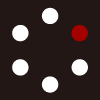
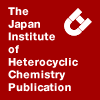
HETEROCYCLES
An International Journal for Reviews and Communications in Heterocyclic ChemistryWeb Edition ISSN: 1881-0942
Published online by The Japan Institute of Heterocyclic Chemistry
e-Journal
Full Text HTML
Received, 19th May, 2013, Accepted, 19th June, 2013, Published online, 5th July, 2013.
DOI: 10.3987/COM-13-S(S)15
■ New, Lithiation-Based Synthesis of Tofisopam, a 2,3-Benzodiazepine Type Anxiolytic Drug
Erika Molnárné Samu,* Gyula Lukács, Balázs Volk, and Gyula Simig
Chemical Research Deivision, EGIS Pharmaceuticals PLC, 1106 Hungary, Budapest,
Keresztúri út 30-38, Hungary
Abstract
The classical, widely-used synthesis of the anxiolytic drug tofisopam applies chromium(VI) oxide reagent for the synthesis of the diketone key intermediate. The chromium salts formed in the course of the reaction are strongly toxic compounds. In order to reduce the reagents contributing to the pollution of the environment, an alternative manufacturing process has been elaborated at our laboratory. Starting compound (3,4-dimethoxyphenyl)acetone was transformed to the ethylene ketal of (2-bromo-4,5-dimethoxyphenyl)acetone. Bromine-lithium exchange and introduction of a 3,4-dimethoxybenzoyl moiety resulted in the new key intermediate. Removal of the ketal protecting group and ring closure with hydrazine was carried out in one pot affording drug substance tofisopam.INTRODUCTION
Tofisopam (1, marketed under brand names Grandaxin®,1 and Emandaxin®) is a 2,3-benzodiazepine type anxiolytic drug. In contrast to the widely used 1,4-benzodiazepines, the compound does not bind to the distinct benzodiazepine binding site of the GABAA (γ-aminobutyric acid) receptor and its psychopharmacological and clinical profile differ from those of such compounds. It is an anxiolytic agent without the sedative-hypnotic, muscle relaxant and anticonvulsive side effects generally associated with the use of 1,4-benzodiazepines. Moreover, it has no addiction potential.2
The manufacturing syntheses of tofisopam (1) have been based on natural precursors.3 Isoeugenol (2, Scheme 1) is a component of essential oils of plants and easily available also from eugenol (its isomer of the same natural origin) by double bond migration. Acid catalyzed dimerisation of isoeugenol (2)4 afforded diisoeugenol (3), which was O-methylated to diisohomoeugenol (4).5 Alternatively, diisohomoeugenol (4) can be prepared by methylation of isoeugenol to isohomoeugenol (5), followed by dimerization.6 The stereochemistry of intermediates 3 and 4 was usually not investigated, regarding its irrelevance in the next reaction step, in the course of the elaboration of tofisopam synthesis. Nevertheless, the mechanism of the dimerisation of various styrenes and the stereochemistry of the resulting indanes have been discussed in several papers.6b,7
3-{2-[(3,4-Dimethoxyphenyl)carbonyl]-4,5-dimethoxyphenyl}pentan-2-one (diketone, 6) was obtained by oxidation of diisohomoeugenol (4) with chromium(VI) oxide.8,9 Diketone 6 was cyclized directly or via the corresponding 2-benzopyrylium salt 7 to tofisopam (1) with hydrazine hydrochloride or hydrazine hydrate.3 Chromium(VI) oxide applied as the oxidizing agent and the chromium salts formed in the course of the reaction are strongly toxic compounds damaging the environment.
