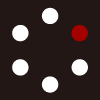
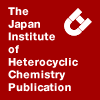
HETEROCYCLES
An International Journal for Reviews and Communications in Heterocyclic ChemistryWeb Edition ISSN: 1881-0942
Published online by The Japan Institute of Heterocyclic Chemistry
e-Journal
Full Text HTML
Received, 12th August, 2013, Accepted, 11th September, 2013, Published online, 17th September, 2013.
DOI: 10.3987/COM-13-S(S)110
■ Formation of Xanthone Oxime and Related Compounds Using a Combination of tert-Butyl Nitrite and Potassium Hexamethyldisilazide
Yusuke Iwama, Takahiro Noro, Kentaro Okano, Hidetsura Cho,* and Hidetoshi Tokuyama*
Department of Organic Chemistry, Graduate School of Pharmaceutical Sciences, Tohoku University, Aramaki, Aoba-ku, Sendai 980-8578, Japan
Abstract
Synthesis of xanthone oxime and related compounds via nitrosation of the dibenzylic position using a combination of tert-butyl nitrite and potassium hexamethyldisilazide is described. The reaction conditions are effective for the synthesis of xanthone oxime as well as thioxanthone, acridone, and anthrone oximes, which have been difficult to synthesize from the corresponding ketones by conventional dehydrative condensation with hydroxylamine.INTRODUCTION
Xanthone, thioxanthone, acridone, and anthrone oximes are highly attractive synthetic precursors for nitrogen-containing heterocycles since Beckmann rearrangement1 or other ring-expansion reactions2 provide the corresponding medicinally attractive nitrogen-containing tricyclic compounds. But despite their potential utility, there is no efficient method for preparation of these oximes. Thus, the conventional dehydrative condensation of the corresponding ketones and hydroxylamine is inefficient due to the low reactivity of the carbonyl group caused by the resonance effects of a heteroatom. Although several examples of dehydrative condensation with hydroxylamine have been documented in the literature, yields of the corresponding oximes were not reported in most cases.3 As an alternative route, a two-step method including conversion of ketone to more reactive thione followed by condensation with hydroxylamine was developed.1d,4 In addition to the classical dehydrative condensation of ketones and hydroxylamine, oximes have also been synthesized by several other methods.5 For example, nitrosation of alkane under radical conditions and subsequent tautomerization of the resultant nitroso compound gives the corresponding oxime;5c however, radicalic conditions under photo irraditation6 or using a combination of tert-butyl nitrite (t-BuONO) and N-hydroxyphthalimide,7 have a limited substrate scope. Nitrosation of enolizable methylene, such as the α-position of carbonyl or cyano groups,8 takes place under acidic conditions. Typical conditions such as nitrite salts and strong aqueous acids, however, promote electrophilic nitrosation on an aromatic ring9 and are inappropriate for the electron-rich aromatic compounds employed in this study.
With this background, we investigated oximation of 1 to xanthone oxime 2a and related compounds 2b-2e via nitroso compound 3 (Scheme 1).8a,10 Herein we report a formation of oxime using a combination of t-BuONO and potassium hexamethyldisilazide (KHMDS).
RESULTS AND DISCUSSION
At the outset of our research, we carefully investigated the conventional condensation of ketones and hydroxylamine (Table 1). Disappointingly, the reaction rate was slow to provide the corresponding oximes in low to moderate yields with recovery of the starting ketones 4a and 4b (entries 1 and 2). N-Boc acridone 4c was completely consumed in 12 h even at 80 °C; however, the desired oxime 2c was isolated in 21%. Instead, removal of the Boc group took place to provide acridone in 78% yield (entry 3). Reaction using N-methylacridone 4d resulted in recovery of 4d (entry 4), and on the other hand, reaction of anthorone 4e provided a complex mixture (entry 5).
