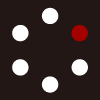
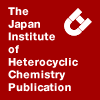
HETEROCYCLES
An International Journal for Reviews and Communications in Heterocyclic ChemistryWeb Edition ISSN: 1881-0942
Published online by The Japan Institute of Heterocyclic Chemistry
e-Journal
Full Text HTML
Received, 2nd July, 2013, Accepted, 17th September, 2013, Published online, 26th September, 2013.
DOI: 10.3987/COM-13-S(S)80
■ Facile Formation of Imidazolinium Salt by Reaction of Corresponding Diamine and Trimethyl Orthoformate in 1,1,1,3,3,3-Hexafluoroisopropanol
Kensuke Usui and Masahisa Nakada*
Department of Chemistry and Biochemistry, School of Advanced Science and Engineering, Waseda University, 3-4-1 Okubo, Shinjuku-ku, Tokyo 169-8555, Japan
Abstract
The preparation of imidazolinium salts, which can be used as precursors of pincer-type N-heterocyclic carbene ligands, is described. The formation of imidazolinium salts under standard conditions is difficult, but they have been successfully synthesized by reaction of the corresponding diamines and trimethyl orthoformate in 1,1,1,3,3,3-hexafluoroisopropanol. The synthesis of new chiral C2 symmetric imidazolinium salts is also described.N-Heterocyclic carbenes (NHCs) were first reported as organometallic complexes by Öfele1 and Wanzlick,2 and were isolated as stable crystals by Arduengo3 in 1968. Since their discovery, stable NHCs have been drawing attraction from many chemists because of their unique structures and properties. NHCs have been extensively studied, especially in oganometallic chemistry, as new ligands that can be used as substitutes for phosphine ligand.4 Recently, NHCs have also been recognized as organocatalysts.5 Research on NHCs is therefore expected to be further developed.
Pincer-type ligands have been studied since the early 1970s, and are known to form stable metal complexes6 by forming carbon–metal bonds and coordination bonds with σ-donor heteroatoms such as nitrogen and phosphorus. Metal complexes of pincer-type ligands show high and long-lasting catalytic activities, and have therefore been used for many catalytic reactions.
Consequently, pincer-type NHC ligands are expected to be useful. However, a limited number of pincer-type NHC ligands have been described,7,8 and their applications in asymmetric catalysis have not been reported. The development of new pincer-type NHC ligands could contribute to developments in organometallic chemistry as well as asymmetric catalysis.
We therefore decided to design and synthesize chiral C2 symmetric pincer-type NHC ligands 1 and 2, which are shown as their complexes in Figure 1. In this paper, we report the preparation of imidazolinium salts corresponding to 1 and 2, which are only formed in 1,1,1,3,3,3-hexafluoroisopropanol (HFIP).
We first prepared achiral diamine 7 (Scheme 1) to investigate the preparation and properties of the corresponding imidazolinium salts and NHCs. The first step in the synthesis of 7 was the preparation of 4 by a Suzuki–Miyaura coupling reaction. The palladium-catalyzed coupling reaction of o-nitrophenylboronic acid 3, which was prepared from 1-iodo-2-nitrobenzene according to the report by Yu,9 with 2-bromopyridine afforded 4 under standard conditions. Reduction of 4 with NaBH4 gave arylamine 5, which was converted to bis-amide 6 by reaction with oxallyl chloride; subsequent DIBAL-H reduction afforded diamine 7.
We next examined the conversion of diamine 7 to the corresponding imidazolinium salts (Table 1). However, none of the reactions using the reported conditions afforded imidazolinium salts 8 (entries 1–4). The low reactivity of diamine 7 was assumed to be the result of internal hydrogen bonding of the 1,2-diamine. The reaction was therefore carried out in 1-butanol to weaken the hydrogen bonds (entry 4). As a result, a polar compound was formed, which appeared on the base line in thin-layer chromatography, but was converted to the formamide of 7 during evaporation of the solvent. HFIP is a volatile solvent and easily removed under reduced pressure. Moreover, HFIP has been reported to be slightly acidic (pKa = 9.3) and a strong hydrogen-bond donor with high ionizing power and polarity; it therefore activates orthoesters towards nucleophilic attack of amino groups.10 So, we examined the reaction of 7 with trimethyl orthoformate in HFIP, and found that the reaction proceeded even at room temperature (entry 5) to afford imidazolinium salts 8a and 8b in79% and 73% yields, respectively.
