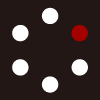
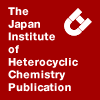
HETEROCYCLES
An International Journal for Reviews and Communications in Heterocyclic ChemistryWeb Edition ISSN: 1881-0942
Published online by The Japan Institute of Heterocyclic Chemistry
e-Journal
Full Text HTML
Received, 1st July, 2013, Accepted, 26th July, 2013, Published online, 7th August, 2013.
DOI: 10.3987/COM-13-S(S)76
■ A Thermal Potential [6+4] 2H-Transfer Reaction in a Cyclic Dimer of Dimethyl 2a,8a-Dihydrocyclopent[c,d]azulene-1,2-dicarboxylate
Peter Uebelhart, Erja Lehto, and Hans-Jürgen Hansen*
Institute of Organic Chemistry, University of Zürich, Winterthurerstrasse 190, CH-8057 Zürich, Switzerland
Abstract
Azulenes and dimethyl acetylenedicarboxylate (ADM) react under catalysis of diethylaluminum chloride (Et2AlCl) in toluene to corresponding 2a,8a-dihydrocyclopenta[cd]azulene-1,2-dicarboxylates, which on further influence of Et2AlCl dimerize by bond formation between the central C(8b) and C(8’b) atoms and inter alia C(3) and C(8’) atoms. The thus formed heptacyclic compounds undergo on heating in tetralin an intramolecular disproportionation by transfer of two H-atoms from one to the other part of the heptacyclic structures. The kinetics of this process is studied with partially deuterated heptacycles.INTRODUCTION
The reaction of azulenes and dimethyl acetylenedicarboxylate (ADM), catalyzed by BrØnsted or Lewis acids, normally takes place prosaically in that mixtures of the corresponding dimethyl 2-(azulen-1-yl)fumarates and -maleates are formed.1,2 However, several years ago, we found that the reaction of azulene (1) itself with ADM in the presence of small amounts of trifluoroacetic acid (TFA) in tetralin with a “breeding” time of 14 d at ambient temperature leads to the formation of a number of further products still with dimethyl 2-(azulen-1-yl)fumarate and maleate (2; (E)-/(Z)-2 = 1.8; Scheme 1) as main components.3 Realizing the structure of 3 and 4 (together with those of two additional products in amounts ≤2% and not shown in Scheme 1), there was little doubt that dimethyl 2a,8a-dihydrocyclopent[c,d]azulene-1,2-dicarboxylate (5) as formal (10 + 2) cycloadduct is a second important product of the acid catalyzed reaction of 1 with ADM, which by prototropic shift yields dimethyl 3,4-dihydrocyclopenta[cd]azulene-1,2-dicarboxylate (3) and by dimerization via bond formation between the central C(8b) and C(8’b) atoms and the perimeter C(6) and C(8’) atoms the heptacyclic compound 4.4
In the frame of these investigations, we found that diethylaluminum chloride (Et2AlCl) in toluene promotes specifically the formation of heptacyclic compounds of type 4, which all showed C(8b),C(8’b) binding but variations in the binding of the peripheral C-atoms – mainly in dependence of the substitution pattern of the starting azulenes.5,6 Further not foreseeable results, accomplished with the dimer derived from 6-(tert-butyl)azulene and ADM, will be discussed in the following part.
RESULTS AND DICUSSION
6-(tert-Butyl)azulene (6) and ADM in toluene at 0 °C reacted almost spontaneously when a 1.4 M solution of Et2AlCl in hexane was added. The products, formed after a reaction time of 15 min at ambient temperature and separated by chromatography, are displayed in Scheme 2.
The characterization of 7 as 8b–8’b;8–3’ dimer of 7-tBu-5 followed from its spectroscopic data, specifically from its 1H-NMR coupling patterns and by comparison with those of the 7,7’-Me2 analog, for which we had an X-ray crystal structure at hand.6,7 Most interesting was the fact that colorless 7, on heating in tetralin at 170 °C, formed a new pale yellow product 9 (Scheme 3), of the same molecular mass as 7, however, with the appearance of the signals of two CH2 groups with Jgem = 21.7 and 15.9 Hz, respectively, in its 1H-NMR spectrum. Final certainty of the structure of 9 gave its X-ray crystal structure analysis (Figure 1).
Taking into account the directly involved carbon fragments of both molecules, one recognizes that the process 7 → 9 can be regarded as a thermally allowed, suprafacial [4+6] H-transfer reaction between a rigid cis-but-2-ene and a fixed s-trans-butadiene subunit (Scheme 4).
It was, therefore, of interest for us to study the kinetic H/D isotope effect of this intramolecular disproportionation by jump of two H-atoms from one to the other part of the molecule as measure for its concertedness.