Having confirmed the infeasibility of the conventional condensation for preparation of the desired oximes, we investigated our working hypothesis, using xanthene 1a11 as a substrate (Table 2). First, we examined a variety of bases (2.0 equiv.) using 2.0 equiv. of t-BuONO as a nitrosation reagent. While the use of KOt-Bu10b,10c provided oxime 2a in 78% yield with recovery of 6% starting xanthene 1a, more basic NaH gave none of the desired product 2a (entries 1 and 2). Alkyllithium, such as MeLi, n-BuLi, and t-BuLi, also provided minute amounts of the oxime with recovery of ca. 90% of the starting xanthene 1a (entries 3–5), which indicated that t-BuONO reacted with a base. To circumvent the undesired reaction, we used
hindered bases such as LDA and LiHMDS, and found that hexamethyldisilazide was the better base (entries 6 and 7). During the investigations, we observed a significant effect of counter ion of hexamethyldisilazide. Thus, the use of KHMDS improved the yield of the oxime up to 85% (entries 7–9). Finally, the desired oxime 2a was obtained in excellent yield even with the decreased amount (1.2 equiv.) of KHMDS and t-BuONO (entry 10).
We then focused on feasibility of the established optimal conditions for formation of the variety of oximes (Table 3). In addition to xanthene 1a, thioxanthene 1b11 and N-Boc-dihydroacridine 1c were successfully converted to oximes 2b or 2c in excellent yields respectively using 1.2 equiv. of t-BuONO and KHMDS (entries 2 and 3). In the case of N-methyldihydroacridine 1d,12 2.0 equiv. of t-BuONO and KHMDS were necessary for complete consumption of 1d to provide oxime 2d in 54% yield (entry 4). While dihydroanthracene 1e11 did not gave the desired oxime 2e under the optimal conditions using KHMDS, the use of n-BuLi provided oxime 2e in moderate yield.
In summary, we have established an efficient synthesis of oximes via nitrosation of dibenzylic position by using a combination of t-BuONO and KHMDS. This method would be an effective choice for oximation of xanthone, thioxanthone, acridone derivatives, and anthrone, which were difficult to be converted to the corresponding oximes by the conventional condensation of ketones with hydroxylamine.
EXPERIMENTAL
General Remarks: Materials were obtained from commercial suppliers and used without further purification unless otherwise mentioned. All reactions were carried out in oven-dried glassware under a slight positive pressure of argon unless otherwise noted. Anhydrous THF was purchased from Kanto Chemical Co. Inc. Anhydrous pyridine was dried and distilled according to the standard protocols. Flash column chromatography was performed on Silica Gel 60N (Kanto, spherical neutral, 40–50 µm) using the indicated eluent. Preparative TLC and analytical TLC were performed on Merck 60 F254 glass plates precoated with a 0.25 mm thickness of silica gel. IR spectra were measured on a SHIMADZU FTIR-8300 spectrometer or a JASCO FT/IR-4100 spectrometer. NMR spectra were recorded on a GX500 spectrometer and JNM-AL400 spectrometer. For 1H NMR spectra, chemical shifts are expressed in parts per million downfield from internal tetramethylsilane (δ 0) or relative internal CHCl3 (δ 7.26). For 13C NMR spectra, chemical shifts are expressed in ppm downfield from relative internal CDCl3 (δ 77.0). Mass spectra were recorded on a JEOL JMS-DX-303 spectrometer (EI), a JMS-T100GC spectrometer (EI), or a Bruker micrOTOF II (ESI). Elemental analyses were performed by a Yanaco CHN CORDER MT-5.
tert-Butyl 9-oxoacridine-10(9H)-carboxylate (4c): A 30-mL two-necked round-bottomed flask equipped with a magnetic stirring bar, a rubber septum, and a three-way stopcock was charged with acridone (402.0 mg, 2.06 mmol) and anhydrous MeCN (6.8 mL). To the solution was added Boc2O (761.9 mg, 3.49 mmol), followed by DMAP (753.7 mg, 6.17 mmol). The resulting mixture was stirred for 4 h, after which time TLC (hexanes-EtOAc = 4:1) indicated complete consumption of acridone. To the resulting mixture was added H2O, and the mixture was extracted with EtOAc three times. The combined organic extracts were washed with saturated aqueous NH4Cl and brine, dried over anhydrous sodium sulfate, and filtered. The organic solvents were removed under reduced pressure to give crude N-Boc-acridone 4c, which was purified by flash silica gel column chromatography (hexanes-EtOAc = 4:1) to afford N-Boc-acridone 4c (565.3 mg, 1.91 mmol, 93%) as a colorless solid. The analytical data (HRMS, 1H and 13C NMR) were identical with those reported in the literature.13 Rf = 0.45 (hexanes-EtOAc = 4:1); IR (neat, cm–1): 3446, 1743, 1636, 1604, 1488, 1459, 1369, 1360, 1246, 1178, 1142, 1121, 937, 840, 749, 670, 610, 554.