An alternative procedure involving the oxidation of isochroman derivative 8 (Scheme 2) with chromium(VI) oxide to diketone 6 has the same disadvantage, i.e. the use of Jones reagent. Isochroman 8 was prepared starting from (3,4-dimethoxyphenyl)acetone (9). Ethylation of 9 at the benzylic carbon10,11 to give 10, followed by reduction gave alcohol 11, which was treated with 3,4-dimethoxybenzaldehyde to afford compound 8.9,11
In order to avoid the necessity of the oxidation with the toxic chromium reagent, Grasser et al. prepared diketone 6 from ketone 10 by treatment with 3,4-dimethoxybenzoyl chloride in the presence of aluminum chloride under classical Friedel-Crafts conditions either directly or via the benzopyrilium salt (7),12 depending on the work-up. However, the scale-up of this procedure did not result in a feasible manufacturing synthesis of tofisopam (1) in our hands, probably because of the O-demethylation side reactions caused by aluminum chloride on the compounds containing several methoxy groups.13 It is noteworthy that our efforts to carry out the benzoylation reaction of 10 in polyphosphoric acid or with phosphorus pentoxide in various solvents failed,14 contrary to some analogies described in the literature.15
RESULTS AND DISCUSSION
In the modern pharmaceutical manufacturing processes, there must be a focus on the reduction or complete elimination of reagents that contribute to the pollution of the environment. The present paper describes a new industrial synthesis of tofisopam (1), which eliminates the use of the environmentally hazardous chromium reagents. Our strategy was to carry out the 3,4-dimethoxybenzoylation of compound 10 by applying lithiation chemistry instead of Friedel-Crafts reaction.16
Prior to lithiation the ketone 10 has to be protected suitably, e.g. as a ketal. Direct lithiation of such a ketal is expected to occur at the doubly activated position between the methoxy group and the side-chain containing the ketal moiety. However, bromination of starting material 10 with NBS led to bromo derivative 12 in a regioselective manner (Scheme 3), affording the possibility of the introduction of the 3,4-dimethoxybenzoyl group in the required position after protection of the keto function and bromine-lithium exchange.
Bromo-ketone 12 was transformed to the corresponding ethylene ketal 13 in high yield. Lithiation was carried out at low temperature (–40 °C) with hexyllithium, the use of which is more advantageous for manufacturing purposes than that of butyllithium. Subsequent quenching of the lithio species with N,N-dimethyl-3,4-dimethoxybenzamide16b,17 gave keto-ketal 14. In this key reaction step, use of reagents other than N,N-dimethyl-3,4-dimethoxybenzamide was also considered. Nevertheless, all these attempts proved disadvantageous compared to the amide. When ethyl 3,4-dimethoxybenzoate was used, the expected 14 was obtained as a minor product.18 Use of the corresponding benzonitrile also led to significant side-reactions, in accord with the findings of Brovchenko et al. described for analogous compounds.19 Finally, N,3,4-trimethoxy-N-methylbenzamide (Weinreb amide)20 was discarded for cost calculation reasons.
The optimal reaction conditions elaborated for the cyclisation of compounds 6 and 7 to tofisopam (1) are appropriate for the removal of the ketal group, as well.3 Therefore, the transformation of keto-ketal 14 to tofisopam (1), which requires the removal of the ketal protecting group before the cyclisation, was carried out in one pot to afford tofisopam (1) in good yield.18
A new manufacturing procedure has been elaborated at our laboratory for the synthesis of the drug substance tofisopam (1). The keto-ketal key intermediate (14) was obtained using lithiation chemistry instead of the generally used, environmentally harmful oxidation approach leading to the corresponding diketone 6.
EXPERIMENTAL
All melting points were determined on a Büchi 535 capillary melting point apparatus. IR spectra were obtained on a Bruker IFS-113v FT spectrometer in KBr pellets. 1H NMR and 13C NMR spectra were recorded on Bruker Avance III (400 and 100 MHz for 1H and 13C NMR spectra, respectively) spectrometer. CDCl3 or DMSO-d6 was used as solvent and tetramethylsilane (TMS) as internal standard. Chemical shifts (δ) and coupling constants (J) are given in ppm and in Hz, respectively. Elemental analyses were performed on a Perkin-Elmer 2400 analyzer. The reactions were followed by analytical thin layer chromatography on silica gel 60 F254. All unspecified reagents were purchased from commercial sources.