Once imidazolinium salts 8a and 8b had been successfully synthesized, we proceeded to prepare chiral diamine 13 and its imidazolinium salts. 2-Bromophenyl benzyl ether 10, prepared from 2-bromophenol 9, was converted to compound 11 by a palladium-catalyzed coupling reaction. Benzyl ethers in 11 were removed by hydrogenolysis to afford bisphenol 12, which was successfully converted to the chiral diamine 13 by reaction with 2-fluoropyridine.
Diamine 13 was not converted to the corresponding imidazolinium salts under standard conditions (Table 2, entries 1-3); however, the reaction conditions used for the synthesis of 8 (Table 1, entries 5 and 6) significantly accelerated the formation of imidazolinium salts 13, even at room temperature, and 13a (entry 5) and 13b (entry 6) were successfully obtained in 59% and 73% yields, respectively.
Imidazolinium salts 8 and 14 were unstable and easily hydrolyzed and converted to the corresponding formamides. The formation of these salts was therefore confirmed by 1H-NMR spectroscopy and mass spectrometry of the crude products. Selected chemical shifts [δ (ppm), downfield from tetramethylsilane] of imidazolinium salts 8 and 14 are shown in Figure 2.
Imidazolinium salts 8 and 14 were found to be unstable in less-polar solvents, but, interestingly, were stable in polar solvents such as methanol and dimethyl sulfoxide (DMSO). For all the imidazolinium salts, the Hb signals were found at around 4 ppm (downfield from tetramethylsilane) in DMSO-d6, i.e., shifted downfield compared with the Hb signals of the corresponding diamines, and the Ha signals appeared at around 9 ppm, suggesting formation of the imidazolinium salts.
Once imidazolinium salts 14a and 14b were obtained as described above, we finally examined the synthesis of metal complexes of 14-M (Table 3). As the imidazolinium salts 14a and 14b were unstable, they were prepared just before the reactions in Table 3, and used after removing all volatile materials under reduced pressure.
We first examined the synthesis of silver complexes of 14a and 14b, which have been reported to be converted to various metal complexes.11 The reactions of 14a and 14b with Ag2O were carried out in the presence of MS 4A, but complex mixtures or only formamide 15 were obtained (entries 1–3).
We then examined the synthesis of palladium complexes of 14a and 14b using a base and Pd(OAc)2, but only observed the formation of complex mixtures (entries 4–6). The formation of 15 suggests that it might be derived from the NHC of 14b, which is too unstable to be isolated. Usually, imidazolinium salts are stable and isolable in air. The low stability of the NHC of 14 could therefore be attributed to a factor such as degradation accelerated via ylide formation by the formed carbene and pyridine nitrogen. The unsuccessful results in Table 3 will be useful in the further design and synthesis of new NHC ligands.
In conclusion, new chiral C2 symmetric imidazolinium salts were successfully synthesized. We found that the formation of imidazolinium salts was significantly enhanced, even at room temperature, when the reaction was carried out in HFIP. The use of HFIP in the synthesis of imidazolinium salts enables the formation of new imidazolinium salts that are difficult to prepare under known conditions. Further investigations of the use of HFIP in the preparation of imidazolinium salts are now underway, and the results will be reported in due course.
EXPERIMENTAL
1H and 13C NMR spectra were recorded on 400 MHz spectrometer. 1H and 13C chemical shifts are reported in ppm downfield from tetramethylsilane (TMS, δ scale) with the solvent resonances as internal standards. The following abbreviations were used to explain the multiplicities: s, singlet; d, doublet; t, triplet; q, quartet; m, multiplet; band, several overlapping signals; brs, broad. IR spectra were recorded on a FT/IR spectrometer. Mass spectra were provided at the Materials Characterization Central Laboratory, Waseda University. All reactions were carried out under an argon atmosphere with dry, freshly distilled solvents under anhydrous conditions, unless otherwise noted. All reactions were monitored by thin-layer chromatography carried out on 0.25 mm silica gel plates using UV light as visualizing agent and phosphomolybdic acid and heat as developing agents. Silica gel (60, particle size 0.040-0.063 mm) was used for flash chromatography. Preparative thin-layer chromatography (PTLC) separations were carried out on self-made 0.3 mm silica gel plates. THF was distilled from sodium/benzophenone ketyl. Toluene was distilled from sodium. MeOH was distilled with a small amount of magnesium and I2. All commercially available reagents were used without further purification. Optical rotations were measured on a polarimeterat a wavelength of 589 nm. High resolution mass spectra (HRMS) were obtained by either an electronspray ionization (ESI) or a fast atom bombardment (FAB), and theoretical monoisotopic molecular masses were typically ≤5 ppm. Melting point was uncorrected. Melting point was uncorrected. TLC Rfs of purified compounds were included.
