was stirred at room temperature. After 5 h, the mixture was diluted with H2O (10 mL) and EtOAc (10 mL), passed through a filter paper and the catalyst was wash with H2O (2 × 5 mL) and EtOAc (2 × 5 mL). The filtrate was separated into two layers, and the aqueous layer was extracted with EtOAc (2 × 10 mL). The combined organic layers were concentrated in vacuo and the residue was diluted with EtOH to 50 mL of total volume. The aqueous layer was diluted with H2O to 50 mL of total volume. The residual copper involved in each solution was then assayed using a Shimadzu ICP8000 (Shimadzu, Kyoto, Japan). The concentration of leached copper was 12 ppm (organic layer) and <1 ppm (aqueous layer).
ACKNOWLEDGEMENTS
We thank the Mitsubishi Chemical Corporation for the gift of Diaion HP20. We also thank the N.E. Chemcat Corporation for the ICP-AES measurements.
References
1. Y. Monguchi, Y. Fujita, S. Hashimoto, M. Ina, T. Takahashi, R. Ito, K. Nozaki, T. Maegawa, and H. Sajiki, Tetrahedron, 2011, 67, 8628. CrossRef
2. V. V. Rostovtsev, L. G. Green, V. V. Fokin, and K. B. Sharpless, Angew. Chem. Int. Ed., 2002, 41, 2596. CrossRef
3. C. W. Tornøe, C. Christensen, and M. Meldal, J. Org. Chem., 2002, 67, 3057. CrossRef
4. For a selected review on CuAAC, see: L. Liang and D. Astruc, Coord. Chem. Rev., 2011, 255, 2933. CrossRef
5. a) R. Huisgen, Angew. Chem., Int. Ed. Engl., 1963, 2, 565; CrossRef b) A. Brik, J. Muldoon, Y.-C. Lin, J. H. Elder, D. S. Goodsell, A. J. Olson, V. V. Fokin, K. B. Sharpless, and H. Wong, ChemBioChem, 2003, 4, 1246; CrossRef c) A. K. Feldman, B. Colasson, and V. V. Fokin, Org. Lett., 2004, 6, 3897; CrossRef d) M. IJsselstijin and J.-C. Cintat, Tetrahedron, 2006, 62, 3837; CrossRef e) R. K. Reddy, K. Rajgopal, and L. M. Kantam, Synlett, 2006, 6, 957; CrossRef f) S. Diez-Gonzalez, E. D. Stevens, and S. P. Nolan, Chem. Commun., 2008, 4747; CrossRef g) S. Ozcubukcu, E. Ozcal, C. Jimeno, and M. A. Pericas, Org. Lett., 2009, 11, 4680; CrossRef h) S. Diez-Gonzalez, A. Correa, L. Cavallo, and S. P. Nolan, Chem. Eur. J., 2006, 12, 7558; CrossRef i) S. Diez-Gonzalez, E. D. Stevens, and S. P. Nolan, Chem. Commun., 2008, 4747; CrossRef j) S. Diez-Gonzalez and S. P. Nolan, Angew. Chem. Int. Ed., 2008, 47, 8881; CrossRef k) W. Wang, J. Wu, C. Xia, and F. Li, Green Chem., 2011, 13, 3440; CrossRef l) C. Gaulier, A. Hospital, B. Legeret, A. F. Delmas, V. Aucagne, F. Cisnetti, and A. Gautier, Chem. Commun., 2012, 18, 4005; CrossRef m) T. R. Chan, R. Hilgraf, K. B. Sharpless, and V. V. Fokin, Org. Lett., 2004, 6, 2853; CrossRef n) N. Candelon, D. Lastécouères, A. K. Diallo, J. R. Aranzaes, D. Astruc, and J.-M. Vincent, Chem. Commun., 2008, 741; CrossRef o) S. Ozcubukcu, E. Ozcal, C. Jimeno, and M. A. Pericas, Org. Lett., 2009, 11, 4680; CrossRef p) P. Fabbrizzi, S. Cicci, A. Brandi, E. Sperotto, and G. van Koten, Eur. J. Org. Chem., 2009, 5423. CrossRef
6. a) T. Miao and L. Wang, Synthesis, 2008, 3, 363; CrossRef b) T. Shamim and S. Paul, Catal. Lett., 2010, 136, 260;; CrossRef c) P. Veerakumar, M. Velayudham, K.