3-(3,4-Dimethoxyphenyl)pentan-2-one (10).12 To a stirred solution of NaOH (120 g, 3.0 mol) in water (120 mL), benzyltrimethylammonium hydroxide (Triton B, 40 w/w% solution in MeOH, 5.0 mL, 4.6 g, 0.011 mol) and (3,4-dimethoxyphenyl)acetone (194.2 g, 1.0 mol) was added iodoethane (123.7 mL, 187.2 g, 1.2 mol) over a period of 15 min, while keeping the temperature at 10–15 ºC. The mixture was stirred for 6 h at 60 ºC. After cooling to ambient temperature the mixture was extracted with t-BuOMe (200 mL). The organic layer was dried (MgSO4) and evaporated to give a light brown oil (202.4 g, 91%), which could be used in the next reaction without further purification. IR (KBr): 1715 cm–1. 1H NMR (CDCl3, 400 MHz): 6.83 (d, 1H, J = 8.2 Hz), 6.77 (dd, 1H, J = 8.2, 2.0 Hz), 6.69 (d, 1H, J = 2.1 Hz), 3.87 (s, 6H), 3.45 (dd, 1H, J = 7.4 Hz), 2.06 (s, 3H), 2.02 (m, 1H), 1.70 (m, 1H), 0.84 (t, 3H, J = 7.4 Hz) ppm. 13C NMR (CDCl3, 100 MHz): 208.7, 149.1, 148.1, 131.3, 120.6, 111.3, 110.8, 60.9, 55.8, 55.8, 28.8, 24.8, 11.9 ppm. Anal. Calcd for C13H18O3 (222.29): C 70.24, H 8.16%. Found: C 70.13, H 8.32%.
3-(2-Bromo-4,5-dimethoxyphenyl)pentan-2-one (12). N-Bromosuccinimide (123.7 g, 0.66 mol) was added to a solution of 3-(3,4-dimethoxyphenyl)pentan-2-one (10, 133.4 g, 0.60 mol) in EtOAc (400 mL) at 0–5 ºC, over a period of 2.5 h and the mixture was stirred for further 30 min at the same temperature. It was cooled to –6 ºC and the precipitate (succinimide) was separated by filtration. The solvent was evaporated and the residue was distilled in vacuo (bp 144–147 ºC/20 Pa) to give a yellow oil (153.6 g, 85%). IR (film): 1715 cm–1. 1H NMR (DMSO-d6, 400 MHz): 7.19 (s, 1H), 6.67 (s, 1H), 3.99 (dd, 1H, J = 7.9, 6.7 Hz), 3.79 (s, 3H), 3.73 (s, 3H), 2.02 (s, 3H), 1.98 (m, 1H), 1.64 (m, 1H), 0.77 (t, 3H, J = 7.4 Hz) ppm. 13C NMR (DMSO-d6, 100 MHz): 207.1, 148.8, 148.7, 130.0, 115.8, 114.9, 111.5, 58.5, 56.0, 55.8, 29.2, 24.1, 11.7 ppm. Anal. Calcd for C13H17BrO3 (301.18): C 51.84, H 5.69, Br 26.53%. Found: C 51.68, H 5.74, Br 26.15%.
