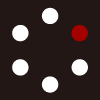
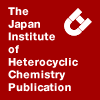
HETEROCYCLES
An International Journal for Reviews and Communications in Heterocyclic ChemistryWeb Edition ISSN: 1881-0942
Published online by The Japan Institute of Heterocyclic Chemistry
e-Journal
Full Text HTML
Received, 28th June, 2013, Accepted, 7th August, 2013, Published online, 14th August, 2013.
DOI: 10.3987/COM-13-S(S)61
■ Synthesis of p-tert-Butylcalix[4]thiacrowns Exhibiting Sulfur Number-Dependent Complexation with Mercury(II) Ion
Tatsuya Takimoto,* Hirohito Tsue, Hiroki Takahashi, Rui Tamura, and Hideaki Sasaki
Faculty of Pharmaceutical Sciences, Kobe Gakuin University, 1-1-3 Minatojima, Chuo-ku, Kobe 650-8586, Japan
Abstract
A novel synthetic pathway to calix[4]thiacrowns 3 and 4 has been developed. NMR studies revealed that the complexation behavior of 3 and 4 with mercury(II) ion was controlled by the number of sulfur atoms involved in the thiacrown moiety.The discharge of heavy metal ions into the environment is severely restricted by laws1 because a number of heavy metal ions are non-degradable and toxic not only to human beings, but also to animal life and even plant life. Once heavy metal ions are released unintentionally or accidentally into the environment, they may be biologically accumulated in our body through food chains.2 Among heavy metal ions, mercury ion has a lot of adverse effects on health when we are exposed to it in the long term even if its concentration is low.3 Therefore, in addition to the enactment of environment-related laws, a variety of effective adsorbents are being developed for removing mercury ion from the aqueous environment.
In this context, we previously synthesized p-tert-butylcalix[4]crowns 1 and 2 and applied them to an absorbent for removing organic and inorganic pollutants.4 Crystalline solids of 1 and 2 effectively removed organic pollutants from aqueous solution,5 but they showed no affinity for “soft” mercury ion because of the involvement of “hard” oxygen atoms in the crown moiety. This experimental fact prompted us to study analogous compounds 3 and 4 in which “soft” sulfur atoms are incorporated in the crown framework. However, an insuperable difficulty emerged in the preparation of a known compound 3 (vide infra), though we followed the synthetic protocols reported in a literature.6 Thus, we have newly devised a synthetic pathway to 3, and the strategy was applicable to the synthesis of a new compound 4. In this paper, we report the synthesis of calix[4]thiacrowns 3 and 4, together with their complexation behavior with mercury(II) ion.
We first tried to synthesize calix[4]thiacrown 3 according to the procedure described in a literature6 (Scheme 1). After tosylation of 3,6-dithiaoctane-1,8-diol,7 the resultant tosylate 6 was reacted with p-tert-butylcalix[4]arene (5) in anhydrous benzene using tert-BuOK as a base. However, contrary to the paper,6 calix[4]thiacrown 3 was not obtained. Instead, unreacted starting material 5 was recovered, and ring-closed 1,4-dithia-9-crown-3 (7) was only obtained from the reaction mixture.
This experimental result intended us to develop a different synthetic strategy. After our numerous efforts, we have finally devised a new synthetic pathway to calix[4]thiacrowns 3 and 4, as shown in Scheme 1. Starting from the regioselective introduction of bromoethyl groups onto the distal hydroxy groups of 5,8 the resultant 9 was subsequently reacted with dithiols 10 and 11 in THF and DMF, respectively, in the presence of Cs2CO3 to yield calix[4]thiacrowns 39 (32%) and 410 (53%) in a successful manner. The molecular structure of a new compound 4 was fully characterized by MS, NMR, IR, elemental analysis, and X-ray crystallographic analysis. Unfortunately, the single-crystal quality of 4 was not high for X-ray diffraction study, and the final R-value remained large (RW=0.164).11 Nonetheless, the conformation of the calixarene moiety of 4 was determined as cone, as shown in Figure 1.
