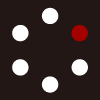
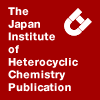
HETEROCYCLES
An International Journal for Reviews and Communications in Heterocyclic ChemistryWeb Edition ISSN: 1881-0942
Published online by The Japan Institute of Heterocyclic Chemistry
e-Journal
Full Text HTML
Received, 29th June, 2013, Accepted, 31st July, 2013, Published online, 8th August, 2013.
DOI: 10.3987/COM-13-S(S)68
■ The Paecilin Puzzle ‒ Enantioselective Synthesis of the Proposed Structures of Paecilin A and B
Lutz F. Tietze,* Ling Ma, Stefan Jackenkroll, Johannes R. Reiner, Judith Hierold, Boopathy Gnanaprakasam, and Sven Heidemann
Institute of Organic and Biomolecular Chemistry, University of Göttingen, Tammannstr. 2, D-37077 Göttingen, Germany
Abstract
For the synthesis of the two diastereomers 3c and 3d of the proposed structure of paecilin B (3) phenol 19, containing an alkenyl moiety, was treated with Pd(II) in the presence of the chiral BOXAX ligand 9b to give 20 with 96% ee. A subsequent Sharpless dihydroxylation afforded two isomeric diols, which were further transformed into 31 and 32. The final steps included removal of the silyl protecting group with simultaneous lactone formation, oxidation and cleavage of the methyl ether. For the preparation of the dimeric paecilin A (1) brominated intermediate 38 was treated with (Bpin)2, S-Phos and Pd(OAc)2. The spectroscopic data of the new compounds did not match those of the isolated natural products.INTRODUCTION
The secondary metabolites paecilin A (1) and B (3) of the endophytic fungus Paecilomyces sp. have been isolated in 2007 by Guo et al.; however, the authors only published the constitution of these natural products, the absolute and relative configurations are still unknown.1 Both paecilins have a chromanone skeleton and contain quaternary stereogenic centers as well as γ-lactone moieties. Paecilin A (1) is likely a dimer of paecilin B (3) with a 8,8’-biaryl connection. In an initial bioassay, paecilin A (1) and B (3) were tested using KB cell lines, but showed no pronounced cytotoxicity. However, several structurally related chromanones substituted with a γ-lactone moiety exhibit promising biological activities such as the dimer gonytolide A (2), which promotes the innate immune response.2 Therefore, it seems reasonable to assume that 1 and 3 may also have some so far unknown bioactivities.
Paecilin B (3) contains three stereogenic centers, therefore it could exist in eight different stereoisomeric forms, the diastereomers 3a-d and their enantiomers ent-3a-d (Figure 2). Though the absolute configuration of 3 is not known, a comparison with the natural products gonytolide A (2),2 diversonol (4)4 as well as blennolide A (5a) and blennolide B (5b)3 would make it feasible to assume that paecilin B should have the relative and absolute configuration as depicted in 3a-d. Recently, Porco et al. described a very nice synthesis of the two racemic diastereomers 3a and 3b; however, their spectroscopic data did not match those published for 3.3c They therefore concluded that the natural product must be 3c, 3d or their respective enantiomers.
Recently, we have described the total syntheses of the tetrahydroxanthenones (–)-diversonol (ent-4)4c and (–)-blennolide A (ent-5a).3b For the synthesis of (–)-diversonol (ent-4) we employed an enantioselective domino Wacker/carbonylation/methoxylation reaction using BOXAX ligand 9a to furnish ester 7 in 96% ee and 80% yield (Scheme 1).4c,5
Reduction of 7 to form aldehyde 8 set the stage for the direct introduction of the hydroxyl group at C-4 (numbering as in 4) using a hydroxylating Knoevenagel condensation.6 To our surprise, however, the steric integrity of the stereogenic center in 8 was lost in the course of the reaction to yield the racemic esters rac-11 and rac-12. Most likely, a retro-1,6-Michael addition led to an opening of the chroman ring system and subsequent racemization via the intermediates 13-16. We therefore sought alternative ways for the hydroxylation. In a revised strategy we introduced the C-4 hydroxy group via a Sharpless dihydroxylation7 of readily accessible vinyl chroman 17, which led to ent-4 after 10 additional steps.
In a related approach we synthesized (–)-blennolide A (ent-5a) via an enantioselective domino Wacker/carbonylation/methoxylation reaction with 96% ee as described before and an enantioselective Wacker oxidation of the diastereomeric alkenyl phenols 19 (E/Z = 1:1.7) to give vinyl chroman 20 using catalytic amounts of palladium(II) trifluoracetate [Pd(OTFA)2] and the chiral (S,S)-Bn-BOXAX ligand 9a in methanol with however only 85% ee and 82% yield (Scheme 2).3b
Herein we report the syntheses of two diastereomers of the proposed structures of paecilin B (3) in their enantiomeric forms ent-3c and ent-3d and one diastereomer of the dimer paecilin A (1) using an enantioselective Wacker oxidation to finally solve the absolute and relative configuration of paecilin A (1) and paecilin B (3) and moreover to provide enough material for further biological studies.
RESULTS AND DISCUSSION
In accordance with the syntheses of ent-4 and ent-5a, retrosynthetic analysis of the dimer paecilin A (1) leads to the methylated monomeric paecilin B (22) via halogenated intermediate 21, from which 1 could be obtained via a Suzuki type coupling. Methylated paecilin B (22) should be accessible from 23 by benzylic oxidation and lactonization (Scheme 3). The latter could arise from intermediate 20, employing a dihydroxylation and Wittig-Horner reaction followed by Michael addition.
Finally, for the synthesis of 20 an enantioselective Wacker oxidation of 19 was envisaged, which in turn can be synthesized from resorcinol (24) in six steps.
As already mentioned, an enantioselective Wacker oxidation of 19 to give 20 has been used by us for the synthesis of (-)-blennolide A (ent-5a)3b using the chiral (S,S)-Bn-BOXAX ligand (9a)8 in methanol with 85% ee and 82% yield. Gratifyingly, the enantioselective Wacker oxidation of 19 could now be improved using (S,S)-iPr-BOXAX (9b) instead of 9a, which resulted in an enantioselectivity of 96% ee and an almost identical yield of 79% (Scheme 4).
Sharpless dihydroxylation of 20 in which the desired hydroxyl groups at C-1’ and C-2’ (numbering as in 20) were set up, was followed by a sequence of double TBS protection, chemoselective removal of the primary silyl group and subsequent oxidation to give the aldehydes 25 and 26, which could be readily separated by column chromatography (Scheme 5). In analogy to the preparation of 32 from 26 via 28 and 30, with ester 32 being an intermediate in the enantioselective synthesis of (–)-blennolide A (ent-5a), diastereomer 31 was synthesized from 25 via 27 and 29 by a Wittig-Horner reaction followed by the introduction of a methyl group at C-3 (numbering as in 27).
Thus, the Wittig-Horner reaction of 25 with (MeO)2P(O)CH2CO2Me and sodium hydride in THF led to the corresponding α,β-unsaturated ester quantitatively with a very good selectivity (E/Z = 15.6:1). The E-diastereomer E-27 was then subjected to a Michael addition using CuBr ∙ Me2S, methyl lithium and TMSCl to yield 29a in 94% yield as the exclusive diastereomer. This stereochemical outcome can be rationalized as a result of a Felkin-Anh transition state without any chelating effects.9 The assigned relative configuration of 30 was confirmed by comparison of the NMR data of (–)-blennolide A (ent-5a) with the natural product (+)-blennolide A. In analogy we rationalized the relative configuration of 29a using the same Felkin-Anh transition state model as for 30. Subsequent hydrogenolysis of the benzyl group with palladium on charcoal and oxidation of the resulting primary alcohol using DMP and KOH/I2 in methanol10 led to the methyl ester 31 in 90% over 3 steps via 29b and 29c. Having successfully prepared the diastereomeric chromanes 31 and 32 the stage was set for the lactonization and benzylic oxidation.