2-[1-(2-Bromo-4,5-dimethoxyphenyl)propyl]-2-methyl-1,3-dioxolane (13). 3-(2-Bromo-4,5-dimethoxyphenyl)pentan-2-one (12, 131.0 g, 0.435 mol), ethylene glycol (54.7 mL, 60.9 g, 0.982 mol) and para-toluenesulfonic acid (14.6 g) were refluxed in toluene (730 mL) for 24 h in a flask equipped with a Dean-Stark apparatus. After cooling to ambient temperature the solution was extracted with aqueous sodium hydrogencarbonate solution (5 w/w%, 2×200 mL), dried (MgSO4) and evaporated. The residue was distilled in vacuo (bp 149–152 ºC/15 Pa) to give a colorless oil (127 g, 86%), which solidified upon standing (mp 49–51 ºC). 1H NMR (CDCl3, 400 MHz): 7.05 (s, 1H), 7.01 (s, 1H), 3.99–3.82 (m, 4H), 3.86 (s, 6H), 3.45–3.42 (m, 1H), 1.99–1.94 (m, 1H), 1.65–1.58 (m, 1H), 1.18 (s, 3H), 0.72 (t, 3H, J = 7.3 Hz) ppm. 13C NMR (CDCl3, 100 MHz): 148.3, 147.9, 132.5, 117.0, 114.8, 111.5, 111.5, 65.4, 64.6, 55.9, 55.9, 53.4, 23.2, 23.1, 11.6 ppm. Anal. Calcd for C15H21BrO4 (345.24): C 52.19, H 6.13, Br 23.14%. Found: C 52.36, H 6.12, Br 23.23%.
{4,5-Dimethoxy-2-[1-(2-methyl-[1,3]dioxolan-2-yl)-propyl]-phenyl}-(3,4-dimethoxy-phenyl)-methanone(14). A solution of hexyllithium (2.3 M in hexane, 47.8 mL, 0.110 mol) was added to a solution of 2-[1-(2-bromo-4,5-dimethoxyphenyl)propyl]-2-methyl-1,3-dioxolane (13, 34.5 g, 0.100 mol) in toluene (520 mL) at –40 ºC. After stirring at –40 ºC for 30 min 3,4-dimethoxy-N,N-dimethylbenzamide21 (25.1 g, 0.12 mol) was added. The temperature of the reaction mixture increased to 0 ºC and it was stirred for further 90 min. Saturated aqueous solution of ammonium chloride (100 mL) was added at 0 ºC and the layers were separated. The organic layer was extracted with water (100 mL), dried (MgSO4) and evaporated. The crystalline residue was triturated with EtOH (180 mL) at 0 ºC to give 14 (26.2 g, 61%) as colorless crystals, mp 165–166 ºC (EtOH). IR (KBr): 1638 cm–1. 1H NMR (CDCl3, 400 MHz): 7.54 (d, 1H, J = 2.0 Hz), 7.31 (dd, 1H, J = 8.4, 2.0 Hz), 7.17 (s, 1H), 6.84 (d, 1H, J = 8.4 Hz), 6.79 (s, 1H), 3.95 (s, 6H), 3.92 (s, 3H), 3.88 (m, 1H), 3.81 (s, 3H), 3.78 (m, 1H), 3.71 (m, 2H), 3.25 (dd, 1H, J = 11.3, 3.6 Hz), 1.91 (m, 1H), 1.71 (m, 1H), 1.18 (s, 3H), 0.76 (t, 3H J = 7.3 Hz) ppm. 13C NMR (CDCl3, 100 MHz): 196.6, 153.1, 149.9, 148.8, 146.1, 133.8, 133.3, 131.4, 126.0, 111.7, 111.7, 111.3, 111.1, 109.6, 65.1, 64.5, 56.0, 55.9, 55.9, 55.9, 49.6, 23.1, 23.0, 12.2 ppm. Anal. Calcd for C24H30O7 (430.50): C 66.96, H 7.02%. Found: C 67.07, H 7.01%.