10-Methylacridin-9(10H)-one (4d): A 30-mL flame-dried round-bottomed flask equipped with a magnetic stirring bar, a rubber septum, and a three-way stopcock was charged with acridone (398.0 mg, 2.04 mmol) and anhydrous DMF (6.8 mL). To the solution was added NaH (164.0 mg, 4.10 mmol) at 0 °C and the resulting mixture was stirred at room temperature for 30 min. To the resulting mixture was added MeI (255 µL, 4.10 mmol) dropwise at 0 °C. The resulting mixture was stirred for 4 h, after which time TLC (hexanes-EtOAc = 1:1) indicated complete consumption of acridone. The reaction mixture was quenched with H2O, and the mixture was extracted with EtOAc three times. The combined organic extracts were washed with H2O and brine, dried over anhydrous sodium sulfate, and filtered. The organic solvents were removed under reduced pressure to give crude N-methylacridone 4d, which was purified by flash silica gel column chromatography (hexanes-EtOAc = 4:1) to afford N-methylacridone 4d (410.8 mg, 1.96 mmol, 96%) as a pale yellow solid. The analytical data (IR, HRMS, 1H and 13C NMR) were identical with those reported in the literature.14 Rf = 0.44 (hexanes-EtOAc = 1:1).
Procedure for dehydrative condensation of benzophenones and hydroxylamine.
9H-Xanthen-9-one oxime (2a): A 10-mL flame-dried screw-top test tube equipped with a magnetic stirring bar was charged with xanthone 4a (100.5 mg, 0.512 mmol), NH2OH·HCl (107.1 mg, 1.54 mmol) and anhydrous pyridine (0.51 mL). The resulting mixture was stirred at 110 °C for 24 h. To the resulting mixture was added H2O, and the mixture was extracted with EtOAc three times and CH2Cl2 once. The combined organic extracts were washed with brine, dried over anhydrous sodium sulfate, and filtered. The organic solvents were removed under reduced pressure to give crude xanthone oxime 2a. Residual pyridine was azeotropically removed with toluene. The residue was purified by flash silica gel column chromatography (CHCl3) to afford xanthone oxime 2a (48.0 mg, 0.227 mmol, 44%) as a colorless solid with recovery of xanthone 4a (56.5 mg, 0.288 mmol, 56%).
9H-Thioxanthen-9-one oxime (2b): A 10-mL flame-dried screw-top test tube equipped with a magnetic stirring bar was charged with thioxantone 4b (101.0 mg, 0.476 mmol), NH2OH·HCl (99.0 mg, 1.42 mmol) and anhydrous pyridine (0.48 mL). The resulting mixture was stirred at 110 °C for 24 h. To the resulting mixture was added H2O, and the mixture was extracted with CH2Cl2 three times. The combined organic extracts were washed with brine, dried over anhydrous sodium sulfate, and filtered. The organic solvents were removed under reduced pressure to give crude thioxanthone oxime 2b. Residual pyridine was azeotropically removed with toluene. The residue was purified by flash silica gel column chromatography (CHCl3 to toluene-Et2O = 3:2) to afford thioxanthone oxime 2b (27.9 mg, 0.123 mmol, 26%) as a yellow solid with recovery of thioxanthone 4b (69.6 mg, 0.328 mmol, 69%).