Both esters immediately cyclized upon exposure to tetrabutylammonium fluoride (TBAF · 3 H2O) to give the desired lactones 33 and 35 in 86% and 88% yield, respectively (Scheme 6). To adress the benzylic oxidation of 33 and 35, we used potassium permanganate,11 dirhodium-tetrakiscaprolactamate (36)/tert-butylhydroperoxide (tBuOOH)12 and Mn(OAc)3/tBuOOH13 as oxidizing agents. Treatment of lactone 33 with potassium permanganate in an ultrasonic bath afforded chromanone 34 in 68% yield. For the synthesis of 37 from 35 the use of catalytic amounts of dirhodium-tetrakiscaprolactamate (36), NaHCO3 and an excess of tBuOOH gave the highest yield with 63%. Finally, cleavage of the methyl ether moiety in 34 and 37 with BBr3 in dichloromethane at –78 °C led to ent-3c and ent-3d in 84% and 83% yield, respectively. However, as was the case in the work of Porco et al.,3c the NMR data of compounds ent-3c and ent-3d were not in agreement with those reported for the natural product implying that the published structure of 3 is not correct or the natural product contained some impurities. Unfortunately, we were not able to compare our spectroscopic data with the original spectra, since Guo et al. did not reply to our request.
Significant differences in the 1H- and 13C-NMR spectra were found for 3-Hb, 2’-H, 4’-Ha and 4’-Hb with δ = 3.46 ppm (d, J = 17.1 Hz), 4.34 ppm (d, J = 3.6 Hz), 2.20 ppm (dd, J = 17.7, 3.9 Hz) and 2.99 ppm (dd, J = 17.7, 9.6 Hz) for 3c and δ = 3.17 ppm (d, J = 17.1 Hz), 4.42 ppm (d, J = 3.9 Hz), 2.20 ppm (dd, J = 17.1, 3.9 Hz) and 2.87 ppm (dd, J = 17.1, 9.3 Hz) for 3d. The corresponding signals of the natural product are δ = 3.53 ppm (d, J = 17.4 Hz), 4.97 ppm (d, J = 6.6 Hz), 2.41 ppm (dd, J = 17, 7 Hz) and 2.73 ppm (dd, J = 17, 7 Hz).
For the synthesis of the dimeric paecilin A (1) (Scheme 7) we have chosen a one pot borylation/Suzuki-Miyaura reaction.14 In order to access the desired coupling partners, we halogenated diverse chromanes and chromanones and subjected them to Suzuki conditions. A bromination of 34 and 37 (= 22 without stereochemistry) to give 21 was not suitable, however bromination of 31 at C-8 with tetrabutylammonium tribromide (TBABr3) provided 38 in 91% yield with high selectivity.15 After considerable optimization, we were pleased to observe that reaction of 38 with 10 mol% Pd(OAc)2, 25 mol% S-Phos, (Bpin)2 and Cs2CO3 at 50 °C for 21 h led to the desired dimer 39 in 32% yield. In addition, 62% of the debrominated compound 31 and some starting material 38 were isolated. Desilylation of 39 using triethylamine trihydrofluoride (NEt3 · 3 HF) and cleavage of the methyl ether moieties with BBr3 gave 41 in 66% yield over 2 steps. The brominated diastereomer 42, which was obtained from 32 in 83% yield using TBABr3, did not dimerize to 43 under various reaction conditions. This illustrates that the dimerization is a very sensitive transformation and even slight stereochemical variations impede the reaction. Comparison of the spectroscopic data of 41 again failed to match those published for paecelin A (1).
CONCLUSION
For the structural determination of paecilin A (1) and B (3) we prepared the almost enantiopure homo dimer 41 and two diastereomeric monomers ent-3c and ent-3d. Their spectroscopic data however did not match the published information. The closest fit exists for 3b, prepared by Porco et al.,3c in which only the signal for 3-Hb differs significantly with δ = 3.25 ppm (d, J = 17.3 Hz) instead of δ = 3.53 ppm (d, J = 17.3 Hz).
EXPERIMENTAL
Synthesis of the vinylchroman 20
(S)-2-(Benzyloxymethyl)-5-methoxy-2-vinylchroman (20): A solution of Pd(OTFA)2 (121 mg, 365 µmol, 10 mol%) and (S,S)-iPr-BOXAX (9b) (174 mg, 365 µmol, 10 mol%) in MeOH (3.6 mL) was stirred at rt for 30 min. After addition of a solution of phenol E/Z-19 (E/Z = 1:1.7, 1.14 g, 3.65 mmol, 1.00 eq.) in MeOH (5.4 mL) and p-benzoquinone (1.58 g, 14.6 mmol, 4.00 eq.) the mixture was heated at 60 °C for 24 h and then cooled to rt. Filtration over a pad of silica gel (15 × 6 cm, washing with petroleum ether/EtOAc = 10:1, TLC monitoring), evaporation of the solvent in vacuo and column chromatography on silica gel (petroleum ether/EtOAc = 30:1) provided vinylchroman 20 as a colorless oil (900 mg, 2.90 mmol, 79%, 96% ee). Analytical HPLC (column: Daicel Chiralcel® OD): wavelength: 205 nm, flow: 0.8 mL/min, eluent: n-hexane/i-PrOH = 99:1; tR = 12.0 min, (–)-(S)-20, 98.0%; tR = 17.0 min, (+)-(R)-20, 2.0%; 96% ee, α = 1.83. Optical Rotation: [α]D23 –75.2 (c 0.19, CHCl3). TLC: Rf = 0.35 (petroleum ether/EtOAc = 20:1). 1H-NMR (300 MHz, CDCl3): δ = 1.87–2.08 (m, 2 H, 3-H2), 2.44 (ddd, J = 17.1, 10.8, 6.6 Hz, 1 H, 4-Ha), 2.73 (ddd, J = 17.1, 4.8, 3.9 Hz, 1 H, 4-Hb), 3.53 (d, J = 16.5 Hz, 1 H, CHaOBn), 3.57 (d, J = 16.5 Hz, 1 H, CHbOBn), 3.79 (s, 3 H, 5-OCH3), 4.59 (d, J = 12.3 Hz, 1 H, OCHaPh), 4.63 (d, J = 12.3 Hz, 1 H, OCHbPh), 5.17 (dd, J = 10.8, 1.5 Hz, 1 H, 2'-Ha), 5.25 (dd, J = 17.4, 1.5 Hz, 1 H, 2'-Hb), 5.85 (dd, J = 17.4, 10.8 Hz, 1 H, 1'-H), 6.40 (d, J = 8.1 Hz, 1 H, 6-H), 6.58 (d, J = 8.1 Hz, 1 H, 8-H), 7.06 (t, J = 8.1 Hz, 1 H, 7-H), 7.23–7.38 (m, 5 H, 5 × Ph-H) ppm. 13C-NMR (125 MHz, CDCl3): δ = 16.4 (C-4), 26.6 (C-3), 55.5 (5-OCH3), 73.7 (OCH2Ph), 75.6 (CH2OBn), 78.8 (C-2), 101.6 (C-6), 109.8 (C-8), 110.8 (C-4a), 116.2 (C-2'), 126.9 (C-7), 127.5 (Ph-Cp), 127.6 (Ph-Co), 128.3 (Ph-Cm), 137.8 (C-1'), 138.3 (Ph-Ci), 154.4 (C-8a), 157.5 (C-5) ppm. IR (film): ν (cm-1) = 2934, 2856, 1592, 1468, 1409, 1345, 1315, 1267, 1250, 1193, 1167, 1096, 1028, 929, 773, 738, 698. UV (CH3OH): λmax (lg ε) = 204.0 nm (4.701), 271.5 (3.113), 279.0 (3.121). MS (ESI): m/z (%) = 643.3 (53) [2M+Na]+, 333.2 (100) [M+Na]+, 311.2 (25) [M+H]+. Calcd for C20H22O3: 311.1642 [M+H]+, Found: 311.1641 [M+H]+ (ESI-HRMS).