2-(2-Nitrophenyl)pyridine (4).12 To a suspension of K2CO3 (4.65 g, 33.6 mmol), Pd(OAc)2 (0.189 g, 0.840 mmol), and PPh3 (0.882 g, 3.36 mmol) in 170 mL of DME/EtOH/H2O = 4/1/1 was added 2-nitrophenylboronic acid (3) (3.37 g, 20.2 mmol) and 2-bromopyridine (1.60 mL, 16.8 mmol). After stirring at 70 °C overnight, the reaction mixture was concentrated under reduced pressure. To the residue were added water (100 mL) and Et2O (100 mL), and the aqueous layer was extracted with Et2O (50 mL × 2). The combined organic layers were washed with brine (50 mL), dried over Na2SO4, and evaporated. The residue was purified by silica gel chromatography (hexane/EtOAc = 6/1) to afford 2-(2-nitrophenyl)pyridine (4) (2.23 g, 66%) as white solid. Spectroscopic data were in agreement with those previously reported:12 Rf = 0.28 (hexane/EtOAc = 2/1); 1H NMR (400 MHz, CDCl3): δ 8.66 (d, J = 5.0 Hz, 1H), 7.91 (d, J = 8.2 Hz, 1H), 7.81 (dd, J = 7.7, 7.7 Hz, 1H), 7.68 (d, J = 7.2 Hz, 1H), 7.63 (dd, J = 7.7, 7.7 Hz, 1H), 7.55 (dd, J = 7.7, 7.2 Hz, 1H), 7.48 (d, J = 8.2 Hz, 1H), 7.32 (dd, J = 7.7, 5.0 Hz, 1H).
2-(Pyridin-2-yl)benzenamine (5).13 To a solution of 2-(2-nitrophenyl)pyridine (4) (2.23 g, 11.1 mmol) in THF/ MeOH = 20/1 (230 mL) was added NaBH4 (2.53 g, 66.9 mmol). After stirring at room temperature for 3 days, the reaction mixture was concentrated under reduced pressure. To the residue were added water (100 mL) and Et2O (100 mL), and the aqueous layer was extracted with Et2O (50 mL × 2). The combined organic layers were washed with brine (50 mL), dried over Na2SO4, and evaporated. The residue was purified by silica gel chromatography (hexane/EtOAc = 10/1) to afford 2-(pyridin-2-yl)benzeneamine (5) (1.68 g, 89%) as a reddish liquid. Spectroscopic data were in agreement with those previously reported:13 Rf = 0.43 (hexane/EtOAc = 2/1); 1H NMR (400 MHz, CDCl3): δ 8.61 (d, J = 5.0 Hz, 1H), 7.77 (dd, J = 7.7, 7.7 Hz, 1H), 7.66 (d, J = 7.7 Hz, 1H), 7.51 (d, J = 7.7 Hz, 1H), 7.21–7.15 (m, 2H), 6.79 (dd, J = 7.7, 7.7 Hz, 1H), 6.77 (d, J = 7.7 Hz, 1H), 5.74 (br, 2H).
N1,N2-Bis(2-(pyridin-2-yl)phenyl)oxalamide (6). To a solution of 2-(pyridin-2-yl)benzeneamine (5) (1.68 g, 9.89 mmol) and Et3N (1.67 mL, 11.9 mmol) in dichloromethane (DCM) (50 mL) was added a solution of (COCl)2 (ca. 0.5 mL) in DCM (2 mL) dropwise at room temperature until the starting material disappeared. The resulting mixture was quenched with saturated aqueous NaHCO3 (20 mL), and the aqueous layer was extracted with DCM (20 mL × 2). The combined organic layers were washed with water (20 mL), dried over Na2SO4, and evaporated. The residue was purified by trituration (hexane/EtOAc = 2/1) to afford N1,N2-bis(2-(pyridin-2-yl)phenyl)oxalamide (6) (1.71 g, 88%) as a reddish brown solid: Rf = 0.41 (hexane/EtOAc = 2/1); 1H NMR (400 MHz, CDCl3): δ 13.68 (s, 2H), 8.88 (d, J = 4.9 Hz, 2H), 8.67 (d, J = 8.3 Hz, 2H), 7.84 (dd, J = 7.8, 7.8 Hz, 2H), 7.72 (d, J = 7.8 Hz, 2H), 7.71 (d, J = 7.8 Hz, 2H), 7.47 (dd, J = 7.8, 7.8 Hz, 2H), 7.31 (dd, J = 7.8, 4.9 Hz, 2H), 7.25 (dd, J = 8.3, 7.8 Hz, 2H); 13C NMR (100 MHz, CDCl3): δ 158.9, 157.5, 148.3, 137.6, 136.3, 129.9, 128.9, 127.3, 124.5, 122.6, 122.1, 121.8; IR (ATR): 3059.2, 1678.4, 1583.6, 1512.0, 1474.1, 1439.3, 1419.7, 1312.5, 753.6, 547.9 cm–1; mp 225 °C; HRMS (ESI) calcd. for C24H19O2N4 [M+H+] 395.1503, found 395.1502.