The formation of 7 as a result of the dimerization of 7-tBu-5 as intermediate led to the conclusion that the introduction of deuterium could only be realized by the synthesis of correspondingly deuterated 6-tert-butylazulenes 6, whereby the Cs-symmetry of 6 would only allow double [2H] labeling. The azulenes to be strived for were therefore 1,3-[2H2]-, 4,8-[2H2]-, and, finally, 1,3,4,8-[2H4]-6. Key azulene was 4,8-[2H2]-6, which we synthesized in established manner from 4-(tert-butyl)-1-butyl-2,6-[2H2]pyridin-1-ium bromide (prepared by heating of its protio form with D2O (cf.8) at 135 °C over weekend) and sodium cyclopentadienide in DMF. Thus formed 4,8-[2H2]-6 (with a deuterium content of ca. 95%) as well as 6 itself were heated in MeO[2H] at 100 °C in the presence of a drop of MeCOO[2H] to accomplish the quantitative deuteration at C(1) and C(3) of the azulenes.9
The reaction of the deuterated azulenes with ADM in the presence Et2AlCl was performed in the same manner as for 6 and ADM (Scheme 2) and delivered the deuterated dimers 7 (Scheme 5) in yields around 40%. A certain loss of deuterium in the range of about 10% in the case of 1,3-[2H2]- and 1,3,4,8-[2H4]-6 was recognized thereby. Heating of these samples in tetralin at 160 °C led to the dimers 9 with the specific [2H]-labeling pattern shown in Scheme 5.
The position of Hex and Hen at the methylene C-atoms 4’ and 6’ clearly shows that the thermal H-transfer is indeed an intramolecular process, whereby H and D jumps from above of the lower molecular plane to below of the upper molecular plane. Hex and Hen of the two CH2 groups are unequivocally characterized by their specific coupling constants and their chemical shifts (see EXPERIMENTAL).
For a better understanding of the given stereochemical facts of the 2H-transfer reaction of 7, it is necessary to inspect the X-ray crystal structure of its 7,7’-Me2 analog (Figure 2) and compare it with the X-ray structure of 9 (Figure 1). The decisive bond lengths and interatomic distances are listed in Table 1. First of all, one recognizes that the two σ-bonds between the two halves of the molecules are distinctly longer than normal C–C bonds. Moreover, the difference in the central C–C bond lengths of the 7-analog is larger than in 9 where they are more equalized. The average central C–C bond length of the 7-analog amounts to 158.7 pm and that of 9 to 157.8 pm. The thermal 2H-transfer seems therefore be accompanied by a certain degree of strain relief between the two halves of the dimers in addition to the gain in conjugation of the dehydrogenated part of dimer 9 (see bond lengths in Table 1). However, most interesting are the interatomic distances between the H-atoms to be transferred and the corresponding acceptor C-atoms of the 7-analog and the analogous post festum distances of 9. These distances are with 285 and 231 pm markedly different from those in 9, which are with 279 and 289 pm more equalized.
In Table 2 are listed several data of 7 and 9 and their desmethoxycarbonyl forms calculated with AM1. First of all, they show that dimer 9 is indeed the thermodynamically favored form. The calculation of the pure carbon skeleton of 7 and 9 (E = H) indicate furthermore that the main contribution to the larger stability of 9 versus 7 stems indeed from the changes in the carbon skeletons due to the thermal 2H transfer. A comparison of the calculated structure of 7 (7,7’-tBu2, E = H) and 7 (7,7’-Me2, E = H) demonstrates that alkyl substituents at C(7) and C(7’) have almost no influence upon the critical parameters for the 2H-transfer (cf. first column, third and forth line Table 2).
We determined the kinetics of the 7 and its [2H]-labeled forms (Scheme 5) on heating in tetralin (c = 10%) in a thermostated oil bath at 150 ± 1 °C. After 14 min preconditioning time, which also served to minimize the amount molecules 7 with an incomplete deuteration, small samples were drawn in appropriate time intervals, and, after exchange of tetralin by CH2Cl2, subjected to HPLC analyses. The outcome was that the transformation 7 → 9 took place in all for cases by zero-order kinetics. The development of product formation is graphically displayed in Figure 3. It reveals that the rates of non-labeled 7 and of 2a,4,2’a,4’-[2H4]-7 are quite similar with a light tendency of a lower rate for the labeled form and those of 5,8a,5’,8’a-[2H4]- and 2a,4,5,8a,2’a,4’,5’,8’a-[2H8]-7 are almost identical. As a result, one recognizes that the intramolecular transfer H–C(8a) → C(4’) is associated with a primary kinetic H/D-isotope effect of k0/k2 = 1.8, i.e., at that side of dimers 7 with the shorter interatomic distance C(8a)–H…C(4’) (Table 1). The observed kinetic H/D-isotope effect at the other side of dimers 7 between C(2a)–H…C(6’) with the distinctly larger interatomic distance (Table 1) is with k0/k1 = 1.1 small, but just within the limit of confidence. These kinetic findings demonstrate that the imposed structural constraints of dimer 7 and 9 lead, as expected, to a strong asymmetric transition state for the 2H-transfer 7 → 9; nevertheless, the transfer is still concerted.