Syntheses of the lactonyl chromanones ent-3c and ent-3d
Methyl (S)-5-Methoxy-2-[(2S,3S)-3-methyl-5-oxotetrahydrofuran-2-yl]chroman-2carboxylate (33): A solution of chroman 31 (318 mg, 681 µmol, 1.00 eq.) in THF (9.5 mL) was treated with TBAF·3 H2O (430 mg, 1.36 mmol, 2.00 eq.) at rt and the reaction mixture was stirred at rt for 1 h. After addition of silica gel (1.5 g) the solvent was evaporated in vacuo. Column chromatography on silica gel (petroleum ether/EtOAc = 4:1) furnished chroman 33 as a colorless foam (187 mg, 584 µmol, 86%). TLC: Rf = 0.22 (petroleum ether/EtOAc = 4:1). 1H-NMR (300 MHz, CDCl3): δ = 1.07 (d, J = 7.2 Hz, 3 H, 3'-CH3), 2.11 (dd, J = 17.7, 3.6 Hz, 1 H, 4'-Ha), 2.19–2.34 (m, 3 H, 3-H2, 4-Ha), 2.66–2.79 (mc, 1 H, 3'-H), 2.81–2.93 (m, 1 H, 4-Hb), 3.02 (dd, J = 17.7, 9.3 Hz, 1 H, 4'-Hb), 3.72 (s, 3 H, COOCH3), 3.77 (s, 3 H, 5-OCH3), 4.44 (d, J = 3.0 Hz, 1 H, 2'-H), 6.41 (dd, J = 8.4, 0.9 Hz, 1 H, 6-H), 6.49 (dd, J = 8.4, 0.9 Hz, 1 H, 8-H), 7.05 (t, J = 8.4 Hz, 1 H, 7-H) ppm. 13C-NMR (125 MHz, CDCl3): δ = 16.5 (C-4), 20.8 (3'-CH3), 25.5 (C-3), 30.3 (C-3'), 36.4 (C-4'), 52.8 (COOCH3), 55.4 (5-OCH3), 82.1 (C-2), 87.7 (C-2'), 102.6 (C-6), 109.4 (C-8), 109.8 (C-4a), 127.3 (C-7), 153.8 (C-8a), 157.4 (C-5), 171.0 (COOCH3), 176.5 (C-5') ppm. IR (neat): ν (cm-1) = 2949, 1777, 1757, 1731, 1605, 1591, 1467, 1449, 1440, 1344, 1284, 1272, 1247, 1171, 1131, 1086, 1018, 965, 773. UV (CH3OH): λmax (lg ε) = 272.0 nm (3.132), 279.0 (3.127). MS (ESI): m/z (%) = 663.3 (100) [2M+Na]+, 343.1 (26) [M+Na]+. Calcd for C17H20O6: 343.1158 [M+Na]+, Found: 343.1152 [M+Na]+ (ESI-HRMS).
Methyl (S)-5-Methoxy-2-[(2R,3R)-3-methyl-5-oxotetrahydrofuran-2-yl]chroman-2carboxylate (35): A solution of chroman 32 (900 mg, 1.93 mmol, 1.00 eq.) in THF (27 mL) was treated with TBAF·3 H2O (1.22 g, 3.86 mmol, 2.00 eq.) at rt and the reaction mixture was stirred at rt for 100 min. After addition of silica gel (3.9 g) the solvent was evaporated in vacuo. Column chromatography on silica gel (petroleum ether/EtOAc = 4:1 → 3:1) furnished chroman 35 as a colorless foam (542 mg, 1.69 mmol, 88%). TLC: Rf = 0.19 (petroleum ether/EtOAc = 4:1). 1H-NMR (300 MHz, CDCl3): δ = 1.23 (d, J = 7.2 Hz, 3 H, 3'-CH3), 1.76–1.90 (m, 1 H, 3-Ha), 2.12 (dd, J = 17.7, 3.9 Hz, 1 H, 4'-Ha), 2.22–2.41 (m, 2 H, 3-Hb, 4-Ha), 2.61–2.76 (mc, 1 H, 3'-H), 2.81–2.92 (m, 1 H, 4-Hb), 2.90 (dd, J = 17.7, 9.3 Hz, 1 H, 4'-Hb), 3.70 (s, 3 H, COOCH3), 3.77 (s, 3 H, 5-OCH3), 4.45 (d, J = 3.3 Hz, 1 H, 2'-H), 6.42 (dd, J = 8.1, 0.6 Hz, 1 H, 6-H), 6.54 (dd, J = 8.1, 0.6 Hz, 1 H, 8-H), 7.07 (t, J = 8.1 Hz, 1 H, 7-H) ppm. 13C-NMR (125 MHz, CDCl3): δ = 16.2 (C-4), 21.2 (3'-CH3), 25.0 (C-3), 29.7 (C-3'), 36.4 (C-4'), 52.9 (COOCH3), 55.4 (5-OCH3), 81.8 (C-2), 89.0 (C-2'), 102.6 (C-6), 109.4 (C-8), 109.5 (C-4a), 127.5 (C-7), 153.6 (C-8a), 157.4 (C-5), 170.8 (COOCH3), 176.1 (C-5') ppm. IR (neat): ν (cm-1) = 2956, 1772, 1751, 1733, 1606, 1591, 1470, 1347, 1268, 1248, 1170, 1145, 1082, 972, 769, 710, 514. UV (CH3CN): λmax (lg ε) = 203.0 nm (4.630), 273.0 (3.150), 279.0 (3.148), 300.0 (2.433). MS (ESI): m/z (%) = 663.3 (100) [2M+Na]+, 343.1 (34) [M+Na]+. Calcd for C17H20O6: 343.1158 [M+Na]+, Found: 343.1155 [M+Na]+ (ESI-HRMS).