N1,N2-Bis(2-(pyridin-2-yl)phenyl)ethylenediamine (7). To a solution of N1,N2-bis(2-(pyridin-2- yl)phenyl)oxalamide (6) (105 mg, 0.266 mmol) in 1,4-dioxane (2.7 mL) was added DIBAL (1.02 M in toluene, 2.22 mL, 2.26 mmol). After stirring at 50 °C for 3 h, the resulting mixture was quenched with saturated aqueous Rochelle salt (3 mL) and diluted with Et2O (5 mL), and the aqueous layer was extracted with Et2O (5 mL × 2). The combined organic layers were washed with brine (5 mL), dried over Na2SO4, and evaporated. The residue was purified by silica gel chromatography (hexane/EtOAc = 15/1) to afford N1,N2-bis(2-(pyridin-2-yl)phenyl)ethylenediamine (7) (29.0 mg, 30%) as a yellow solid: Rf = 0.50 (hexane/EtOAc = 2/1); 1H NMR (400 MHz, CDCl3): δ 8.38 (br, 2H), 8.30 (d, J = 5.0 Hz, 2H), 7.70 (dd, J = 7.7, 7.7 Hz, 2H), 7.64 (d, J = 8.2 Hz, 2H), 7.53 (d, J = 7.7 Hz, 2H), 7.26 (dd, J = 8.2, 7.2 Hz, 2H), 7.07 (dd, J = 7.2, 5.0 Hz, 2H), 6.83 (d, J = 7.7 Hz, 2H), 6.73 (dd, J = 7.7, 7.7 Hz, 2H), 3.55 (s, 4H); 1H NMR (400 MHz, DMSO-d6): δ 8.62 (br, 2H), 8.23 (d, J = 5.0 Hz, 2H), 7.85 (dd, J = 7.7, 7.7 Hz, 2H), 7.80 (d, J = 8.2 Hz, 2H), 7.60 (d, J = 7.7 Hz, 2H), 7.23 (dd, J = 8.2, 7.7 Hz, 2H), 7.21 (dd, J = 7.7, 5.0 Hz, 2H), 6.82 (d, J = 7.7 Hz, 2H), 6.67 (dd, J = 7.7, 7.7 Hz, 2H), 3.46 (br, 4H); 13C NMR (100 MHz, CDCl3): δ 159.6, 147.6, 147.3, 136.7, 130.3, 129.4, 122.1, 121.6, 120.6, 115.8, 111.4, 42.6; IR (ATR): 3257.7, 3056.6, 2849.0, 1602.7, 1580.9, 1560.6, 1510.2, 1477.0, 1439.0, 744.2, 626.3 cm–1; mp 146.4 °C; HRMS (ESI) calcd. for C24H23N4 [M+H+] 367.1917, found 367.1917.
General procedure for the preparation of imidazolinium salts. To a suspension of diamine and NH4X (1.2 equiv. of diamine) in HFIP (0.1 M) was added CH(OMe)3 (10% w/w of HFIP). After stirring at room temperature for 1 h, to the mixture was added an internal standard, and then the excess CH(OMe)3 and HFIP were removed under reduced pressure to afford a crude residue. The residue was dissolved in DMSO-d6, and the solution was analyzed by 1H-NMR. NMR yields were obtained by careful integration based on the internal standard.