EXPERIMENTAL
General: See.10,11
Formation of the 8b–8’b;8–3’-dimer 7. – Azulene 6 (282.8 mg, 1.535 mmol) and ADM (327.0 mg, 2.303 mmol) were dissolved in toluene (25 mL). The solution was cooled to 0 °C when a 1.4 M solution of Et2AlCl in hexane (1.2 mL, 1.68 mmol) was added. After 15 min stirring at 0 °C, the reaction was stopped by addition of ice and water. The toluene phase was diluted with CH2Cl2 and the aqueous phase several time extracted with CH2Cl2. The combined organic phases were washed with sat. aqueous NaHCO3, then with brine and dried (Na2SO4). The semi-solid residue, left after evaporation, was dissolved in boiling toluene (10 – 15 mL) whereby a small part was not dissolved. The toluene solution was filtered and cooled. The formed almost colorless crystals were filtered and washed with a small amount of Et2O. Yield of 7 (263.4 mg, 52.6%). Dimer 7 was further purified by dissolution in boiling CHCl3 and filtration over Celite. Preparative TLC (silica gel, Et2O/hexane 1:1) of the toluene mother liquor gave (E)-8 (Rf (Et2O/hexane 0.41)) as brown oil (73.8 mg, 14.7%) and (Z)-8 (Rf (Et2O/hexane 0.31)) as dark red oil (60.6 mg, 12.0%).
Data of 7: Colorless crystals, mp 247.6–248.9 °C (toluene). Rf (Et2O/hexane 3:2) 0.36. UV (CH2Cl2): λmax 291 (4.22), 226sh (4.20); λmin 245 (4.08). 1H-NMR (300 MHz, CDCl3)12: 6.562 (d, 3J(5’,6’) = 12.3, H–C(5’)); 6.381 (d, 3J(4,3) = 5.5, H–C(4)); 6.281 (dd, 3J(3,4) = 5.3, 3J(3,2a) = 2.3, H–C(3)); 6.267 (d, 3J(5,6) = 8.4, H–C(6)); 6.127 (d, 3J(5,6) = 8.6, H–C(5)); 6.066 (br. d, 3J(6’,5’) = 12.2, H–C(6’)); 5.979 (d, 3J(4’,3’) = 3.2, H–C(4’)); 5.714 (d, 3J(8’,8’a) = 8.8, H–C(8’)); 3.783 (t, Σ 3J(3’,4’) + 3J(3’,8) = 7.3, 3J(3’,2’a) ≅ 0, H–C(3’)); 3.690 (dd, 3J(8a,8) = 4.5, 5J(8a,2a) = 3.4, H–C(8a)); 3.690, 3.616, 3.595 (3s, 2:1:1, MeOOC–(1’,2’,1,2)); 3.556 (dd, 3J(8’a,8’) = 8.8, 5J(8’a,2’a) = 3.8, H–C(8’a)); 3.409 (t, Σ 3J(8,8a) + 3J(8,3’) = 8.4, H–C(8)); 3.091(t, Σ 3J(2a,3) + 5J(2a,8a) = 6.2, H–C(2a)); 2.927 (d, 5J(2’a,8’a) = 3.7, 3J(2’a,3’) ≅ 0, H–C(2’a)); 1.220 (s, tBu–C(7)); 1.135 (s, tBu–C(7’)). 13C-NMR (150MHz; CDCl3; CDCl3 at δ 76.95): 166.35 and 166.30 (O=C–C(2’,2)); 164.26 (O=C–C(1’)); 164.02 (O=C–C(1)); 154.29 (C(7)); 152.14 (C(1’)); 150.02 (C(2)); 148.05 (C(7’)); 146.54 (C(1)); 146.13 (C(2’)); 137.64 (C(4)); 136.75 (C(4’a)); 135.25 (C(3)); 132.66 (C(4’)); 129.70 (C(4a)); 127.63 (C(5’)); 126.17 (C(6’)); 120.77 (C(6)); 120.51 (C(5)); 119.35 (C(8’)); 66.28 (C(8’b)); 60.83 (C(8b)); 58.38 (C(2’a)); 54.26 (C(2a)); 52.54 (C(3’)); 51.86 (MeOOC–C(2’,2)); 51.71 (MeOOC–C(1’)); 51.59 (MeOOC–C(1)); 48.97 (C(8’a)); 45.17 (C(8a)); 38.77 (C(8)); 38.54 (Me3C–C(7)); 36.83 (Me3C–C(7’)); 30.68 (Me3C–C(7)); 29.79 (Me3C–C(7’)). EI-MS (C40H44O8; 652.79): 653.4 (9, [M + 1]+); 652.4 (18, [M+]); 620.3 (9, [M – MeOH]+); 327.3 (17, [C20H23O4]+); 326.3 (41, [C20H22O4]+); 325.3 (100, [C20H21O4]+); 324.3 (78, [C20H20O4]+); 296.2 (7); 295.2 (21, [C20H23O4 – MeOH]+); 294.2 (32, [C20H22O4 – MeOH]+); 293.2 (85, [C20H21O4 – MeOH]+).