tert-Butyl acridine-10(9H)-carboxylate oxime (2c): A 10-mL flame-dried screw-top test tube equipped with a magnetic stirring bar was charged with N-Boc-acridone 4c (105.6 mg, 0.358 mmol), NH2OH·HCl (75.1 mg, 1.08 mmol) and anhydrous pyridine (0.36 mL). The resulting mixture was stirred at 80 °C for 12 h after which time TLC (hexanes-EtOAc = 4:1) indicated complete consumption of N-Boc-acridone 4c. To the resulting mixture was added H2O, and the mixture was extracted with THF twice and CH2Cl2 three times. The combined organic extracts were washed with brine, dried over anhydrous sodium sulfate, and filtered. The organic solvents were removed under reduced pressure to give crude N-Boc-acridone oxime 2c. Residual pyridine was azeotropically removed with toluene. The residue was purified by flash silica gel column chromatography (hexanes-EtOAc = 4:1 to CHCl3-MeOH = 9:1) to afford N-Boc acridone oxime 2c (23.6 mg, 0.076 mmol, 21%) as a colorless solid with recovery of acridone (54.4 mg, 0.279 mmol, 78%).
A 10-mL flame-dried screw-top test tube equipped with a magnetic stirring bar was charged with N-methylacridone (4d) (100.7 mg, 0.481 mmol), NH2OH·HCl (99.6 mg, 1.43 mmol) and anhydrous pyridine (0.48 mL). The resulting mixture was stirred at 110 °C for 24 h. To the resulting mixture was added H2O, and the mixture was extracted with CH2Cl2 three times. The combined organic extracts were washed with brine, dried over anhydrous sodium sulfate, and filtered. The organic solvents were removed under reduced pressure to give crude material. Residual pyridine was azeotropically removed with toluene. Purification by flash silica gel column chromatography (CHCl3) resulted in only recovery of N-methylacridone 4d (94.6 mg, 0.452 mmol, 94%).
General procedure for constructing oxime via electrophilic nitrosation: A flame-dried 30-mL two-necked round-bottomed flask equipped with a magnetic stirring bar was charged with starting material (100 mg) and anhydrous THF (0.1 M). To the solution was added 1.2 equiv. of t-BuONO at 0 °C, followed by 1.2 equiv. of KHMDS dropwise at 0 °C. The resulting solution was stirred for 30 min, after which time TLC indicated complete consumption of starting material. The reaction was quenched with saturated aqueous NH4Cl, and the aqueous layer was extracted three times with Et2O. The combined organic extracts were washed with brine, dried over anhydrous sodium sulfate, and filtered. The organic solvents were removed under reduced pressure to give a crude material. The residue was purified by flash silica gel column chromatography to afford the desired oxime.
9H-Xanthen-9-one oxime (2a): a colorless solid. Rf = 0.48 (hexanes-EtOAc = 4:1); IR (neat, cm–1): 3179, 3033, 2916, 1623, 1594, 1454, 1341, 1257, 998, 932, 738; 1H NMR (400 MHz, CDCl3): δ 9.01 (dd, 1H, J = 8.4, 1.2 Hz), 8.44 (brs, 1H), 8.00 (dd, 1H, J = 8.4, 1.2 Hz), 7.49 (ddd, 1H, J = 8.4, 7.2, 1.2 Hz), 7.42 (ddd, 1H, J = 8.4, 7.2, 1.2 Hz), 7.36-7.14 (m, 4H); 13C NMR (100 MHz, CDCl3): δ 152.8, 151.5, 141.4, 131.9, 131.6, 130.7, 124.0, 123.8, 122.8, 119.1, 117.3, 117.0, 116.1; HRMS (ESI) Calcd. for C13H10NO2 (M+H+), 212.0706; Found: 212.0711; Anal. Calcd for C13H9NO2: C, 73.92; H, 4.29; N, 6.63; Found: C, 73.99; H, 4.39; N, 6.59.