1,3-Bis(2-(pyridin-2-yl)phenyl)imidazolinium tetrafluoroborate (8a). According to general procedure, 1,3-bis(2-(pyridin-2-yl)phenyl)imidazolinium tetrafluoroborate (8a) was prepared from N1,N2-bis(2-(pyridin-2-yl)phenyl)ethylenediamine (7) (8.0 mg, 0.022 mmol) and NH4BF4 (2.7 mg, 0.026 mmol). The NMR yield was obtained using fluorene (3.9 mg, 0.024 mmol) as an internal standard (79%): Rf = 0.25 (DCM/MeOH = 10/1); 1H NMR (400 MHz, DMSO-d6): δ 8.92 (s, 1H), 8.71 (d, J = 4.6 Hz, 2H), 8.00 (dd, J = 7.8, 7.8 Hz, 2H), 7.78–7.74 (m, 2H), 7.72 (d, J = 7.8 Hz, 2H), 7.66–7.60 (m, 6H), 7.50 (dd, J = 7.8, 4.6 Hz, 2H), 4.17 (s, 4H); 13C NMR (100 MHz, DMSO-d6): δ 158.9, 157.2, 155.0, 149.5, 137.7, 136.0, 133.7, 131.2, 130.0, 127.3, 124.0, 123.2, 52.7; HRMS (ESI) calcd. for C25H21N4 [M-BF4-] 377.1761, found 377.1754.
1,3-Bis(2-(pyridin-2-yl)phenyl)imidazolinium chloride (8b). According to general procedure, 1,3-bis(2-(pyridin-2-yl)phenyl)imidazolinium chloride (8b) was prepared from N1,N2-bis(2-(pyridin-2-yl)phenyl)ethylenediamine (7) (9.1 mg, 0.025 mmol) and NH4Cl (1.6 mg, 0.030 mmol). The NMR yield was obtained using fluorene (5.2 mg, 0.031 mmol) as an internal standard (73%): Rf = 0.14 (DCM/MeOH = 10/1); 1H NMR (400 MHz, DMSO-d6): δ 8.89 (s, 1H), 8.68 (d, J = 5.0 Hz, 2H), 7.98 (dd, J = 7.7, 7.7 Hz, 2H), 7.75–7.72 (m, 2H), 7.70 (d, J = 7.7 Hz, 2H), 7.65–7.59 (m, 6H), 7.48 (dd, J = 7.7, 5.0 Hz, 2H), 4.18 (s, 4H) ppm; 13C NMR (100 MHz, DMSO-d6): δ 158.9, 157.3, 155.0, 149.5, 137.7, 136.0, 133.7, 131.1, 130.0, 127.3, 124.0, 123.2, 52.7 ppm; HRMS (ESI): calcd. for C25H21N4 [M-Cl-] 377.1761, found 377.1761.
2-Benzyloxybromobenzene (9).13 To a suspension of 2-bromophenol (8) (1.79 g, 10.4 mmol) and K2CO3 (2.86 g, 20.7 mmol) in DMF (10 mL) was added benzyl chloride (1.31 mL, 11.4 mmol). After stirring at 70 °C for 1.5 h, to the resulting mixture was added MeOH (0.5 mL), Et2O (20 mL), and water (20 mL), and the aqueous layer was extracted with Et2O (10 mL × 2). The combined organic layers were washed with water (20 mL) and brine (20 mL), dried over Na2SO4, and evaporated. The residue was purified by silica gel chromatography (hexane/EtOAc = 100/1) to afford 2-benzyloxybromobenzene (9) (2.69 g, 99%) as a clear liquid. Spectroscopic data were in agreement with those previously reported:13 Rf = 0.71 (hexane/EtOAc = 2/1); 1H NMR (400 MHz, CDCl3): δ 7.56 (d, J = 8.0 Hz, 1H), 7.48 (d, J = 7.3 Hz, 2H), 7.39 (dd, J = 7.3, 7.3 Hz, 2H), 7.33 (d, J = 7.3 Hz, 1H), 7.23 (dd, J = 8.0, 8.0 Hz, 1H), 6.94 (d, J = 8.0 Hz, 1H), 6.85 (d, J = 8.0 Hz, 1H) 5.17 (s, 2H).