Formation of dimer 9. Dimer 7 (16.9 mg, 0.026 mmol) was dissolved in tetralin (2.0 mL) and heated at 170 °C. After 1.5 h a new distinct yellow spot was visible on TLC (Rf (silica gel, Et2O/4% MeOH) 0.56). The solvent was removed in HV. Prep. TLC with Et2O/2% MeOH delivered the yellow product, which was recrystallized from hexane/Et2O (9.9 mg, 58.6%). In a second run, 7 (66.5 mg, 1.02 mmol) was heated in tetralin (6 mL) for 3 h at 160 °C. Work up as described gave after two recrystallizations of the TLC product suitable crystals from hexane/Et2O for the X-ray crystal structure analysis (Figure 1).
Data of 9: Yellow prisms, mp 235–238 °C (hexane/Et2O). Rf (Et2O/4% MeOH) 0.56. UV/VIS (CH2Cl2): λmax 292 (4.10), 372 (3.53; very broad, tailing up to 460); λmin 266 (4.02), 327 ( 3.45). 1H-NMR (600 MHz, CDCl3): 6.860 (d, 3J(4,3) = 5.3, H–C(4)); 6.788 (d, 3J(3,4) = 5.2, H–C(3)); 6.241 (d, 3J(6,5) = 8.7, H–C(6)); 6.005 (d, 3J(5,6) = 8.7, H–C(5)); 5.557 (d×q-like, 3J(5’,6’exo) = 7.9, 3J(5’,6’endo) ≈ 4J(5’,4’exo) ≅ 4J(5’,4’endo) = 1.8, H–C(5’)); 5.337 (dd-like, 3J(8’,8’a) = 7.7, 4J(8’,6’exo) = 1.0, H–C(8’)); 4.160 (d, 3J(8,3’) = 3.7, H–C(8)); 3.839, 3.738, 3.710, 3.688 (4s, MeOOC–C(1,2,1’,2’)); 3.750 (m, H–C(8’a)); 3.442 (br. t-like, 3J(2’a,3’) ≅ 5J(2’a,8’a) ≅ 5, H–C(2’a)); 3.166 (d×sept-like, Jgem = 21.7, Hendo–C(6’)); 3.012 (dd, 3J(3’,2’a) = 5.9, 3J(3’,8) = 3.7, H–C(3’)); 2.655 (dd, Jgem = 21.7, 3J(6’exo,5’) = 8.0, Hexo–C(6’)); 2.266 (d×sept-like, Jgem = 15.9, 3J(4’exo,3’) ≈ 3, Hexo–C(4’)); 2.065 (d×q-like, Jgem = 16.2, 3J(4’endo,3’) < 1.5, Hendo–C(4’)); 1.249 (s, tBu–C(7)); 1.034 (s, tBu–C(7’)). EI-MS (C40H44O8; 652.79): 653.2 (5, [M + 1]+); 652.2 (11, [M]+); 620.2 (4, [M – MeOH]+); 327.1 (6, [C20H23O4]+); 326.1 (22, [C20H22O4]+); 325.1 (87, [C20H21O4]+); 324.1 (67, [C20H20O4]+); 295.1 (13, [C20H23O4 – MeOH]+); 294.1 (28, [C20H22O4 – MeOH]+); 293.0 (100, [C20H21O4 – MeOH]+).
Deuterated compouds. – Azulenes. – 1,3-[2H2]azulene 6. Azulene 6 (184 mg, 1.0 mmol) was dissolved in MeO[2H] (1.8 mL) and one drop (ca. 10 mg) MeCOO[2H] added.9 The solution was heated in a sealed thick-wall glass-tube at 100 °C for 6 h. After evaporation of the solvent, the residue was chromatographed with hexane on Alox (basic, act. IV) to yield 1,3-[2H2]-6 (169 mg, 91%).