Methyl (S)-5-Methoxy-2-[(2S,3S)-3-methyl-5-oxotetrahydrofuran-2-yl]-4-oxochroman-2carboxylate (34): Method A (Mn-catalysed oxidation): A solution of chroman 33 (40.0 mg, 125 µmol, 1.00 eq.) and tert-butyl hydroperoxide (230 µL of a 5.5 m solution in decane, 1.25 mmol, 10.0 eq.) in EtOAc (0.45 mL) was treated with powdered molecular sieves 3 Å (45 mg) and the resulting mixture was stirred at rt for 30 min. After addition of Mn(OAc)3∙2 H2O (6.70 mg, 25.0 µmol, 20 mol%) stirring was continued for 2 d before additional Mn(OAc)3∙2 H2O (3.40 mg, 12.7 µmol, 10 mol%), tert-butyl hydroperoxide (115 µL of a 5.5 m solution in decane, 633 µmol, 5.06 eq.) and EtOAc (0.2 mL) were added. The mixture was stirred for further 24 h at rt and filtered over silica gel (eluting with EtOAc). After concentration in vacuo and column chromatography on silica gel (petroleum ether/EtOAc = 3:2) chromanone 34 was obtained as a colorless solid (29.3 mg, 87.6 µmol, 70%). Method B (Rh-catalysed oxidation): A solution of chroman 33 (40.0 mg, 125 µmol, 1.00 eq.) and dirhodium-tetrakiscaprolactamate (36) (410 µg, 625 nmol, 0.5 mol%) in dichloroethane (0.5 mL) was treated with NaHCO3 (5.30 mg, 62.5 µmol, 0.50 eq.). tert-Butyl hydroperoxide (114 µL of a 5.5 m solution in decane, 625 µmol, 5.00 eq.) was added and the resulting deep-red solution was heated with stirring at 40 °C. After 3 h the mixture was treated with additional dirhodium-tetrakiscaprolactamate (36) (410 µg, 625 nmol, 0.5 mol%) and tert-butyl hydroperoxide (114 µL of a 5.5 m solution in decane, 625 µmol, 5.00 eq.). Stirring was continued at 40 °C for 19 h before additional dirhodium-tetrakiscaprolactamate (36) (820 µg, 1.25 µmol, 1 mol%) and tert-butyl hydroperoxide (228 µL of a 5.5 m solution in decane, 1.25 mmol, 10.0 eq.) were added. After stirring at 40 °C for further 8 h the solids were removed by filtration over silica gel (eluting with EtOAc). After evaporation of the solvent in vacuo and column chromatography on silica gel (petroleum ether/EtOAc = 3:2) chromanone 34 was obtained as a colorless solid (28.0 mg, 83.7 µmol, 67%). Method C (KMnO4 oxidation): A suspension of chroman 33 (50.0 mg, 156 µmol, 1.00 eq.), potassium permanganate (99.0 mg, 624 µmol, 4.00 eq.), 15% aq. MgSO4 solution (0.25 mL) and acetone (1 mL) in a sealed tube was kept for 4 h at 60 °C in ultrasonic bath. A second portion of potassium permanganate (99.0 mg, 624 µmol, 4.00 eq.), 15% aq. MgSO4 solution (0.25 mL) and acetone (0.5 mL) was added before the irradiation was continued for 3 h at 60 °C. After addition of a third portion of potassium permanganate (150 mg, 949 µmol, 8.00 eq.) and 15% aq. MgSO4 solution (0.38 mL) the reaction mixture was irradiated for further 3 h bei 60 °C, cooled to rt and passed through silica gel in column (6 × 3 cm, washing with EtOAc, TLC monitoring). After evaporation of the solvent in vacuo column chromatography on silica gel (petroleum ether/EtOAc = 3:2) furnished chromanone 34 as a colorless solid (35.5 mg, 106 µmol, 68%, 73% brsm). Optical Rotation: [α]D24 –36.3 (c 0.60, CHCl3). TLC: Rf = 0.24 (petroleum ether/EtOAc = 1:1). 1H-NMR (300 MHz, CDCl3): δ = 1.14 (d, J = 7.2 Hz, 3 H, 3'-CH3), 2.18 (dd, J = 17.7, 4.2 Hz, 1 H, 4'-Ha), 2.71–2.85 (mc, 1 H, 3'-H), 2.93 (d, J = 16.2 Hz, 1 H, 3-Ha), 2.98 (dd, J = 17.7, 9.3 Hz, 1 H, 4'-Hb), 3.35 (d, J = 16.2 Hz, 1 H, 3-Hb), 3.68 (s, 3 H, COOCH3), 3.85 (s, 3 H, 5-OCH3), 4.34 (d, J = 3.3 Hz, 1 H, 2'-H), 6.51 (dd, J = 8.4, 0.9 Hz, 1 H, 6-H), 6.58 (dd, J = 8.4, 0.9 Hz, 1 H, 8-H), 7.38 (t, J = 8.4 Hz, 1 H, 7-H) ppm. 13C-NMR (125 MHz, CDCl3): δ = 20.5 (3'-CH3), 29.6 (C-3'), 36.3 (C-4'), 42.4 (C-3), 53.3 (COOCH3), 56.2 (5-OCH3), 84.1 (C-2), 86.5 (C-2'), 104.7 (C-6), 109.8 (C-8), 110.6 (C-4a), 136.4 (C-7), 160.3 (C-5), 161.0 (C-8a), 169.1 (COOCH3), 175.7 (C-5'), 187.4 (C-4) ppm. IR (neat):ν(cm-1) = 2973, 1779, 1747, 1694, 1601, 1578, 1471, 1441, 1291, 1259, 1199, 1164, 1012, 788, 742, 580, 529. UV (CH3CN): λmax (lg ε) = 214.0 nm (4.187), 265.0 (3.907), 327.0 (3.564). MS (ESI): m/z (%) = 691.2 (100) [2M+Na]+, 357.1 (28) [M+Na]+. Calcd for C17H18O7: 357.0950 [M+Na]+, Found: 357.0946 [M+Na]+ (ESI-HRMS).
Methyl (S)-5-Methoxy-2-[(2R,3R)-3-methyl-5-oxotetrahydrofuran-2-yl]-4-oxochroman-2-carboxylate (37): A solution of chroman 35 (90.0 mg, 281 µmol, 1.00 eq.) and dirhodium-tetrakiscaprolactamate (1.84 mg, 2.81 µmol, 1 mol%) in dichloroethane (1.1 mL) was treated with NaHCO3 (11.8 mg, 141 µmol, 0.50 eq.). tert-Butyl hydroperoxide (510 µL of a 5.5 m solution in decane, 2.81 mmol, 10.0 eq.) was added and the resulting deep-red solution was heated with stirring at 40 °C. After 4 h the mixture was treated with additional dirhodium-tetrakiscaprolactamate (1.84 mg, 2.81 µmol, 1 mol%) and tert-butyl hydroperoxide (510 µL of a 5.5 m solution in decane, 2.81 mmol, 10.0 eq.). Stirring was continued at 40 °C for 15 h before additional dirhodium-tetrakiscaprolactamate (1.84 mg, 2.81 µmol, 1 mol%) and tert-butyl hydroperoxide (510 µL of a 5.5 m solution in decane, 2.81 mmol, 10.0 eq.) were added. After stirring at 40 °C for further 6.5 h the solids were removed by filtration over silica gel (eluting with EtOAc). After evaporation of the solvent in vacuo and column chromatography on silica gel (petroleum ether/EtOAc = 1:1) chromanone 37 was obtained as a colorless solid (59.0 mg, 176 µmol, 63%). Mn-catalysed oxidation (51% yield, 64% brsm) and KMnO4 oxidation (47% yield) were performed according to the described procedure for synthesis of 34. TLC: Rf = 0.21 (petroleum ether/EtOAc = 1:1). 1H-NMR (300 MHz, CDCl3): δ = 1.25 (d, J = 6.9 Hz, 3 H, 3'-CH3), 2.19 (dd, J = 17.1, 3.6 Hz, 1 H, 4'-Ha), 2.74–2.89 (mc, 1 H, 3'-H), 2.88 (dd, J = 17.1, 9.3 Hz, 1 H, 4'-Hb), 2.93 (d, J = 16.2 Hz, 1 H, 3-Ha), 3.06 (d, J = 16.2 Hz, 1 H, 3-Hb), 3.68 (s, 3 H, COOCH3), 3.88 (s, 3 H, 5-OCH3), 4.41 (d, J = 3.6 Hz, 1 H, 2'-H), 6.54 (dd, J = 8.4, 0.9 Hz, 1 H, 6-H), 6.65 (dd, J = 8.4, 0.9 Hz, 1 H, 8-H), 7.41 (t, J = 8.4 Hz, 1 H, 7-H) ppm. 13C-NMR (125 MHz, CDCl3): δ = 21.0 (3'-CH3), 29.8 (C-3'), 36.1 (C-4'), 41.6 (C-3), 53.4 (COOCH3), 56.2 (5-OCH3), 84.1 (C-2), 87.7 (C-2'), 104.9 (C-6), 110.1 (C-8), 110.9 (C-4a), 136.8 (C-7), 160.5 (C-5), 161.2 (C-8a), 168.9 (COOCH3), 175.4 (C-5'), 186.4 (C-4) ppm. IR (neat): ν (cm-1) = 1767, 1755, 1674, 1601, 1574, 1471, 1440, 1336, 1257, 1178, 1100, 1076, 1001, 790, 742, 650, 576, 520. UV (CH3CN): λmax (lg ε) = 265.0 nm (3.995), 328.0 (3.677). MS (ESI): m/z (%) = 691.2 (100) [2M+Na]+, 357.1 (40) [M+Na]+. Calcd for C17H18O7: 357.0950 [M+Na]+, Found: 357.0945 [M+Na]+ (ESI-HRMS).