(1R,2R)-N1,N2-Bis(2-benzyloxyphenyl)-1,2-cyclohexanediamine (10). To a suspension of NaOtBu (980 mg, 10.2 mmol), (R)-BINAP (254 mg, 0.407 mmol), and Pd2(dba)3 (156 mg, 0.170 mmol) in degassed toluene (12 mL), was added a solution of (1R,2R)-1,2-cyclohexanediamine (388 mg, 3.40 mmol) and 2-benzyloxybromobenzene (9) (2.24 g, 8.48 mmol) in degassed toluene (5 mL). After stirring at 140 °C for 25 h, the resulting mixture was diluted with Et2O (30 mL), filtered through a Celite® pad, and evaporated. The residue was purified by silica gel chromatography (hexane/EtOAc = 50/1) to afford (1R,2R)-N1,N2-bis(2-benzyloxyphenyl)-1,2-cyclohexanediamine (10) (1.46 g, 90%) as a clear viscous liquid: Rf = 0.35 (hexane/EtOAc = 10/1); 1H NMR (400 MHz, CDCl3): δ 7.26–7.12 (m, 10H), 6.88 (dd, J = 7.8, 7.8 Hz, 2H), 6.81 (d, J = 7.8 Hz, 2H), 6.71 (d, J = 7.8 Hz, 2H), 6.64 (dd, J = 7.8, 7.8 Hz, 2H), 4.99 (d, J = 12.0 Hz, 2H), 4.93 (d, J = 12.0 Hz, 2H), 4.57 (br, 2H), 3.30 (br, 2H), 2.31 (br, 2H), 1.75 (br, 2H), 1.43 (br, 2H), 1.31 (br, 2H); 13C NMR (100 MHz, CDCl3): δ 146.4, 138.4, 137.2, 128.4, 127.7, 127.1, 121.8, 116.3, 111.9, 110.5, 70.5, 57.0, 32.4, 24.5; IR (ATR): 3405.7, 3062.2, 2928.7, 2856.2, 1599.8, 1506.4, 1444.7, 1023.4, 732.0, 696.1 cm–1; [α]D27 +5.4 (c 0.0054, CHCl3); HRMS (ESI) calcd. for C32H35O2N2 [M+H+] 479.2693, found 479.2695.
(1R,2R)-N1,N2-Bis(2-hydroxyphenyl)-1,2-cyclohexanediamine dihydrochloride (11). To a solution of (1R,2R)-N1,N2-bis(2-benzyloxyphenyl)-1,2-cyclohexanediamine (10) (336 mg, 0.701 mmol) in MeOH/AcOH = 1/1 (8 mL) was added Pd/C (743 mg, 0.0701 mmol). After vigorously stirring under H2 atmosphere at room temperature for 5 days, the resulting mixture was diluted with EtOAc (10 mL) and filtered through a Celite® pad. The filtrate was added conc. HCl (0.2 mL) and evaporated, and then the residue was purified by trituration (EtOAc) to afford (1R,2R)-N1,N2-bis(2-hydroxyphenyl)-1,2-cyclohexanediamine dihydrochloride (11) (284 mg, quant.) as a gray powder: Rf (before hydrochloride salt) = 0.35 (hexane/EtOAc = 2/1); 1H NMR (400 MHz, DMSO-d6): δ 10.27 (br, 2H), 8.36 (br, 2H), 7.14 (d, J = 7.3 Hz, 2H), 6.95 (d, J = 7.3 Hz, 2H), 6.92 (dd, J = 7.3, 7.3 Hz, 2H), 6.80 (dd, J = 7.3, 7.3 Hz, 2H), 3.58 (br, 2H), 1.88 (br, 2H), 1.66 (br, 2H), 1.40 (br, 2H), 1.21 (br, 2H); 13C NMR (100 MHz, DMSO-d6): δ 148.9, 127.3, 124.9, 120.4, 119.3, 115.7, 58.7, 28.5, 23.5; IR (ATR): 2941.7, 2680.2, 1736.7, 1600.4, 1508.6, 1470.6, 1236.5, 1097.8, 1039.9, 751.1 cm–1; [α]D29–16.0 (c 0.0050, MeOH); mp 111 °C (decomposition); HRMS (ESI) calcd. for C18H22O2N2Na [M–2HCl+Na+] 321.1573, found 321.1574.