9H-Thioxanthen-9-one oxime (2b): a colorless solid. Rf = 0.47 (hexanes-EtOAc = 4:1); IR (neat, cm–1): 3174, 3042, 2906, 1587, 1435, 992, 923, 755, 727; 1H NMR (400 MHz, CDCl3): δ 8.57 (brs, 1H), 8.41 (dd, 1H, J = 8.0, 2.4 Hz), 7.84 (dd, 1H, J = 8.0, 1.6 Hz), 7.51 (dd, 1H, J = 7.6, 2.4 Hz), 7.43 (dd, 1H, J = 7.6, 1.6 Hz), 7.40–7.28 (m, 4H); 13C NMR (100 MHz, CDCl3): δ 149.0, 134.1, 132.6, 131.4, 131.0, 129.4, 128.8, 126.7, 126.5, 125.8, 125.6, 125.4 (one carbon is missing due to overlap); HRMS (ESI) Calcd. for C13H10NOS (M+H+), 228.0478; Found: 228.0483; Anal. Calcd for C13H9NOS: C, 68.70; H, 3.99; N, 6.16; Found: C, 68.87; H, 4.10; N, 6.15.
tert-Butyl acridine-10(9H)-carboxylate (1c): A two-necked 20-mL round-bottomed flask equipped with a magnetic stirring bar was charged with acridone (195 mg, 1.00 mmol), and THF (2.5 mL). To the solution was added BH3·THF (1.08 M in THF, 1.3 mL, 1.4 mmol) at room temperature. The reaction mixture was heated to reflux for 4 h, after which time TLC (hexanes-EtOAc = 3:1) indicated complete consumption of acridone. The reaction was quenched with brine and 2 M aqueous NaOH, and the mixture was extracted with Et2O three times. The combined organic extracts were washed with saturated aqueous NaHCO3, dried over anhydrous magnesium sulfate, and filtered. The filtrate was concentrated under reduced pressure to afford the crude dihydroacridine as a colorless solid, which was used for the next reaction without further purification.
A 50-mL round-bottomed flask equipped with a magnetic stirring bar was charged with dihydroacridine, Boc2O (766 mg, 3.51 mmol), and MeCN (3.3 mL). To the solution was added DMAP (203 mg, 1.66 mmol) at 0 °C. The solution was stirred for 11 h at room temperature, after which time TLC (hexanes-EtOAc = 9:1) indicated complete consumption of dihydroacridine. The reaction was diluted with Et2O. The mixture was washed with saturated aqueous NH4Cl and brine, dried over anhydrous sodium sulfate, filtered, and concentrated under reduced pressure. The residue was purified by silica gel column chromatography (hexanes-EtOAc = 9:1) to afford N-Boc-dihydroacridine 1c (226 mg, 0.803 mmol, 80%) as colorless crystals; Rf = 0.47 (hexanes-EtOAc = 9:1); IR (neat, cm–1): 1706, 1476, 1328, 1269, 1253, 1151, 760; 1H NMR (400 MHz, CDCl3): δ 7.63 (d, 2H, J = 8.0 Hz), 7.36–7.17 (m, 4H), 7.12 (d, 2H, J = 7.2 Hz), 3.80 (s, 2H), 1.54 (s, 9H); 13C NMR (125 MHz, CDCl3): δ 152.5, 138.8, 133.0, 126.9, 125.9, 125.04, 124.96, 33.8, 28.2, 27.9; HRMS (ESI): Calcd. for C14H12NO2 [(M–t-Bu)+], 226.0863; Found: 226.0878; Anal. Calcd for C18H19NO2: C, 76.84; H, 6.81; N, 4.98; Found: C, 76.64; H, 6.83; N, 5.01.