Methyl (S)-5-Hydroxy-2-[(2S,3S)-3-methyl-5-oxotetrahydrofuran-2-yl]-4-oxochroman-2carboxylate (ent-3c): A solution of BBr3 (7.78 mL of a 1.0 m solution in CH2Cl2, 7.78 mmol, 10.0 eq.) was added slowly to a stirred solution of chromanone 34 (260 mg, 778 µmol, 1.00 eq.) in CH2Cl2 (40 mL) at –78 °C. Stirring was continued for 30 min at –78 °C before being quenched with sat. aq. NaHCO3 solution (7 mL) at –78 °C. The organic layer was separated and the aqueous layer was extracted with EtOAc (3 × 40 mL). The combined organic layers were dried over Na2SO4 and the solvent was evaporated in vacuo. After column chromatography on silica gel (petroleum ether/EtOAc = 2:1) chromanone ent-3c was obtained as a colorless solid (209 mg, 653 µmol, 84%). Optical Rotation: [α]D24 –68.1 (c 1.20, CHCl3). TLC: Rf = 0.31 (petroleum ether/EtOAc = 2:1). 1H-NMR (300 MHz, CDCl3): δ = 1.16 (d, J = 7.2 Hz, 3 H, 3'-CH3), 2.20 (dd, J = 17.7, 3.9 Hz, 1 H, 4'-Ha), 2.76–2.90 (mc, 1 H, 3'-H), 2.99 (dd, J = 17.7, 9.6 Hz, 1 H, 4'-Hb), 3.05 (d, J = 17.1 Hz, 1 H, 3-Ha), 3.46 (d, J = 17.1 Hz, 1 H, 3-Hb), 3.72 (s, 3 H, COOCH3), 4.34 (d, J = 3.6 Hz, 1 H, 2'-H), 6.47 (dd, J = 8.4, 0.9 Hz, 1 H, 8-H), 6.52 (dd, J = 8.4, 0.9 Hz, 1 H, 6-H), 7.37 (t, J = 8.4 Hz, 1 H, 7-H), 11.42 (sbr, 1 H, 5-OH) ppm. 13C-NMR (125 MHz, CDCl3): δ = 20.5 (3'-CH3), 29.6 (C-3'), 36.3 (C-4'), 40.5 (C-3), 53.5 (COOCH3), 84.2 (C-2), 86.4 (C-2'), 107.4 (C-8, C-4a), 110.4 (C-6), 138.8 (C-7), 159.2 (C-8a), 161.8 (C-5), 169.0 (COOCH3), 175.5 (C-5'), 194.8 (C-4) ppm. IR (neat): ν (cm-1) = 2976, 2955, 1779, 1738, 1639, 1623, 1577, 1466, 1342, 1293, 1201, 1179, 1156, 1048, 1008, 840, 802, 728, 637, 579, 515. UV (CH3CN): λmax (lg ε) = 271.0 nm (3.930), 347.0 (3.485). MS (ESI): m/z (%) = 663.2 (100) [2M+Na]+, 343.1 (31) [M+Na]+. Calcd for C16H16O7: 343.0794 [M+Na]+, Found: 343.0796 [M+Na]+ (ESI-HRMS).
Methyl (S)-5-Hydroxy-2-[(2R,3R)-3-methyl-5-oxotetrahydrofuran-2-yl]-4-oxochroman2-carboxylate(ent-3d): A solution of BBr3 (18.5 mL of a 1.0 m solution in CH2Cl2, 18.5 mmol, 10.0 eq.) was added slowly to a stirred solution of chromanone 37 (620 mg, 1.85 mmol, 1.00 eq.) in CH2Cl2 (90 mL) at –78 °C. Stirring was continued for 30 min at –78 °C before being quenched with sat. aq. NaHCO3 solution (170 mL) at –78 °C. The organic layer was separated and the aqueous layer was extracted with EtOAc (3 × 80 mL). The combined organic layers were dried over Na2SO4 and the solvent was evaporated in vacuo. After column chromatography on silica gel (petroleum ether/EtOAc = 2:1) chromanone ent-3d was obtained as a pale-yellow foam (492 mg, 1.54 mmol, 83%). Optical Rotation: [α]D23 –39.1 (c 1.02, CHCl3). TLC: Rf = 0.61 (petroleum ether/EtOAc = 1:1). 1H-NMR (300 MHz, CDCl3): δ = 1.26 (d, J = 6.9 Hz, 3 H, 3'-CH3), 2.20 (dd, J = 17.1, 3.9 Hz, 1 H, 4'-Ha), 2.74–2.89 (mc, 1 H, 3'-H), 2.87 (dd, J = 17.1, 9.3 Hz, 1 H, 4'-Hb), 3.01 (d, J = 17.1 Hz, 1 H, 3-Ha), 3.17 (d, J = 17.1 Hz, 1 H, 3-Hb), 3.70 (s, 3 H, COOCH3), 4.42 (d, J = 3.9 Hz, 1 H, 2'-H), 6.51 (d, J = 8.4 Hz, 1 H, 8-H), 6.54 (d, J = 8.4 Hz, 1 H, 6-H), 7.39 (t, J = 8.4 Hz, 1 H, 7-H), 11.41 (sbr, 1 H, 5-OH) ppm. 13C-NMR (125 MHz, CDCl3): δ = 20.8 (3'-CH3), 29.9 (C-3'), 36.0 (C-4'), 39.7 (C-3), 53.6 (COOCH3), 84.2 (C-2), 87.5 (C-2'), 107.6 (C-4a), 107.6 (C-8), 110.6 (C-6), 139.0 (C-7), 159.1 (C-8a), 161.9 (C-5), 168.8 (COOCH3), 175.1 (C-5'), 193.9 (C-4) ppm. IR (neat):ν(cm-1) = 2957, 1784, 1738, 1646, 1626, 1579, 1461, 1354, 1228, 1202, 1171, 1051, 1008, 796, 731, 638. UV (CH3OH): λmax (lg ε) = 206.0 nm (4.246), 272.0 (3.945), 349.0 (3.471). MS (ESI): m/z (%) = 663.2 (100) [2M+Na]+, 343.1 (20) [M+Na]+. Calcd for C16H16O7: 343.0794 [M+Na]+, Found: 343.0790 [M+Na]+ (ESI-HRMS).