The complexation behavior of calix[4]thiacrowns 3 and 4 toward mercury(II) picrate salt13 was investigated by means of 1H-NMR technique. As shown in Figures 2c and 2d, tert-butyl protons of 4 at 0.93 and 1.30 ppm experienced an upfield shift (Δδ = 0.12, 0.05 ppm) by the addition of mercury ion to the solution of 4, and this was also the case for the benzylic methylene protons at 4.36 ppm (Δδ = 0.26 ppm). The observed upfield shift is attributable to the conformatinal change of the calixarene moiety of 4 upon complexation with mercury ion in the thiacrown moiety; two distal benzene rings get closer to each other to undergo an enhanced ring current. Equally important is a large downfield shift (Δδ = 0.3–0.8 ppm) observed for the thiacrown moiety of 4, whose methylene protons adjacent to the sulfur atoms (3.02–4.14 ppm) are deshielded by a mercury cation bound to the sulfur atoms. The observed trends in the NMR spectra were in a good agreement with the study of Reinhoudt and co-workers,14 who reported that calix[4]crown 2 exhibited upfield and downfield shifts of the tert-butyl and oxacrown moieties, respectively, by the complexation with potassium ion. It is also noteworthy that hydroxy protons of 4 at 7.07 ppm show a large upfield shift (Δδ = 0.77 ppm) and a signal broadening, suggesting that intramolecular hydrogen bonds are
cleaved to allow the hydroxy groups to take part in coordination to mercury ion. A similarly large upfield shift (Δδ = 0.51 ppm) was observed for the picrate protons at 9.19 ppm and was conceivable by considering that complexation broke a contact ion pair of mercury cation and picrate anion to liberate the latter ion and hence recover the original electron density. On the other hand, all NMR signals of 3 and picrate ion were not shifted at all even in the presence of mercury ion (Figures 2a and 2b), indicating that two sulfur atoms in 3 were not enough to stabilize the coordination to mercury ion. In other words, three sulfur atoms are essential to stabilize and accommodate mercury ion in the cavity, at least, under the experimental conditions examined herein. Although the ring size of crown moiety seems an important factor influencing coordination ability, Huang and co-workers reported that neither 12, 13, nor 14 with similar sized crown moiety to 3 and 4 extracted mercury ion, which was only captured by 15 involving three soft donor atoms such as two sulfur atoms and one pyridyl group.15 As a result, in line with the Huang’s study, the present complexation study demonstrated that the complexation behavior of 3 and 4 with mercury ion was controlled by the number of sulfur atoms incorporated in the thiacrown moiety.
In conclusion, we have devised a novel synthetic pathway to calix[4]thiacrowns 3 and 4. Unlike calix[4]crowns 1 and 2, sulfur-containing derivative 4 forms a complex with mercury ion, though smaller homologue 3 captures no mercury ion because of its smaller number of sulfur atoms involved in the thiacrown moiety. Since the complexation ability of 3 and 4 for mercury ion is turned on and off by the number of sulfur atoms, we anticipate that calix[4]thiacrowns 3 and 4 can be applied to an adsorbent capable of selectively removing heavy metal ions. Investigations along this line are being continued in our laboratory to gain a further insight into the host–guest chemistry of these macrocycles.
References
1. The Basic Environment Law in Japan, Law No. 91 of 1993.
2. (a) G. M. Woodwell, C. F. Wurster, and P. A. Isaacson, Science, 1967, 156, 821; CrossRef (b) C. Theo, D. Dianne, and P. M. Johon, Our Stolen Future: Dutton, 1996.
3. Concise International Chemical Assessment Document, No. 50 Elemental Mercury and Inorganic Mercury Compound: Human Health Aspects, 2003.
4. (a) H. Tsue, T. Takimoto, R. Tamura, C. Kikuchi, and S. Tanaka, Lett. Org. Chem., 2004, 1, 276; CrossRef (b) H. Tsue, T. Takimoto, C. Kikuchi, H. Yanase, H. Takahashi, K. Amezawa, K. Ishibashi, S. Tanaka, and R. Tamura, Chem. Lett., 2005, 34, 1030; CrossRef (c) H. Tsue, T. Takimoto, C. Kikuchi, H. Yanase, K. Ishibashi, K. Amezawa, H. Miyashita, H. Miyafuji, S. Tanaka, and R. Tamura, Chem. Lett., 2006, 35, 254. CrossRef
5. T. Takimoto, Thesis, Kyoto University, 2006.
6. G. U. Akkus, S. Memon, and M. Yilmaz, Polycyclic Aromat. Compd., 2002, 22, 1075. CrossRef
7. M. Aizenberg, L. Turculet, and M. W. Davis, Organomet., 1998, 17, 4795. CrossRef
8. J. Xie, Q.-Y. Zheng, Y.-S. Zheng, C.-F. Chen, and Z.-T. Huang, J. Inclusion Phenom. Macrocyclic Chem., 2001, 40, 125. CrossRef
9. Synthesis of 3: A solution of 9 (1.00 g, 1.2 mmol) and 10 (109 mg, 1.2 mmol) in anhydrous THF (40 mL) was added dropwise to a suspension of Cs2CO3 (420 mg, 1.3 mmol) in anhydrous THF (140 mL) over a period of 45 min under Ar. After stirring for 5 days at 50 °C, the solvent was evaporated to dryness. The resulting residue was acidified with 1 M HCl and extracted with CH2Cl2 (70 mL). The organic layer was washed with saturated aqueous NaHCO3 and brine, dried over Na2SO4, filtrated, and evaporated under reduced pressure. The crude product was purified by chromatography on alumina using CH2Cl2–hexane (1:2, v/v) as an eluent to give 3 (298 mg, 32%) as a colorless solid. mp 303–311 °C; 1H-NMR (CDCl3, 270 MHz) δ 0.93 (18H, s), 1.30 (18H, s), 3.15 (4H, t, J=5.4 Hz), 3.33 (8H, m), 4.12 (4H, t, J=5.4 Hz), 4.27 (4H, d, J=13.5 Hz), 6.79 (4H, s), 7.07 (4H, s), 7.38 (2H, s); 13C-NMR (CDCl3, 125 MHz) δ 150.6, 149.3, 146.9, 141.2, 132.4, 127.1, 125.5, 124.7, 76.8, 33.95, 33.90, 33.1, 31.8, 31.7, 31.0.