4,8-[2H2]Azulene 6. – 4-(tert-Butyl)-1-butylpyridin-1-ium bromide (1.00 g, 3.67 mmol)13 was dissolved in D2O (10 mL), a spatula tip of CaCO3 was added, and the mixture was heated at 135 °C over weekend (ca. 66 h),8 which led to >95% H/D exchange at 2,6-position. Without CaCO3, the H/D exchange took place extremely sluggishly (140 °C/40 h, rate of D incorporation 5–7%). The dried deuterated pyridinium salt (940 mg, 3.46 mmol) was added at 20 °C in portions to a solution of sodium cyclopentadienide (6.33 mmol) in DMF (12 mL). The mixture was stirred for 17 h at ambient temperature and then poured into half-conc. brine. The residue of the Et2O extract was chromatographed as described above to yield 4,8-[2H2]-6 (in total 252 mg, 39%).
1,3,4,8-[2H4]Azulene 6. – 4,8-[2H2]-6 (125 mg, 0.671 mmol) was treated as described above with MeO[2H] (1.5 mL) and a drop of MeCOO[2H] (100 °C/9 h). Yield of dried 1,3,4,8-[2H4]-6: 124 mg (98%).
Dimers. – 2a,4,2’a,4’-[2H4]Dimer 7. The reaction was performed with 1,3-[2H2]-6 (169 mg, 0.907 mmol), ADM (193 mg, 1.36 mmol) and 1.4 M Et2AlCl (0.85 ml, 1.2 mmol) in toluene (15 mL) in the described manner. The product was obtained as almost colorless powder (108 mg, 36.4%).
Data of 2a,4,2’a,4’-[2H4]-7: mp 231.4–232.2 °C. 1H-NMR (300 MHz, CDCl3): No signals at 6.379 (ca. 11% H; H–C(4)), 5.979 (ca. 9% H; H–C(4’)), 3.083 (H–C(2a)), and 2.919 (H–C(2’a)). 6.278 (s, H–C(3)); 6.064 (dd, Jgem = 12.3, 4J(6’,8’) = 1.4, H–C(6’)); 3.776 (d, 3J(3’,8) = 3.9, H–C(3’)); 3.693 (d, 3J(8a,8) = 4.5, H–C(8a)); 3.557 (d, 3J(8’,8’a) = 8.8, H–C(8’a)).
Thermal rearrangement: Dimer 2a,4,2’a,4’-[2H4]-7 (50.1 mg, 0.0763 mmol) was heated in tetralin (6 mL) during 6 h at 160 °C. The solvent was distilled off in HV. The residue was purified by prep. TLC (Et2O/0.5% MeOH) to yield dimer 4,2’a,4’,6’-[2H4]-9 (26.9 mg, 53.7%).
Data of 4,2’a,4’,6’-[2H4]-9: mp 150–156 °C (hexane/Et2O). 1H-NMR (300 MHz, CDCl3): 6.860 (weak d, J = 5.3, residual H–C(4)); 6.784 (s, flanked by weak d, J = 5.3, H–C(3)); 6.238 (d, J = 8.7, H–C(6)); 6.005 (d, J = 8.6, H–C(5)); 5.552 (dd, J(5’,6’exo) = 8.0, J(5’,4’en) = 1.6, H–C(5’)); 5.329 (dd, J(8’,8’a) = 7.7, J(8’,6’ex) = 1.0, H–C(8’)); 4.152 (d, J(8,3’) = 3.7, H–C(8)); 3.767 (d, J(8’a,8) = 8.0, H–C(8’a)); 3.442 (H–C(2’a), no signal); 3.166 (Hen–C(6’), no signal); 2.999 (d, J(3’,8) = 3.7, H–C(3’)); 2.635 (d, J(6’ex,5’) = 7.8, Hex–C(6’)); 2.266 (Hex–C(4’), no signal); 2.035 (br. s, Hen–C(4’)).
5,8a,5’,8’a-[2H4]Dimer 7. The reaction was performed with 4,8-[2H2]-6 (103 mg, 0.553 mmol), ADM (117 mg, 0.83 mmol) and 1.4 M Et2AlCl (0.5 ml, 0.7 mmol) in toluene (10 mL) in the described manner. The product was obtained as almost colorless powder (75.6 mg, 41.6%).
Data of 5,8a,5’,8’a-[2H4]-7: mp 241.1–241.9 °C (AcOEt). 1H-NMR (300 MHz, CDCl3): No signals at 3.696 (H–C(8a)) and 3.559 (H–C(8’a)). 6.570 (weak d, J = 12.4, 9%, H–C(5’)); 6.381 (d, J(4,3) = 5.5, (H–C(4)); 6.280 (dd, J(3,4) = 5.5, J(3,2a) = 3.1, H–C(3)); 6.265 (s, H–C(6)); 6.127 (weak d, J = 8.7, 9%, H–C(5)); 6.065 (br. s, H–C(6’)); 5.981 (br. d, J(4’,3’) = 3.2, H–C4’)); 5.710 (slightly split s, 5J(5’,8’) ≈ 1, H–C(8’)); 3.783 (t, J(3’,2’a) ≅ J(3’,4’) = 3.6, H–C(3’)); 3.400 (d, J(8,3’) = 3.9, H–C(8)); 3.088 (d, J(2a,3) = 3.0, HC(2a)); 2.928 (s, H–C(2’a)).