(1R,2R)-N1,N2-Bis(2-(pyridin-2-yloxy)phenyl)-1,2-cyclohexanediamine (12). To a suspension of NaH (60% in oil, 132 mg, 3.29 mmol) in DMF (3.3 mL) was added (1R,2R)-N1,N2-bis(2-hydroxyphenyl)-1,2-cyclohexanediamine dihydrochloride (11) and 2-fluoropyridine (0.283 mL, 3.29 mmol). After stirring at 120 °C for 2 days, to the resulting mixture were added water (5 mL) and Et2O (5 mL), and the aqueous layer was extracted with Et2O (5 mL × 2). The combined organic layers were washed with water (10 mL) and brine (5 mL), dried over Na2SO4, and evaporated. The residue was purified by silica gel chromatography (hexane/EtOAc = 4/1) to afford (1R,2R)-N1,N2-bis(2-(pyridin-2-yloxy)phenyl)-1,2-cyclohexanediamine (12) (103 mg, 35%) as a clear viscous liquid: Rf = 0.35 (hexane/EtOAc = 2/1); 1H NMR (400 MHz, CDCl3): δ 8.14 (d, J = 5.1 Hz, 2H), 7.51 (dd, J = 8.0, 7.0 Hz, 2H), 7.01 (dd, J = 8.0, 7.6 Hz, 2H), 6.93 (d, J = 7.6 Hz, 2H), 6.90 (dd, J = 7.0, 5.1 Hz, 2H), 6.71 (d, J = 8.0 Hz, 2H), 6.70 (d, J = 8.0 Hz, 2H), 6.34 (dd, J = 7.6, 7.6 Hz, 2H), 4.09 (br, 2H), 3.20 (br, 2H), 2.09 (br, 2H), 1.23 (br, 6H); 1H NMR (400 MHz, DMSO-d6): δ 8.06 (d, J = 4.9 Hz, 2H), 7.67 (dd, J = 8.2, 6.8 Hz, 2H), 7.01 (dd, J = 6.8, 4.9 Hz, 2H), 6.94 (dd, J = 7.7, 7.7 Hz, 2H), 6.83 (d, J = 8.2 Hz, 2H), 6.77 (d, J = 7.7 Hz, 2H), 6.75 (d, J = 7.7 Hz, 2H), 6.53 (dd, J = 7.7, 7.7 Hz, 2H), 4.54 (br, 2H), 3.28 (br, 2H), 1.92 (br, 2H), 1.58 (br, 2H), 1.18 (br, 4H); 13C NMR (100 MHz, CDCl3): δ 163.5, 147.8, 141.1, 140.4, 139.3, 125.9, 121.5, 118.4, 116.7, 112.3, 110.4, 56.4, 31.7, 24.0; IR (ATR): 3394.8, 3056.8, 2930.3, 2855.7, 1606.3, 1509.7, 1466.3, 1424.5, 1234.9, 736.5 cm-1; [α]D28 +1.36 (c 0.012, CHCl3); HRMS (ESI) calcd. for C28H28O2N4Na [M+Na+] 475.2104, found 475.2110.
(3aR,7aR)-1,3-Bis(2-(pyridin-2-yloxy)phenyl)-3a,4,5,6,7,7a-hexahydrobenzoimidazolium tetrafluoroborate (14a). According to general procedure, (3aR,7aR)-1,3-bis(2-(pyridin-2-yloxy)phenyl)-3a,4,5,6,7,7a-hexahydrobenzoimidazolium tetrafluoroborate (14a) was prepared from (1R,2R)-N1,N2-bis(2-(pyridin-2-yloxy)phenyl)-1,2-cyclohexanediamine (12) (13.1 mg, 0.029 mmol) and NH4BF4 (3.6 mg, 0.034 mmol). The NMR yield was obtained using phenanthrene (4.9 mg, 0.028 mmol) as an internal standard (59%): Rf = 0.28 (DCM/MeOH = 10/1); 1H-NMR (400 MHz, DMSO-d6): δ 9.47 (s, 1H), 8.11 (d, J = 5.0 Hz, 2H), 7.84 (dd, J = 8.2, 6.9 Hz, 2H), 7.60 (d, J = 7.8 Hz, 2H), 7.55 (dd, J = 7.8, 7.8 Hz, 2H), 7.42 (dd, J = 7.8, 7.8 Hz, 2H), 7.33 (d, J = 7.8 Hz, 2H), 7.17 (dd, J = 6.9, 5.0 Hz, 2H), 7.02 (d, J = 8.2 Hz, 2H), 3.92 (br, 2H), 2.06 (br, 2H), 1.76 (br, 2H), 1.42 (br, 2H), 1.19 (br, 2H); 13C NMR (100 MHz, DMSO-d6): δ 161.7, 160.6, 148.2, 147.4, 140.8, 130.8, 126.9, 126.8, 125.8, 123.2, 120.1, 111.7, 69.5, 27.0, 23.1; HRMS (ESI) calcd. for C29H27O2N4 [M-BF4–] 463.2129, found 463.2129.