Synthesis of the dimeric compound 41
Methyl (S)-8-Bromo-2-[(1S,2S)-1-(tert-butyldimethylsilyloxy)-4-methoxy-2-methyl-4oxo-butyl]-5- methoxy-4-oxochroman-2-carboxylate (38): nBu4NBr3 (145 mg, 301 µmol, 1.02 eq.) was added to a solution of chromanone 31 (142 mg, 295 µmol, 1.00 eq.) in THF/H2O (1:1, 1.4 mL) at rt and the reaction mixture was stirred for 23 h at rt. After concentration in vacuo and column chromatography on silica gel (petroleum ether/EtOAc = 3:1) chromanone 38 was obtained as a colorless oil (150 mg, 268 µmol, 91%). Optical Rotation: [α]D25 +12.5 (c 1.70, CHCl3). TLC: Rf = 0.31 (petroleum ether/EtOAc = 2:1). 1H-NMR (300 MHz, CDCl3): δ = 0.10 (s, 3 H, Si(CH3)a), 0.15 (s, 3 H, Si(CH3)b), 0.89 (s, 9 H, SiC(CH3)3), 1.11 (d, J = 6.9 Hz, 3 H, 2'-CH3), 2.19 (dd, J = 16.5, 10.5 Hz, 1 H, 3'-Ha), 2.38–2.53 (mc, 1 H, 2'-H), 2.88 (d, J = 16.2 Hz, 1 H, 3-Ha), 3.23 (dd, J = 16.5, 3.0 Hz, 1 H, 3'-Hb), 3.25 (d, J = 16.2 Hz, 1 H, 3-Hb), 3.64 (s, 3 H, 2-COOCH3), 3.65 (s, 3 H, 4'-OCH3), 3.86 (s, 3 H, 5-OCH3), 4.00 (d, J = 2.1 Hz, 1 H, 1'-H), 6.43 (d, J = 9.0 Hz, 1 H, 6-H), 7.60 (d, J = 9.0 Hz, 1 H, 7-H) ppm. 13C-NMR (125 MHz, CDCl3): δ = –4.2, –3.2 (Si(CH3)2), 18.5 (SiC(CH3)3), 19.9 (2'-CH3), 26.1 (SiC(CH3)3), 32.7 (C-2'), 36.6 (C-3'), 43.1 (C-3), 51.5 (4'-OCH3), 53.1 (2-COOCH3), 56.3 (5-OCH3), 78.1 (C-1'), 88.4 (C-2), 102.2 (C-8), 105.4 (C-6), 111.8 (C-4a), 139.1 (C-7), 157.8 (C-8a), 159.4 (C-5), 170.2 (2-COOCH3), 173.6 (C-4'), 188.2 (C-4) ppm. IR (neat):ν(cm-1) = 2953, 2931, 1735, 1639, 1587, 1471, 1436, 1315, 1251, 1099, 1054, 832, 776, 734, 528. UV (CH3CN): λmax (lg ε) = 194.0 nm (4.426), 268.0 (3.813), 336.0 (3.592). MS (ESI): m/z (%) = 1141.3 (100) [2M+Na]+, 1063.4 (9) [2M–Br+Na]+, 583.1 (29) [M+Na]+, 561.2 (48) [M+H]+, 481.3 (6) [M–Br+H]+. Calcd for C24H35BrO8Si: 581.1182 [M+Na]+, Found: 581.1177 [M+Na]+ (ESI-HRMS).
Dimethyl (2S,2'S)-2,2'-bis[(1S,2S)-1-(tert-Butyldimethylsilyloxy)-4-methoxy-2-methyl-4-oxobutyl]- 5,5'-dimethoxy-4,4'-dioxo-[8,8'-bichroman]-2,2'-dicarboxylate (39): A solution of chromanone 38 (127 mg, 227 µmol, 1.00 eq.) in THF (8.5 mL) was added to a mixture of Pd(OAc)2 (5.10 mg, 22.7 µmol, 10 mol%), S-Phos (23.3 mg, 56.8 µmol, 25 mol%), Cs2CO3 (148 mg, 454 µmol, 2.00 eq.), bis(pinacolato)diboron (115 mg, 454 µmol, 2.00 eq.) and water (16.4 µL, 16.4 mg, 908 µmol, 4.00 eq.) at rt and the reaction mixture was stirred at 50 °C for 21 h. The catalyst was removed by filtration over silica gel (washing with EtOAc) and the solvent was evaporated in vacuo. Column chromatography on silica gel (petroleum ether/EtOAc = 2:1 → 1:1) provided biaryl 39 as a colorless solid (35.0 mg, 36.5 µmol, 32%) and a inseparable mixture of chromanonen 31 and 38 as a pale-yellow oil (77.0 mg, 141 µmol, 62%, 31:38 = 1:5). Optical Rotation: [α]D26 –24.6 (c 0.92, CHCl3). TLC: Rf = 0.11 (petroleum ether/EtOAc = 2:1). 1H-NMR (300 MHz, CDCl3): δ = 0.06 (s, 6 H, 2 × Si(CH3)a), 0.09 (s, 6 H, 2 × Si(CH3)b), 0.84–0.96 (m, 24 H, 2 × SiC(CH3)3, 2''-CH3, 2'''-CH3), 1.88 (dd, J = 16.8, 10.8 Hz, 2 H, 3''-Ha, 3'''-Ha), 2.05–2.22 (m, 2 H, 2''-H, 2'''-H), 2.38 (d, J = 16.8 Hz, 2 H, 3''-Hb, 3'''-Hb), 2.87 (dd, J = 15.6 Hz, 2 H, 3-Ha, 3'-Ha), 3.25 (d, J = 15.6 Hz, 2 H, 3-Hb, 3'-Hb), 3.47 (s, 6 H, 4''-OCH3, 4'''-OCH3), 3.63 (sbr, 6 H, 2-COOCH3, 2'-COOCH3), 3.85 (d, J = 1.8 Hz, 2 H, 1''-H, 1'''-H), 3.92 (s, 6 H, 5-OCH3, 5'-OCH3), 6.55 (d, J = 9.0 Hz, 2 H, 6-H, 6'-H), 7.54 (d, J = 9.0 Hz, 2 H, 7-H, 7'-H) ppm. 13C-NMR (125 MHz, CDCl3): δ = –4.4, –3.4 (Si(CH3)2), 18.4 (SiC(CH3)3), 19.1 (2''-CH3, 2'''-CH3), 26.1 (SiC(CH3)3), 32.9 (C-2'', C-2'''), 35.7 (C-3'', C-3'''), 43.3 (C-3, C-3'), 51.1 (4''-OCH3, 4'''-OCH3), 52.9 (2-COOCH3, 2'-COOCH3), 56.0 (5-OCH3, 5'-OCH3), 78.1 (C-1'', C-1'''), 87.6 (C-2, C-2'), 104.0 (C-6, C-6'), 110.8 (C-4a, C-4a'), 118.0 (C-8, C-8'), 139.4 (C-7, C-7'), 158.7 (C-8a, C-8a'), 159.8 (C-5, C-5'), 171.0 (2-COOCH3, 2'-COOCH3), 173.2 (C-4'', C-4'''), 189.1 (C-4, C-4') ppm. IR (neat):ν(cm-1) = 2923, 1728, 1690, 1593, 1572, 1474, 1255, 1166, 1119, 1099, 1039, 827, 772. UV (CH3CN): λmax (lg ε) = 193.0 nm (4.679), 247.0 (4.261), 338.0 (3.818). MS (ESI): m/z (%) = 981.4 (100) [M+Na]+. Calcd for C48H70O16Si2: 981.4100 [M+Na]+, Found: 981.4095 [M+Na]+ (ESI-HRMS).