tert-Butyl 9-(hydroxyimino)acridine-10(9H)-carboxylate oxime (2c): a colorless solid. Rf = 0.35 (hexanes-EtOAc = 4:1); IR (neat, cm–1): 3071, 2974, 2928, 1716, 1598, 1463, 1322, 1251, 1159, 955, 923, 755; 1H NMR (400 MHz, CDCl3): δ 8.42 (dd, 1H, J = 8.0, 1.2 Hz), 8.23 (brs, 1H), 7.79 (d, 1H, J = 8.0 Hz), 7.78 (d, 1H, J = 8.0 Hz), 7.72 (dd, 1H, J = 8.0, 1.2 Hz), 7.41 (ddd, 1H, J = 8.0, 8.0, 1.2 Hz), 7.39 (ddd, 1H, J = 8.0, 8.0, 1.2 Hz), 7.27 (dd, 1H, J = 8.0, 8.0 Hz), 7.24 (dd, 1H, J = 8.0, 8.0 Hz), 1.53 (s, 9H); 13C NMR (100 MHz, CDCl3): δ 152.0, 146.4, 138.5, 137.5, 129.2, 129.0, 128.3, 128.2, 125.47, 125.42, 124.7, 124.6, 124.3, 124.2, 82.9, 28.1; HRMS (ESI) Calcd. for C18H19N2O3 (M+H+), 311.1390; Found: 311.1386; Anal. Calcd for C18H18N2O3: C, 69.66; H, 5.85; N, 9.03; Found: C, 69.79; H, 5.81; N, 8.94.
10-Methylacridin-9(10H)-one oxime (2d): a yellow solid. Rf = 0.28 (hexanes-EtOAc = 4:1); IR (neat, cm–1): 3004, 2891, 1593, 1464, 1362, 1265, 1175, 989, 918, 744; 1H NMR (400 MHz, CDCl3): δ 8.81 (d, 1H J = 8.4 Hz), 7.95 (dd, 1H, J = 8.4, 1.6 Hz), 7.46 (ddd, 1H, J = 8.4, 7.2, 1.6 Hz), 7.43 (ddd, 1H, J = 8.4, 7.2, 1.6 Hz), 7.22–7.05 (m, 4H), 3.57 (s, 3H); 13C NMR (100 MHz, CDCl3): δ 145.5, 142.3, 140.7, 131.2, 130.8, 129.8, 124.7, 121.3, 120.6, 119.6, 116.8, 113.4, 113.1, 34.0; HRMS (ESI) Calcd. for C14H13N2O (M+H+), 225.1022; Found: 225.1018; Anal. Calcd for C14H12N2O: C, 74.98; H, 5.39; N, 12.49; Found: C, 74.89; H, 5.45; N, 12.27.
Anthracen-9(10H)-one oxime (2e): A two-necked 30-mL round-bottomed flask equipped with a magnetic stirring bar was charged with dihydroanthracene 1e (100.2 mg, 0.556 mmol) and anhydrous THF (5.6 mL). To the solution was added n-BuLi (1.51 M in n-hexane, 0.736 mL, 1.11 mmol) dropwise at –78 °C. The resulting suspension was stirred for 5 min. To the mixture was added t-BuONO (0.132 mL, 1.11 mmol) at –78 °C. After the resulting solution was stirred for 30 min, the reaction was quenched with saturated aqueous NH4Cl, and the aqueous layer was extracted three times with Et2O. The combined organic extracts were washed with brine, dried over anhydrous sodium sulfate, and filtered. The organic solvents were removed under reduced pressure to give a crude material, which was purified by flash silica gel column chromatography (hexanes-acetone = 6:1) to afford oxime 2e (54.5 mg, 0.260 mmol, 47%) as a colorless solid. Rf = 0.43 (hexanes-EtOAc = 4:1); IR (neat, cm–1); 3063, 2917, 1450, 1331, 1160, 995, 928, 722, 711; 1H NMR (400 MHz, CDCl3): δ 8.53 (dd, 1H, J = 6.8, 2.0 Hz), 8.12 (brs, 1H), 7.86 (d, 1H, J = 6.8 Hz), 7.44–7.28 (m, 6H), 4.01 (s, 2H); 13C NMR (100 MHz, CDCl3): δ 149.4, 138.1, 135.9, 132.9, 130.6, 129.6, 128.7, 127.9, 127.9, 127.4, 126.9, 126.0, 124.8, 35.5; HRMS (ESI) Calcd. for C14H12NO (M+H+), 210.0913; Found: 209.0910; Anal. Calcd for C14H11NO: C, 80.36; H, 5.30; N, 6.69; Found: C, 80.13; H, 5.36; N, 6.60.