Thermal rearrangement: Dimer 5,8a,5’,8’a-[2H4]-7 (33.8 mg, 0.0515 mmol) was heated in tetralin (3.5 mL) during 9 h at 160 °C. The solvent was distilled off in HV. The residue was purified by prep. TLC (Et2O/0.5% MeOH) to yield dimer 5,4’,5’,8a’-[2H2]-9 (13.3 mg, 39.3%).
Data of 5,4’,5’,8’a-[2H4]-9: mp 228.1–231.7 °C (hexane/Et2O). 1H-NMR (300 MHz, CDCl3): 6.826 (d, J(4,3) = 5.2, H–C(4)); 6.787 (d, J(3,4) = 5.3, H–C(3)); 6.237 (s, flanked by weak d at 6.24, J(6,5) = 8.4, residual H–C(6)); 6.004 (weak d, J(5,6) = 8.7, 13%, residual H–C(5)); 5.557 (residual H–C(5’)); 5.327 (d-like, 4J(8’,6’en) ≈ 1, H–C(8’)); 4.153 (d, J(8,3’) = 3.7, H–C(8)); 3,770 (weak d, J(8’,8’a) ≈ 8, residual H–C(8’a)); 3.436 (br. d-like, H–C(2’a)); 3.155 (ddd, Jgem = 21.8, 5J(6’en,4’ex) = 4.4, 4J(6’en,8’) = 2.2, Hen–C(6’)); 2.994 (ddd, J(3’,4’ex) = 6.2, 3J(3’,8) = 3.7, 3J(3’,2’a) = 1.2, H–C(3’)); 2.650 (br. d, Jgem = 21.8, Hex–C(6’)); 2.238 (br. t-like, 3J(4’ex,3’) ≅ 6, 5J(4’ex,6’en) = 4.4, Hex–C(4’)); 2.060 (weak d×t, Jgem ≈ 16.4, 13%, residual Hen–C(4’)).
2a,4,5,8a,2’a,4’,5’,8’a-[2H8]Dimer 7. The reaction was performed with 1,3,4,8-[2H4]-6 (115.5 mg, 0.613 mmol), ADM (120 mg, 0.847 mmol) and 1.4 M Et2AlCl (0.5 ml, 0.7 mmol) in toluene (10 mL) in the described manner. The product was obtained as almost colorless powder (83.6 mg, 41.3%).
Data of 2a,4,5,8a,2’a,4’,5’,8’a-[2H8]-7: mp: n.d. 1H-NMR (300 MHz, CDCl3): 6.567 (weak d, J(5’,6’) = 12.2, 8%, residual H–C(5’)); 6.382 (weak d, J(4,3) = 5.5, 8%, residual H–C(4)); 6.277 (s, H–C(3)); 6.264 (s, H-C(6)); 6.127 (d, J(5,6) = 8.5, 8%, residual H–C(5)); 6.069 (d, J(6’,8’) = 1.2, H–C(6’)); 5.981 (d, J(4’,3’) = 3.3, 9%, residual H–C(4’)); 5.707 (d, J(8’,6’) = 1.1, H–C(8’)); 3.777(d, J(3’,8) = 3.8, H–C(3’)); 3.558 (d, J(8’a,8’) = 8.9, residual H–C(8’a)); 3.398 (d, J(8,3’) = 3.8, H–C(8)); 3.088 (d, J(2a,3) = 3.1, 7%, residual H–C(2a)); 2.928 (s, 7%, residual H–C(2’a)).
Thermal rearrangement: Dimer 2a,4,5,8a,2’a,4’,5’,8’a-[2H8]-7 (41.2 mg, 0.0623 mmol) was heated in tetralin (4 mL) during 10 h at 160 °C. The solvent was distilled off in HV. The residue was purified by prep. TLC (Et2O/0.5% MeOH) to yield 4,5, 2’a,4’,4’,5’,6’,8’a-[2H8]dimer 9 (16.5 mg, 40.0%).