(3aR,7aR)-1,3-Bis(2-(pyridin-2-yloxy)phenyl)-3a,4,5,6,7,7a-hexahydrobenzoimidazolium chloride (14b). According to general procedure, (3aR,7aR)-1,3-bis(2-(pyridin-2-yloxy)phenyl)-3a,4,5,6,7,7a-hexahydrobenzoimidazolium chloride (14-Cl) was prepared from (1R,2R)-N1,N2-bis(2-(pyridin-2-yloxy)phenyl)-1,2-cyclohexanediamine (12) (13.4 mg, 0.030 mmol) and NH4Cl (1.9 mg, 0.036 mmol). The NMR yield was obtained using phenanthrene (5.3 mg, 0.030 mmol) as an internal standard (73%): Rf = 0.13 (DCM/MeOH = 10/1); 1H-NMR (400 MHz, DMSO-d6): δ 9.47 (s, 1H), 8.08 (d, J = 5.4 Hz, 2H), 7.83 (dd, J = 8.6, 7.2 Hz, 2H), 7.61 (d, J = 8.2 Hz, 2H), 7.53 (dd, J = 8.2, 8.2 Hz, 2H), 7.41 (dd, J = 8.2, 8.2 Hz, 2H), 7.32 (d, J = 8.2 Hz, 2H), 7.15 (dd, J = 7.2, 5.4 Hz, 2H), 7.01 (d, J = 8.6 Hz, 2H), 3.91 (br, 2H), 2.04 (br, 2H), 1.74 (br, 2H), 1.40 (br, 2H), 1.18 (br, 2H); 13C NMR (100 MHz, DMSO-d6): δ 161.7, 160.6, 148.3, 147.4, 140.7, 130.8, 127.0, 126.9, 125.7, 123.1, 120.1, 111.7, 69.6, 27.0, 23.1; HRMS (ESI): calcd. for C29H27O2N4 [M-Cl–] 463.2129, found 463.2131.
ACKNOWLEDGEMENTS
This work was financially supported in part by a Grant-in-Aid for Challenging Exploratory Research (No. 23659014) from JSPS, Japan, the Global COE program "Center for Practical Chemical Wisdom" by MEXT, and a Waseda University Grant for Special Research Projects.
References
1. K. Öfele, J. Organomet. Chem., 1968, 12, 42. CrossRef
2. H.-W. Wanzlick and H. J. Schönherr, Angew. Chem., Int. Ed. Engl., 1968, 7, 141. CrossRef
3. A. J. Arduengo III, R. L. Harlow, and M. Kline, J. Am. Chem. Soc., 1991, 113, 361. CrossRef
4. (a) W. A. Herrmann, Angew. Chem. Int. Ed., 2002, 41, 1290; CrossRef (b) C. M. Crudden and D. P. Allen, Coord. Chem. Rev., 2004, 248, 2247; CrossRef (c) F. E. Hahn and M. C. Jahnke, Angew. Chem. Int. Ed., 2008, 47, 3122. CrossRef
5. (a) V. Nair, R. S. Menon, A. T. Biju, C. R. Sinu, R. R. Paul, A. Jose, and V. Sreekumar, Chem. Soc. Rev., 2001, 40, 5336; CrossRef (b) D. Enders, O. Niemeier, and A. Henseler, Chem. Rev., 2007, 107, 5606. CrossRef
6. (a) F. Miyazaki, K. Yamaguchi, and M. Shibasaki, Tetrahedron Lett., 1999, 40, 7379; CrossRef (b) J. T. Singleton, Tetrahedron, 2003, 59, 1837; CrossRef (c) N. Selander and K. J. Szabó, Chem. Rev., 2011, 111, 2048. CrossRef
7. (a) E. Peris and R. H. Crabtree, Coord. Chem. Rev., 2004, 248, 2239; CrossRef (b) D. Pugh and A. A. Danopoulos, Coord. Chem. Rev., 2007, 251, 610. CrossRef
8. (a) S. Gischig and A. Togni, Organometallics, 2004, 23, 2479; CrossRef (b) N. Schneider, V. César, S. Bellemin-Laponnaz, and L. H. Gade, Organometallics, 2005, 24, 4886; CrossRef (c) C. Michon, A. Ellern, and R. J. Angelici, Inorg. Chim. Acta, 2006, 359, 4549. CrossRef
9. S. E. Collibee and J. Yu, Tetrahedron Lett., 2005, 46, 4453. CrossRef
10. S. Khaksar, A. Heydari, M. Tajbakhsh, and S. M. Vahdat, J. Fluorine Chem., 2010, 131, 1377. CrossRef
11. (a) H. M. J. Wang and I. J. B. Lin, Organometallics, 1998, 17, 972; CrossRef (b) D. S. McGuinness and K. J. Cavell, Organometallics, 2000, 19, 741. CrossRef
12. L. Zhang, Z. Liu, H. Li, G. Fang, B.-D. Barry, T. A. Belay, X. Bi, and Q. Liu, Org. Lett., 2011, 13, 6536. CrossRef
13. E. Lee, J. M. Hooker, and T. Ritter, J. Am. Chem. Soc., 2012, 134, 17456. CrossRef
14. J. Mangas-Sánchez, E. Busto, V. Gotor-Fernández, and V. Gotor, Org. Lett., 2010, 12, 3498. CrossRef