ACKNOWLEDGMENTS
This work was financially supported by the Ministry of Education, Culture, Sports, Science, and Technology, Japan; the KAKENHI, a Grant-in-Aid for Scientific Research (B) (20390003) and (C) (23590001); the Cabinet Office, Government of Japan through its “Funding Program for Next Generation World-Leading Researchers (LS008)”; JSPS predoctoral fellowship for Y.I.; Tohoku University Global COE program ‘International Center of Research and Education for Molecular Complex Chemistry’.
References
1. (a) L. G. Donaruma and W. Z. Heldt, Org. React., 1960, 11, 1; (b) R. E. Gawley, Org. React., 1988, 35, 1; (c) R. H. Williams and H. R. Snyder, J. Org. Chem., 1973, 38, 809; CrossRef (d) K. Nagarajan, C. L. Kulkarni, and A. Venkateswarlu, Ind. J. Chem., 1974, 12, 247; (e) A. K. Sinha and S. Nizamuddin, Ind. J. Chem. Sect. B, 1984, 23, 165.
2. (a) Y. Girault, M. Decouzon, and M. Azzaro, Bull. Soc. Chim. Fr., 1975, 385; (b) K. Hattori, Y. Matsumura, T. Miyazaki, K. Maruoka, and H. Yamamoto, J. Am. Chem. Soc., 1981, 103, 7368; CrossRef (c) S. Sasatani, T. Miyazaki, K. Maruoka, and H. Yamamoto, Tetrahedron Lett., 1983, 24, 4711; CrossRef (d) J. Fujiwara, H. Sano, K. Maruoka, and H. Yamamoto, Tetrahedron Lett., 1984, 25, 2367; CrossRef (e) K. Maruoka and H. Yamamoto, Angew. Chem., Int. Ed. Engl., 1985, 24, 668; CrossRef (f) Y. Torisawa, T. Nishi, and J. Minamikawa, Bioorg. Med. Chem. Lett., 2002, 12, 387; CrossRef (g) N. Willand, T. Beghyn, G. Nowogrocki, J.-C. Gesquiere, and B. Deprez, Tetrahedron Lett., 2004, 45, 1051; CrossRef (h) M. Ortiz-Marciales, L. D. Rivera, M. De Jesús, S. Espinosa, J. A. Benjamin, O. E. Casanova, I. G. Figueroa, S. Rodríguez, and W. Correa, J. Org. Chem., 2005, 70, 10132; CrossRef (i) P. P. Mukhopadhyay, O. Miyata, and T. Naito, Synlett, 2007, 1403; CrossRef (j) O. Miyata, T. Ishikawa, M. Ueda, and T. Naito, Synlett, 2006, 2219; CrossRef (k) H. Cho, K. Murakami, H. Nakanishi, H. Isoshima, K. Hayakawa, and I. Uchida, Heterocycles, 1998, 48, 919; CrossRef (l) H. Cho, K. Murakami, H. Nakanishi, A. Fujisawa, H. Isoshima, M. Niwa, K. Hayakawa, Y. Hase, I. Uchida, H. Watanabe, K. Wakitani, and K. Aisaka, J. Med. Chem., 2004, 47, 101; CrossRef (m) H. Cho, Y. Iwama, K. Sugimoto, E. Kwon, and H. Tokuyama, Heterocycles, 2009, 78, 1183; CrossRef (n) H. Cho, Y. Iwama, K. Sugimoto, S. Mori, and H. Tokuyama, J. Org. Chem., 2010, 75, 627; CrossRef (o) H. Cho, Y. Iwama, N. Mitsuhashi, K. Sugimoto, K. Okano, and H. Tokuyama, Molecules, 2012, 17, 7348; CrossRef (p) H. Cho, Y. Iwama, K. Okano, and H. Tokuyama, Synlett, 2013, 813. CrossRef
3. (a) T. Kurihara and H. Niwa, Yakugaku Zasshi 1953, 73, 1378 [Chem. Abstr., 1955, 313]; (b) N. Campbell, S. R. McCallum, and D. J. Mackenzie, J. Chem. Soc., 1957, 1922; (c) J. Lalevee, X. Allonas, F. Louerat, J.-P. Fouassier, H. Tachi, A. Izumitani, M. Shirai, and M. Tsunooka, Phys. Chem. Chem. Phys., 2001, 3, 2721; CrossRef (d) Y. S. Or, Y. Sun, and Z. Wang, WO2007 146695 A1; (e) Y. Sun, D. Niu, G. Xu, Y. S. Or, and Z. Wang, US2007 0281884 A1; (f) Y. Sun, Y. S. Or, and Z. Wang, US2008 187516 A1.