Data of 4,5,2’a,4’,4’,5’,6’,8’a-[2H8]-9: mp 151.1–152.5 °C (hexane/Et2O). 1H-NMR (300 MHz, CDCl3): 6.855 (weak s, 5%, residual H–C(4)); 6.785 (s, symmetrically flanked by weak d, J(3,4) = 5.3, residual H–C(3)); 6.237 (s, asymmetrically flanked by weak d, J(6,5) ≈ 8.6, residual H–C(6)); 6.005 (weak d, J(5.6) ≈ 8.6, 13%, residual H–C(5)); 5.560 (br. weak s, residual H–C(5’)); 5.329 (d-like, 4J(8’,6’ex) ≈ 1, H–C(8’)); 4.153 ((d, J(8,3’) = 3.7, H–C(8)); 3.770 (weak d, J(8’,8’a) ≈ 8, residual H–C(8’)); 3.420 (weak s, residual H–C(2’a)); 3.16 (Hen–C(6’); signal not recognizable); 2.993 (d, J(3’,8) = 3.7, H–C(3’)); 2.628 (br, s, Hex–C(6’)); 2.25 (very weak signal, residual Hex–C(4’)); 2.05 (br. weak s, 13%, residual Hen–C(4’)).
Kinetics of the thermal transformation of dimers 7 into dimers 9. – Samples of dimers 7 (each 5.5 mg) were dissolved in tetralin (0.55 mL) in thin-wall glass-tubes and heated in an oil bath at 150 ± 1 °C. In decent time intervals, 4 drops of the solutions were transferred into preparation glasses. Tetralin was removed at 40 °C under HV in a drying pistol and exchanged by CH2Cl2. The samples were then analyzed by HPLC on Spherisorb Si (5 µm) with (CH2Cl2 + 0.5% MeOH)/hexane 7:3. The results are displayed in Figure 3.
X-Ray crystal structure determination of the 7,7’-Me2 analog of 7. Crystal data: Crystals were
obtained from CH2Cl2/Et2O, C34H32O8, M = 568.62, triclinic, space group P, a = 8.916(3) Å, b = 9.445(2) Å, c = 9.732(2) Å, α = 101.99(2)°, β = 103.26(2)°, γ = 92.02(2)°, V = 714.6(3) Å3, Z = 1, Dx = 1.321 g cm3, 2θ(max)= 60°, T = 173K, crystal dimensions: 0.28 × 0.38 × 0.43 mm, Rigaku AFC5R diffractometer, graphite-monochromated Mo Kα radiation, λ = 0.71073 Å, µ(MoKα) = 0.0937 mm–1, 4407 measured reflexions, 4171 symmetry independent reflexions, 2927 reflexions with I < 2σ(I).
The structure was solved by direct methods using SHELXS86,14 which revealed the positions of all non-hydrogen atoms. The molecule lies across a crystallographic center of inversion, although the molecule itself is acentric. Thus the entire molecule is disordered, the two orientations being related by a centre of inversion. The model fully defines one orientation of the complete molecule, but with site occupation factors of 0.5 for all atoms. There are two difficulties associated with this procedure. Firstly, the number of refined parameters is twice the normal number for a unit cell of this volume, so that the ratio of the number of observed reflections to refined parameters is quite low. Secondly, since the molecule itself is quite close to having its own centre of inversion and the positions of many of the atoms are very close to those of other atoms in the second orientation of the molecule. This causes the refinement matrix to be nearly singular and results in a strong correlation between many of the parameters. Subsequently the estimated standard deviations of the atomic parameters and the derived bond lengths and angles are much larger than usual. All of the H-atoms were placed in geometrically calculated positions.
CCDC 946940 contains the supplementary crystallographic data for this compound. These data can be obtained free of charge from The Cambridge Crystallographic Data Centre via www.ccdc.cam.ac.uk/data_request/cif.
X-Ray crystal structure determination of dimer 9. Crystal data: Crystals were obtained from
hexane/Et2O, C40H44O8, M = 692.80 (652.79 + solvent), triclinic, space group P, a = 11.210(3) Å, b = 15.151(4) Å, c = 10.942(3) Å, α = 96.49(2)°, β = 102.81(2)°, γ = 93.39(2)°, V = 1793.7(8) Å3, Z = 2, Dcalc = 1.283 g cm3, 2θ(max)= 60°, T = –100 ± 1 °C, crystal dimensions: 0.20 × 0.30 × 0.38 mm, Rigaku AFC5R diffractometer, graphite-monochromated MoKα radiation, λ = 0.71069 Å, µ(MoKα) = 0.836 cm–1, 10966 measured reflexions, 10474 were unique (Rint = 0.020) and equivalent reflexions were merged.