Dimethyl (2S,2'S)-5,5'-Dimethoxy-2,2'-bis[(2S,3S)-3-methyl-5-oxotetrahydrofuran-2-yl]4,4'-dioxo- [8,8'-bichroman]-2,2'-dicarboxylate (40): NEt3∙3 HF (120 µL, 118 mg, 730 µmol, 25.0 eq.) was added to a solution of biaryl 39 (28.0 mg, 29.2 µmol, 1.00 eq.) in 1,4-dioxane (1 mL) at rt and the reaction mixture was stirred at 60 °C for 3 d. After a second addition of NEt3∙3 HF (120 µL, 118 mg, 730 µmol, 25.0 eq.) stirring was continued for further 4 d at 60 °C before being quenched by carefully addition of sat. aq. NaHCO3 solution (8 mL) at 0 °C. The aqueous layer was extracted with EtOAc (3 × 15 mL), the combined organic layers were dried over Na2SO4 and the solvent was evaporated in vacuo. Column chromatography on silica gel (petroleum ether/EtOAc = 1:5) furnished compound 40 as a colorless foam (19.0 mg, 28.5 µmol, 98%). Optical Rotation: [α]D25 +11.4 (c 0.47, CHCl3). TLC: Rf = 0.12 (petroleum ether/EtOAc = 1:3). 1H-NMR (300 MHz, CDCl3): δ = 1.00 (d, J = 7.2 Hz, 6 H, 3''-CH3, 3'''-CH3), 1.55–1.86 (m, 4 H, 4''-H2, 4'''-H2), 2.47–2.65 (mc, 2 H, 3''-H, 3'''-H), 2.74 (d, J = 15.9 Hz, 2 H, 3-Ha, 3'-Ha), 3.42 (d, J = 15.9 Hz, 2 H, 3-Hb, 3'-Hb), 3.60 (sbr, 6 H, 2-COOCH3, 2'-COOCH3), 3.90 (s, 6 H, 5-OCH3, 5'-OCH3), 4.28 (sbr, 2 H, 2''-H, 2'''-H), 6.61 (d, J = 8.7 Hz, 2 H, 6-H, 6'-H), 7.13–7.29, 7.43–7.64 (2 × m, 2 H, 7-H, 7'-H) ppm. 13C-NMR (125 MHz, CDCl3): δ = 21.0 (3''-CH3, 3'''-CH3), 29.6 (C-3'', C-3'''), 35.3 (C-4'', C-4'''), 42.3 (C-3, C-3'), 52.5 (2-COOCH3, 2'-COOCH3), 56.4 (5-OCH3, 5'-OCH3), 84.2 (C-2, C-2'), 85.9 (C-2'', C-2'''), 104.0 (C-6, C-6'), 110.6 (C-8, C-8'), 118.1 (C-4a, C-4a'), 138.2 (C-7, C-7'), 158.4 (C-8a, C-8a'), 159.6 (C-5, C-5'), 167.9 (2-COOCH3, 2'-COOCH3), 176.9 (C-5'', C-5'''), 188.0 (C-4, C-4') ppm. IR (neat): ν (cm-1) = 2958, 1783, 1748, 1684, 1569, 1474, 1254, 1160, 1091, 1011, 749. UV (CH3CN): λmax (lg ε) = 194.0 nm (4.628), 254.0 (4.251), 336.0 (3.899). MS (ESI): m/z (%) = 1355.4 (71) [2M+Na]+, 689.2 (100) [M+Na]+, 667.2 (60) [2M+H]+. Calcd for C34H34O14: 689.1846 [M+Na]+, Found: 689.1841 [M+Na]+ (ESI-HRMS).
Dimethyl (2S,2'S)-5,5'-Dihydroxy-2,2'-bis[(2S,3S)-3-methyl-5-oxotetrahydrofuran-2-yl]4,4'-dioxo- [8,8'-bichroman]-2,2'-dicarboxylate (41): A solution of BBr3 (590 µL of a 1.0 m solution in CH2Cl2, 590 µmol, 19.9 eq.) was added slowly to a stirred solution of biaryl 40 (19.0 mg, 29.7 µmol, 1.00 eq.) in CH2Cl2 (3 mL) at –78 °C. The resulting solution was stirred for 30 min at –78 °C before being quenched with sat. aq. NaHCO3 solution (10 mL) at –78 °C. The organic layer was separated and the aqueous layer was extracted with EtOAc (3 × 10 mL). The combined organic layers were dried over Na2SO4 and the solvent was evaporated in vacuo. After column chromatography on silica gel (petroleum ether/EtOAc = 1:1) biaryl 41 was obtained as a colorless foam (12.7 mg, 19.9 µmol, 67%). Optical Rotation: [α]D24 +78.3 (c 0.64, CHCl3). TLC: Rf = 0.28 (petroleum ether/EtOAc = 1:1). 1H-NMR (300 MHz, CDCl3): δ = 1.05 (d, J = 6.9 Hz, 6 H, 3''-CH3, 3'''-CH3), 1.82 (dd, J = 18.0, 3.6 Hz, 2 H, 4''-Ha, 4'''-Ha), 2.06 (dd, J = 18.0, 9.0 Hz, 2 H, 4''-Hb, 4'''-Hb), 2.39–2.57 (mc, 2 H, 3''-H, 3'''-H), 3.03 (d, J = 17.4 Hz, 2 H, 3-Ha, 3'-Ha), 3.59 (d, J = 17.4 Hz, 2 H, 3-Hb, 3'-Hb), 3.76 (sbr, 6 H, 2-COOCH3, 2'-COOCH3), 4.26 (d, J = 3.0 Hz, 2 H, 2''-H, 2'''-H), 6.60 (d, J = 8.7 Hz, 2 H, 6-H, 6'-H), 7.47–7.82 (m, 2 H, 7-H, 7'-H), 11.61 (sbr, 2 H, 5-OH, 5'-OH) ppm. 13C-NMR (125 MHz, CDCl3): δ = 20.7 (3''-CH3, 3'''-CH3), 29.7 (C-3'', C-3'''), 35.4 (C-4'', C-4'''), 40.5 (C-3, C-3'), 53.5 (2-COOCH3, 2'-COOCH3), 84.9 (C-2, C-2'), 85.9 (C-2'', C-2'''), 107.5 (C-4a, C-4a'), 109.9 (C-6, C-6'), 115.0 (C-8, C-8'), 140.8 (C-7, C-7'), 156.4 (C-8a, C-8a'), 161.6 (C-5, C-5'), 168.9 (2-COOCH3, 2'-COOCH3), 175.3 (C-5'', C-5'''), 195.3 (C-4, C-4') ppm. IR (neat): ν (cm-1) = 2960, 1787, 1748, 1646, 1465, 1343, 1152, 1008, 733. UV (CH3CN): λmax (lg ε) = 196.0 (4.534), 256.0 (4.361), 359.0 (3.756). MS (ESI): m/z (%) = 1299.3 (100) [2M+Na]+, 661.2 (59) [M+Na]+. Calcd for C32H30O14: 661.1533 [M+Na]+, Found: 661.1530 [M+Na]+ (ESI-HRMS).
ACKNOWLEDGEMENTS
We thank the Deutsche Forschungsgemeinschaft (DFG), the state of Lower Saxony, the VW-foundation and the Fonds of the Chemical Industry for generous support. S.J. thanks the CaSuS Program and J.R.R. thanks the Konrad Adenauer Stiftung for Ph.D. scholarships, J.H. thanks the Dorothea Schlözer Fellowship Programme and B.G. thanks the Alexander von Humboldt Foundation for postdoctoral scholarships.