4. (a) F. G. Mann and J. H. Turnbull, J. Chem. Soc., 1951, 757; CrossRef (b) V. A. Prabhu, R. G. Brown, and J. N. Delgado, J. Pharm. Sci., 1981, 70, 558. CrossRef
5. (a) R. H. Williams and H. R. Snyder, J. Org. Chem., 1971, 36, 2327; CrossRef (b) M. Hafiz and G. A. Taylor, J. Chem. Soc., Perkin Trans. 1, 1980, 1700; CrossRef (c) R. SanMartín, R. Olivera, E. M. de Marigorta, and E. Domínguez, Tetrahedron, 1995, 51, 5361; CrossRef (d) M. Yonekawa, Y. Koyama, S. Kuwata, and T. Takata, Org. Lett. 2012, 14, 1164. CrossRef
6. (a) E. V. Lynn, J. Am. Chem. Soc., 1919, 41, 368; CrossRef (b) E. V. Lynn and O. Hilton, J. Am. Chem. Soc., 1922, 44, 645; CrossRef (c) E. V. Lynn and H. L. Arkley, J. Am. Chem. Soc., 1923, 45, 1045. CrossRef
7. (a) T. Hirabayashi, S. Sakaguchi, and Y. Ishii, Angew. Chem. Int. Ed., 2004, 43, 1120; CrossRef (b) Y. Ishii, S. Sakaguchi, and T. Iwahama, Adv. Synth. Catal., 2001, 343, 393. CrossRef
8. (a) O. Touster, Org. React., 1953, 7, 327; (b) J. Beger and C. Thielemann, J. Prakt. Chem., 1981, 323, 137; CrossRef (c) J. Beger and C. Thielemann, J. Prakt. Chem., 1981, 323, 337; CrossRef (d) R. N. Loeppky, J. Shi, C. L. Barnes, and S. Geddam, Chem. Res. Toxicol., 2008, 21, 295. CrossRef
9. (a) E. Bosch and J. K. Kochi, J. Org. Chem., 1994, 59, 5573; CrossRef (b) R. J. Maleski, M. Kluge, and D. Sicker, Synth. Commun., 1995, 25, 2327; CrossRef (c) M. González-Jiménez, J. Arenas-Valgañón, E. Calle, and J. Casado, Org. Biomol. Chem., 2011, 9, 7680. CrossRef
10. (a) A. D. Dunn and K. I. Kinnear, J. Heterocycl. Chem., 1985, 22, 311; CrossRef (b) M. Le Rudulier and C. Moinet, J. Organomet. Chem., 1988, 352, 337; CrossRef (c) M. C. Senechal-Tocquer, D. Senechal, J. Y. Le Bihan, D. Gentric, B. Caro, M. Gruselle, and G. Jaouen, J. Organomet. Chem., 1992, 433, 261. CrossRef
11. Xanthene 1a, thioxanthene 1b, and dihydroanthracene 1e are commercially available compounds.
12. N-Methyldihydroacridine 1d was prepared from known procedure, which is described in following paper; Á. Pintér, A. Sud, D. Sureshkumar, and M. Klussmann, Angew. Chem. Int. Ed., 2010, 49, 5004. CrossRef
13. A. A. Kulago, E. M. Mes, M. Klok, A. Meetsma, A. M. Brouwer, and B. L. Feringa, J. Org. Chem., 2010, 75, 666. CrossRef
14. X.-A. Li, H.-L. Wang, and S.-D. Yang, Org. Lett., 2013, 15, 1794. CrossRef