The structure was solved by direct methods using SHELXS86,14 which revealed the positions of all non-hydrogen atoms. The ester groups attached to C(1) and C(21) are disordered. For these ester groups, two positions for the methoxy and carbonyl atoms were refined successfully. Evidence was found for the presence of solvent in the crystal lattice, but it remains unclear exactly what the solvent composition is. Atoms were assigned arbitrarily to several peaks of electron density in order to compensate for the electron density in the region occupied by the solvent. The non-hydrogen atoms were refined anisotropically, except for the atoms assigned to the solvent molecule. These latter atoms were refined only isotropically. All of the hydrogen atoms, except those bonded to the disordered ester groups, were placed in the positions indicated by a difference electron density map and their positions were allowed to refine together with individual isotropic temperature factors. The disordered H-atoms were placed in geometrically calculated positions with a C-H distance of 0.95 Å, their positions were not refined and they were assigned fixed isotropic temperature factors with a value of 1.2Beq of the atom to which each was bonded. The final cycle of full-matrix least squares refinement was based on 5162 observed reflections [I > 3σ(I)] and 660 variable parameters, and converged (largest parameter shift was 3.1 times its e.s.d, the large value being associated with oscillating temperature factors for some of the disordered atoms) with un-weighted and weighted agreement factors.
CCDC 941626 contains the supplementary crystallographic data for this compound. These data can be obtained free of charge from The Cambridge Crystallographic Data Centre via www.ccdc.cam.ac.uk/data_request/cif.
ACKNOWLEDGEMENTS
We thank Dr. A. Linden for the X-ray crystal structures of the 7,7’-Me2 analog of dimer 7 as well as of dimer 9 and our NMR and MS laboratories for numerous measurements. Support of this work by the Swiss National Science Foundation is gratefully acknowledged.
References
1. W. Treibs, Naturwiss., 1965, 52, 452.
2. K. Hafner, H. Diehl, and H. U. Süss, Angew. Chem., 1976, 15, 104. CrossRef
3. P. Brügger, P. Uebelhart, R. W. Kunz, R. Siegrist, and H.-J. Hansen, Helv. Chim. Acta, 1998, 81, 2201. CrossRef
4. The systematic IUPAC names, generated with ACD/Name (ACD/Labs, Toronto), of the discussed dimers, are the following:
dimer 4: tetramethyl (2aR*,8aR*,10aS*,11R*,12R*,14aS*)-2a,8a,10a,11,12,14a-hexahydro-12,5-(metheno)-11,6-(prop[1]en[1]yl[3]ylidene)cycloocta[1,2-c:4,3-c']dipentalene-1,2,9,10-tetracarboxylate;
dimer 7: tetramethyl (3aR*,5aS*,6R*,7S*,9aR*)-11,16-di-tert-butyl-3a,5a,6,7,7a,9a-hexahydro-7,14-(metheno)-6,1-(prop[1]en[1]yl[3]ylidene)cyclohepta-[j]cyclopenta[l]-as-indacene-4,5,8,9-tetracarboxylate;
dimer 9: tetramethyl (6R*,7R*,7aR*,9aS*)-11,16-di-tert-butyl-tetrahydro-6H-7,14-methano-6,1-(prop[1]en[1]yl[3]ylidene)cycohepta[j]cyclopenta[l]-as-inda-cene-4,5,8,9-tetracarboxylate
In the present paper, we name dimers of type 4 by the bonds, which link the two 2a,8a-dihydrocyclopenta[cd]azulene-1,2-dicarboxylate units 5. In this way, 4 becomes the 8b–8’b;6–8’ dimer and 7 the 8b–8’b;8–3’ dimer.
5. Y. Chen and H.-J. Hansen, unpubl. results; Y. Chen, Ph.D. thesis, Univ. of Zürich, 1993.
6. All attempts to get good crystals of 7, suitable for an X-ray crystal structure analysis, failed in our hands.
7. We found the 7,7’-Me2 analog of 7 as by-product (0.4%) in the product mixture of the Et2AlCl catalyzed reaction of 6-methylazulene and ADM. It gave suitable crystals from CH2Cl2/Et2O (mp 212.6–213.8 °C) for the X-ray crystal structure analysis.
8. Y. Kawazoe, M. Ohnishi, and Y. Yoshioka, Chem. Pharm. Bull., 1964, 12, 1384.
9. C. Weiss, W. Engewald, and H. Müller, Tetrahedron, 1966, 25, 825. CrossRef
10. S. El Rayes, A. Linden, K. Abou-Hadeed, and H.-J. Hansen, Heterocycles, 2011, 82, 1195. CrossRef
11. V. Lellek and H.-J. Hansen, Heterocycles, 2012, 84, 657. CrossRef
12. All 1H-NMR data are adjusted to CHCl3 at δ 7.260.
13. G. V. Boyd, A. W. Ellis, and M. D. Harms, J. Chem. Soc., Chem. Commun., 1970, 800.
14. G. M. Sheldrick, SHELXS-86, Acta Crystallogr., Sect. A, 1990, 46, 467. CrossRef