-L. Lu, and S. Rajagopal, Catal. Sci. Technol., 2011, 1, 1512; CrossRef d) M. N. S. Rad, S. Behrouz, M. M. Doroodmand, and A. Movahediyan, Tetrahedron, 2012, 68, 7812; CrossRef e) H. Sharghi, R. Khalifeh, and M. M. Doroodmand, Adv. Synth. Catal., 2009, 351, 207; CrossRef f) F. Alonso, Y. Moglie, G. Radivoy, and M. Yus, Eur. J. Org. Chem., 2010, 1875; g) F. Alonso, Y. Moglie, G. Radivoy, and M. Yus, Org. Biomol. Chem., 2011, 9, 6385; CrossRef h) S. Chassaing, M. Kumarraja, A. S. S. Sido, P. Pale, and J. Sommer, Org. Lett., 2007, 9, 883; CrossRef i) S. Chassaing, A. S. S. Sido, A. Alix, M. Kumarraja, P. Pale, and J. Sommer, Chem. Eur. J., 2008, 14, 6713; CrossRef j) S. Chassaing, A. Alix, T. Boningari, K. S. S. Sido, M. Keller, P. Kuhn, B. Louis, J. Sommer, and P. Pale, Synthesis, 2010, 9, 1557; CrossRef k) A. Alix, S. Chassaing, P. Pale, and J. Sommer, Tetrahedron, 2008, 64, 8922; CrossRef l) V. Beneteau, A. Olmos, T. Boningari, J. Sommer, and P. Pale, Tetrahedron Lett., 2010, 51, 3673; CrossRef m) R. Xiao, R. Yao, and M. Cai, Eur. J. Org. Chem., 2012, 4178; CrossRef n) R. Xiao, W. Hao, J. Ai, and M.-Z. Cai, J. Organomet. Chem., 2012, 705, 44; CrossRef o) R. Hosseinzadeh, H. Sepehrian, and F. Shahrokhi, Heteroatom. Chem., 2012, 23, 415; CrossRef p) R. Xiao, R. Yao, and M. Cai, Eur. J. Org. Chem., 2012, 4178; CrossRef q) C. Girard, E. Onen, M. Aufort, S. Beauviere, E. Samson, and J. Herscovici, Org. Lett., 2006, 8, 1689; CrossRef r) U. Sirion, Y. J. Bae, B. S. Lee, and D. Y. Chi, Synlett, 2008, 15, 2326; CrossRef s) T. Suzuka, K. Ooshiro, and K. Kina, Heterocycles, 2010, 81, 601; CrossRef t) T. Suzuka, Y. Kawahara, K. Ooshiro, T. Nagamine, K. Ogihara, and M. Higa, Heterocycles, 2012, 85, 615; CrossRef u) K. R. Reddy, K. Rajgopal, and M. L. Kantam, Catal. Lett., 2007, 114, 36; CrossRef v) Y. Kitamura, K. Taniguchi, T. Maegawa, Y. Monguchi, Y. Kitade, and H. Sajiki, Heterocycles, 2009, 77, 521; CrossRef w) B. H. Lipshutz and B. R. Taft, Angew. Chem. Int. Ed., 2006, 45, 8235; CrossRef x) C.-T. Lee, S. Huang, and B. H. Lipshutz, Adv. Synth. Catal., 2009, 351, 3139; CrossRef y) K. Namitharan, M. Kumarraja, and K. Pitchumani, Chem. Eur. J., 2009, 15, 2755; CrossRef z) H. Hagiwara, H. Sasaki, T. Hoshi, and T. Suzuki, Synlett, 2009, 4, 643; CrossRef aa) K. Yamaguchi, T. Oishi, T. Katayama, and N. Mizuno, Chem. Eur. J., 2009, 15, 10464; ab) T. Katayama, K. Kamata, K. Yamaguchi, and N. Mizuno, ChemSusChem, 2009, 2, 59.
7. M. Clark and P. Kiser, Polym. Int., 2009, 58, 1190. CrossRef
8. S. W. Kwok, J. R. Fotsing, R. J. Fraser, V. O. Rodionov, and V. V. Fokin, Org. Lett., 2010, 12, 4217. CrossRef
9. I. Luz, F. X. L. i Xamena, and A. Corma, J. Catal., 2010, 276, 134. CrossRef
10. H. Kang, H. J. Lee, J. C. Park, H. Song, and K. H. Park, Top Catal., 2010, 53, 523. CrossRef
11. N. Mukherjee, S. Ahammed, S. Bhadra, and B. C. Ranu, Green Chem., 2013, 15, 389. CrossRef
12. I. Jlalia, F. Meganem, J. Herscovici, and C. Girard, Molecules, 2009, 14, 528. CrossRef
13. Y. Monguchi, K. Nozaki, T. Maejima, Y. Shimoda, Y. Sawama, Y. Kitamura, Y. Kitade, and H. Sajiki, Green Chem., 2013, 15, 490. CrossRef
14. Y. Monguchi, Y. Fujita, K. Endo, S. Takao, M. Yoshimura, Y. Takagi, T. Maegawa, and H. Sajiki, Chem. Eur. J., 2006, 12, 5142. CrossRef
15. Y. Monguchi, K. Sakai, K. Endo, Y. Fujita, M. Niimura, M. Yoshimura, T. Mizusaki, Y. Sawama, and H. Sajiki, ChemCatChem, 2012, 4, 546. CrossRef
16. Triethylamine was required probably due to the formation of an intermediary Cu-acetylide complex as in the case of CuI- or CuBr-catalyzed Huisgen cycloaddition, see M. Meldal and C. W. Tornøe, Chem. Rev., 2008, 108, 2952. CrossRef
17. The leached copper in the filtrate of the reaction mixture was analyzed by inductively coupled plasma atomic emission spectrometry (ICP-AES). Approximately 19% of the copper species was leached out from the 1% Cu/HP20.
18. P. Appukkuttan, W. Dehaen, V. V. Fokin, and E. van der Eycken, Org. Lett., 2004, 6, 4223. CrossRef