1-(3,4-Dimethoxyphenyl)-5-ethyl-7,8-dimethoxy-4-methyl-5H-2,3-benzodiazepine (1, tofisopam). A mixture of {4,5-dimethoxy-2-[1-(2-methyl-[1,3]dioxolan-2-yl)-propyl]-phenyl}-(3,4-dimethoxy-phenyl)-methanone (14, 43.1 g, 0.100 mol), glacial acetic acid (46.0 mL, 48.4 g, 0.800 mol) and aqueous hydrochloric acid (37 w/w%, 24 mL, 28.6 g, 0.290 mol) was stirred at ambient temperature for 1 h. MeOH (80 mL) was added and the reddish brown solution was refluxed for 30 min. Hydrazine hydrate (19.4 mL, 20.0 g, 0.400 mol) was added at ambient temperature and the mixture was stirred for 1.5 h at this temperature. The pH was adjusted to 4.5–5.0 with aqueous ammonia (25 w/w%, 12–16 mL) and the resulting suspension was stirred for 1 h at 0 ºC. After filtration, the crude product was recrystallized from MeOH (ca. 200 mL). The colorless crystalline product obtained after filtration (ca. 35 g, adduct of tofisopam with MeOH3) was suspended in water (90 mL) and stirred for 1 h at 75 ºC. After cooling to 0–5 ºC, the crystalline product was filtered to give tofisopam (1, 27.9 g, 73%) as colorless crystals, mp 157–158 ºC.1,3a
References
1. Tofisopam official monograph, Japanese Pharmacopoeia, Sixteenth Edition, p. 1511.
2. (a) E. Berényi and G. Blaskó, Acta Pharm. Hung., 1993, 63, 45; (b) E. Horváth, M. Palkovits, Zs. Lenkei, K. Gyüre, M. I. K. Fekete, and P. Arányi, Mol. Brain. Res., 1994, 22, 211; CrossRef (c) E. J. Horváth, K. Horváth, T. Hámori, M. I. K. Fekete, S. Sólyom, and M. Palkovits, Prog. Neurobiol., 2000, 60, 309. CrossRef
3. (a) J. Körösi and T. Láng, Chem. Ber., 1974, 107, 3883; CrossRef (b) J. Körösi, T. Láng, E. Komlós, and L. Erdélyi, Hungarian Pat. 1966, HU 155572 (Chem. Abstr., 1969, 70, 115026).
4. F. Beniczky, T. Papp, I. Simonyi, Gy. Drozdik, T. Kékesi, and I. Derczbach, Hungarian Pat. 1981, HU 191225 (Chem. Abstr., 1984, 100, 85407).
5. R. D. Haworth and C. R. Mavin, J. Chem. Soc., 1931, 1363. CrossRef
6. (a) A. Müller, M. Raltschewa, and M. Papp, Ber., 1942, 75, 692; (b) E. N. Alesso, G. Y. Moltrasio Iglesias, J. D. Mufato, and J. M. Aguirre, Aust. J. Chem., 1992, 45, 1753. CrossRef
7. (a) J. MacMillan, I. L. Martin, and D. J. Morris, Tetrahedron, 1969, 25, 905; CrossRef (b) E. Alesso, R. Torviso, B. Lantano, M. Erlich, L. M. Finkielsztein, L. M. G. Moltrasio, J. M. Aguirre, and E. Brunet, ARKIVOC, 2003, (X), 283; CrossRef (c) B. Lantano, J. M. Aguirre, E. A. Ugliarolo, M. L. Benegas, and G. Y. Moltrasio, Tetrahedron, 2008, 64, 4090; CrossRef (d) V. V. Kouznetsov and D. R. Merchan Arenas, Tetrahedron Lett., 2009, 50, 1546. CrossRef
8. (a) A. Müller and M Hartai, Ber., 1942, 75, 891; (b) W. E. Doering, and J. A. Berson, J. Am. Chem. Soc., 1950, 72, 1118; CrossRef (c) A. Müller, M. Lempert-Sréter, and M. Karczag-Wilhelms, J. Org. Chem., 1954, 19, 1533; CrossRef (d) J. Körösi, G. Szabó-Czibula, and A. Lay-Kónya, Hungarian Pat. 1969, HU 158091 (Chem. Abstr., 1971, 74, 42168).