10. Synthesis of 4: A solution of 9 (200 mg, 0.23 mmol) and 11 (36.2 mg, 0.23 mmol) in anhydrous DMF (9 mL) was added dropwise to a suspension of Cs2CO3 (83.2 mg, 0.26 mmol) in anhydrous DMF (32 mL) over a period of 7 min under Ar. After stirring for 18 h at room temperature, the solvent was evaporated to dryness. The resulting residue was acidified with 1 M HCl and extracted with CH2Cl2 (35 mL). The organic layer was washed with water and brine, dried over MgSO4, filtrated, and evaporated under reduced pressure. The crude product was purified by chromatography on alumina using CH2Cl2 as an eluent to give 4 (105 mg, 53%) as a colorless solid. mp 229–231 °C; 1H-NMR (CDCl3, 270 MHz) δ 0.93 (18H, s), 1.30 (18H, s), 3.02 (4H, t, J=7.3 Hz), 3.14 (4H, t, J=7.3 Hz), 3.19 (4H, t, J=5.5 Hz), 3.33 (4H, d, J=13.5 Hz), 4.14 (4H, t, J=5.5 Hz), 4.36 (4H, d, J=13.5 Hz), 6.74 (4H, s), 6.84 (2H, s), 7.07 (4H, s); 13C-NMR (CDCl3, 125 MHz) δ 150.4, 149.9, 147.1, 141.7, 132.3, 128.0, 125.6, 125.2, 33.9, 33.8, 33.7, 33.0, 32.1, 31.7, 31.6, 31.0; IR (KBr) ν 3500, 3048, 2961, 2905, 2866, 2359, 1485, 1462, 1361, 1300, 1202, 1123, 1003, 874; Anal. Calcd for C52H70O4S3: C, 73.02; H, 8.25; S, 11.25. Found: C, 72.88; H, 8.22; S, 11.34. MS (EI) m/z 854 (M+).
11. Crystal data for 4: triclinic, space group P-1, a=13.030(1) Å, b=16.708(2) Å, c=26.665(3) Å, α=95.596(9)°, β=96.137(8)°, γ=95.526(9)°, V=5710(1) Å3, Z=4, Dc=2.008 g cm–3, µ=2.25 cm–1, T=173(1) K, 18300 independent reflections (I > 3σ(I)), 1367 refined parameters, R=0.103 (I > 3σ(I)), Rw=0.164 (I > 3σ(I)), S=0.946. A copy of the data is available upon request to the authors.
12. L. J. Farrugia, J. Appl. Crystallogr., 1997, 30, 565. CrossRef
13. Synthesis of mercury(II) picrate: A solution of NaOH (1.09 g, 27.2 mmol) in Milli-Q water (16 mL) was added dropwise to a solution of Hg(NO3)2 (2.63 g, 8.10 mmol) in Milli-Q water (50 mL) under dark conditions. A yellow precipitate was collected by filtration and dissolved in Milli-Q water (30 mL). To the solution was added picric acid (3.77 g, 16.5 mmol). After stirring for 80 h at 50 °C, the reaction mixture was allowed to stand for 2 days at room temperature until precipitation was complete. After filtration, the resultant yellow solid was washed with Milli-Q water (24 mL) and benzene (25 mL), and dried in vacuo to give mercury(II) picrate (1.90 g, 34%) as a yellow solid. 1H-NMR (CDCl3, 270 MHz) δ 9.19 (4H, s). Anal. Calcd for C12H4N6O14Hg·2H2O: C, 20.80; H, 1.16; N, 12.13. Found: C, 20.58; H, 1.05; N, 12.05.
14. P. J. Dijkstra, J. J. A. Brunink, K. E. Bugge, D. N. Reinhoudt, S. Harkema, R. Ungaro, F. Ugozzoli, and E. Ghidini, J. Am. Chem. Soc., 1989, 111, 7567. CrossRef
15. J. Xie, Q.-Y. Zheng, Y.-S. Zheng, C.-F. Chen, and Z.-T. Huang, J. Inclusion Phenom. Macrocyclic Chem., 2001, 40, 125. CrossRef