References
1. Z. Guo, Z. She, C. Shao, L. Wen, F. Liu, Z. Zheng, and Y. Lin, Magn. Reson. Chem., 2007, 45, 777. CrossRef
2. H. Kikuchi, M. Isobe, M. Sekiya, Y. Abe, T. Hoshikawa, K. Ueda, S. Kurata, Y. Katou, and Y. Oshima, Org. Lett., 2011, 13, 4624. CrossRef
3. a) W. Zhang, K. Krohn, Z. Ullah, U. Flörke, G. Pescitelli, L. Di Bari, S. Antus, T. Kurtán, J. Rheinheimer, S. Draeger, and B. Schulz, Chem. Eur. J., 2008, 14, 4913; CrossRef b) L. F. Tietze, L. Ma, J. R. Reiner, S. Jackenkroll, and S. Heidemann, Chem. Eur. J., 2013, 19, 4876; CrossRef c) T. Qin, R. P. Johnson and J. A. Porco, Jr., J. Am. Chem. Soc., 2011, 133, 1714; CrossRef for the syntheses of Blennolide B and C see: d) E. M. C. Gérard and S. Bräse, Chem. Eur. J., 2008, 14, 8086; CrossRef e) K. C. Nicolaou and A. Li, Angew. Chem., 2008, 120, 6681; CrossRef Angew. Chem. Int. Ed., 2008, 47, 6579; Ref. 3c). CrossRef
4. a) W. B. Turner, J. Chem. Soc., Perkin Trans. 1, 1978, 1621; CrossRef b) I. N. Siddiqui, A. Zahoor, H. Hussain, I. Ahmed, V. U. Ahmad, D. Padula, S. Draeger, B. Schulz, K. Meier, M. Steinert, T. Kurtán, U. Flörke, G. Pescitelli, and K. Krohn, J. Nat. Prod., 2011, 74, 365; CrossRef c) L. F. Tietze, S. Jackenkroll, C. Raith, D. A. Spiegl, J. R. Reiner, and M. C. Ochoa Campos, Chem. Eur. J., 2013, 19, 4876; CrossRef for other syntheses of diversonol see: d) M. C. Bröhmer, E. Bourcet, M. Nieger, and S. Bräse, Chem. Eur. J., 2011, 17, 13706; CrossRef e) K. C. Nicolaou and A. Li, Angew. Chem., 2008, 120, 6681; Angew. Chem. Int. Ed., 2008, 47, 6579; CrossRef f) C. F. Nising, U. K. Ohnemüller, and S. Bräse, Angew. Chem., 2006, 118, 313; CrossRef Angew. Chem. Int. Ed., 2006, 45, 307. CrossRef
5. For recent reviews on domino reactions, see: a) L. F. Tietze, M. A. Düfert, and S. C. Schild, in General Principles of Diastereoselective Reactions: ‘Diastereoselective Domino Reactions in Comprehensive Chirality’, Vol. 2 ed. by E. M. Carreira and H. Yamamoto, Elsevier, Amsterdam, 2012, pp. 97-121; b) L. F. Tietze, S. Stewart, and M. A. Düfert, in ‘Domino Reactions in the Enantioselective Synthesis of Bioactive Natural Products in Modern Tools for the Synthesis of Complex Bioactive Molecules’ ed. by J. Cossy and S. Arseniyades, Wiley, Hoboken, 2012; c) H. Pellissier, Adv. Synth. Catal., 2012, 354, 237; CrossRef d) S. Giboulot, F. Liron, G. Prestat, B. Wahl, M. Sauthier, Y. Castanet, A. Montreux, and G. Poli, Chem. Commun., 2012, 48, 5889; CrossRef e) M. Platon, R. Amardeil, L. Djakovitch, and J.-C. Hierso, Chem. Soc. Rev., 2012, 41, 3929; CrossRef f) L. F. Tietze and A. Düfert, Pure Appl. Chem., 2010, 82, 1375; CrossRef g) L. F. Tietze and A. Düfert, in Domino Reactions Involving Catalytic Enantioselective Conjugate Additions in ‘Catalytic Asymmetric Conjugate Reactions’ ed. by A. Cordova, Wiley-VCH, Weinheim, 2010, pp. 321-350; CrossRef h) C. Grondall, M. Jeanty, and D. Enders, Nat. Chem., 2010, 2, 167; CrossRef i) L. F. Tietze and L. Levy, in ‘The Mizoroki–Heck Reaction in Domino Processes’ ed. by M. Oestreich, Wiley, Chichester, 2008, pp. 281-344; j) L. F. Tietze, G. Brasche, and K. M. Gericke, ‘Domino Reactions in Organic Synthesis’; Wiley-VCH, Weinheim, 2006; CrossRef k) K. C. Nicolaou, D. J. Edmonds, and P. G. Bulger, Angew. Chem., 2006, 118, 7292; CrossRef Angew. Chem. Int. Ed., 2006, 45, 7134; CrossRef l) L. F. Tietze, Chem. Rev., 1996, 96, 115; CrossRef m) L. F. Tietze and U. Beifuss, Angew. Chem., 1993, 105, 137; CrossRef Angew. Chem., Int. Ed. Engl., 1993, 32, 131. CrossRef
6. a) R. Tanikaga, Y. Nozaki, T. Tamuraa, and A. Kaji, Synthesis, 1983, 134; CrossRef b) J. Nokami, T. Mandai, Y. Imakura, K. Nishiuchia, M. Kawada, and S. Wakabayashi, Tetrahedron Lett., 1981, 22, 4489; CrossRef c) S. Yamagiwa, H. Sato, N. Hoshi, K. Kosugia, and H. Uda, J. Chem. Soc., Perkin Trans. 1, 1979, 570. CrossRef
7. Z.-M. Wang and K. B. Sharpless, J. Org. Chem., 1994, 59, 8302. CrossRef
8. a) H. Hocke and Y. Uozumi, Tetrahedron, 2003, 59, 619; CrossRef b) T. D. Nelson and A. I. Meyers, J. Org. Chem., 1994, 59, 2655. CrossRef
9. a) S. Hanessian and K. Sumi, Synthesis, 1991, 1083; CrossRef b) S. Hanessian, N. Chahal, and S. Giroux, J. Org. Chem., 2006, 71, 7403; CrossRef c) N. Asao, S. Lee, and Y. Yamamoto, Tetrahedron Lett., 2003, 44, 4265; CrossRef d) J. Yang and G. B. Dudley, Tetrahedron Lett., 2007, 48, 7887; CrossRef e) Y. Chounan, Y. Ono, S. Nishii, H. Kitahara, S. Ito, and Y. Yamamoto, Tetrahedron, 2000, 56, 2821; CrossRef f) A. E. Dorigo and K. Morokuma, J. Am. Chem. Soc., 1989, 111, 6524; CrossRef g) Y. Yamamoto, S. Nishii, and T. lbuka, J. Chem. Soc., Chem. Commun., 1987, 464.
10. T. Satoh, J. Endo. H. Ota, and T. Chyouma, Tetrahedron, 2007, 63, 4806. CrossRef
11. a) P. Nguyen, E. Corpuz, T. M. Heidelbaugh, K. Chow, and M. E. Garst, J. Org. Chem., 2003, 68, 10195; CrossRef b) A. Cartoni, A. Madami, D. Palomba, M. Marras, M. Berettoni, L. Olivieri, A. Ettorre, A. Cipollone, F. Animati, C. A. Maggi, and E. Monteagudo, Tetrahedron, 2003, 59, 1309. CrossRef
12. A. J. Catino, J. M. Nichols, H. Choi, S. Gottipamula, and M. P. Doyle, Org. Lett., 2005, 7, 5167. CrossRef
13. T. K. M. Shing, Y.-Y. Yeung, and P. L. Su, Org. Lett., 2006, 8, 3149. CrossRef
14. a) K. L. Billingsley, T. E. Barder, and S. L. Buchwald, Angew. Chem., 2007, 119, 5455; CrossRef b) K. L. Billingsley, T. E. Barder, and S. L. Buchwald, Angew. Chem. Int. Ed., 2007, 46, 5359; CrossRef c) C. F. Nising, U. K. Schmid, M. Nieger, and S. Bräse, J. Org. Chem., 2004, 69, 6830. CrossRef
15. a) S. Kajigaeshi, T. Kakinami, T. Okamoto, H. Nakamura, and M. Fujikawa, Bull. Chem. Soc. Jpn., 1987, 60, 4187; CrossRef b) S. Kajigaeshi, T. Kakinami, H. Yamasaki, S. Fujisaki, and T. Okamoto, Bull. Chem. Soc. Jpn., 1988, 61, 2681. CrossRef