9. T. Balogh, T. Láng, J. Körösi, G. Zólyomi, P. Botka, T. Hámori, Gy. Somogyi, and T. Láng, Hungarian Pat. 1984, HU 194529 (Chem. Abstr., 1986, 105, 152712).
10. A. Jończyk, B. Serafin, and M. Makosza, Tetrahedron Lett., 1971, 18, 1351. CrossRef
11. F. Gatta, D. Piazza, M. R. Del Giudice, and M. Massotti, Il Farmaco Ed. Sci., 1985, 40, 942.
12. K. Grasser, L. Petöcz, J. Rákóczi, I. Beck, I. Kosóczky, and J. Csöndes, Hungarian Pat. 1978, HU 187161 (Chem. Abstr., 1984, 101, 90584).
13. (a) Zh.-T. Du, J. Lu, H.-R. Yu, Y. Xu, and A.-P. Li, J. Chem. Res., 2010, 34, 222; CrossRef (b) B. Huras, A. Rzewnicka, M. Czajka, J. Zakrzewski, and J. Kazimierczak, Pol. J. Appl. Chem., 2003, 47, 147.
14. Gy. Lukács, unpublished results.
15. (a) A. P. Venkov and I. I. Ivanov, Synth. Commun., 1994, 24, 1145; CrossRef (b) M. Munchhof and A. I. Meyers, J. Org. Chem., 1995, 60, 7086; CrossRef (c) M. Zappala, A. Pellicano, N. Micale, F. S. Menniti, G. Ferreri, G. De Sarro, S. Grasso, and C. De Micheli, Bioorg. Med. Chem. Lett., 2006, 16, 167; CrossRef (d) M. Zappala, G. Postorino, N. Micale, S. Caccamese, N. Parrinello, G. Grazioso, G. Roda, F. S. Menniti, G. De Sarro, and S. Grasso, J. Med. Chem., 2006, 49, 575. CrossRef
16. For a similar strategy for the synthesis of polyalkoxy substituted congested benzophenone derivatives, see: (a) B. L. Chenard, T. W. Butler, F. S. Menniti, M. A. Prochniak, and K. E. G. Richter, Bioorg. Med. Chem. Lett., 1993, 3, 1991; CrossRef (b) T. Ukita, Y. Nakamura, A. Kubo, Y. Yamamoto, Y. Moritani, K. Saruta, T. Higashijima, J. Kotera, M. Takagi, K. Kikkawa, and K. Omori, J. Med. Chem., 2001, 44, 2204; CrossRef (c) A. S. Capilla, M. D. Romero, D. H. Caignard, and P. Renard, Tetrahedron, 2001, 57, 8297; CrossRef (d) S. Lebrun, A. Couture, E. Deniau, and P. Grandclaudon, Org. Biomol. Chem., 2003, 1, 1701. CrossRef
17. H. W. Gschwend and H. R. Rodriguez, Org. React., 1979, 26, 1.
18. (a) E. Molnárné Samu and Gy. Simig, PCT Internat. Pat. Appl. 2004, WO 2004054952 (Chem. Abstr., 2004, 141, 71348); (b) E. Molnárné Samu, Gy. Simig, P. Vágó, and Z. Greff, PCT Internat. Pat. Appl. 2003, WO 2003050092 (Chem. Abstr., 2003, 139, 53044).
19. V. G. Brovchenko, B. B. Paidak, and E. V. Kuznetsov, Khim. Geterotsikl. Soed., 1988, 1, 134.
20. S. Nahm and S. M. Weinreb, Tetrahedron Lett., 1981, 22, 3815. CrossRef
21. For the preparation of N,N-dimethyl-3,4-dimethoxybenzamide, see: M. A. Matulenko and A. I. Meyers, J. Org. Chem., 1996, 61, 573. At our laboratory, thionyl chloride was used instead of oxalyl chloride. CrossRef