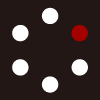
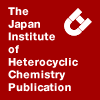
HETEROCYCLES
An International Journal for Reviews and Communications in Heterocyclic ChemistryWeb Edition ISSN: 1881-0942
Published online by The Japan Institute of Heterocyclic Chemistry
e-Journal
Full Text HTML
Received, 30th August, 2013, Accepted, 31st October, 2013, Published online, 8th November, 2013.
DOI: 10.3987/COM-13-S(S)115
■ Acid-Promoted Aromatization of Perylene-Based Endoxides
Alejandro Criado, Manuel Vilas-Varela, Agustín Cobas, Dolores Pérez, Diego Peña, and Enrique Guitián*
Departamento de Química Orgánica, Facultad de Química, Universidad de Santiago de Compostela, E-15706 Santiago de Compostela, Spain
Abstract
The aromatization of perylene-based endoxides by treatment with HCl and EtOH has been studied.We have recently reported the synthesis of benzo[a]perylene (4) as shown in Scheme 1.1 The reaction of 1 with benzyne (2) triggers a tandem domino Diels-Alder sequence to afford adduct 3 in excellent yield and high stereoselectivity, which by treatment with HCl/EtOH afforded 4 in 72% yield. Following the same protocol we have prepared several analogues of 3 and the corresponding perylene-derivatives.
In that publication we focused our attention on the synthetic strategy and on the properties of perylene-derivatives, without a detailed mechanistic discussion. Only some details of the computational study of the transformation of 1 into endoxide 3 were included. However the transformation of endoxide 3 into perylene 4 is far from trivial. We will discuss here some mechanistic aspects of this reaction, but before treating the above transformation we will outline the more reasonable mechanisms for the acid-promoted aromatization of 1,4-endoxides.
Acid-promoted aromatization of cyclohexene-1,4-endoxides such as I is usually an easy process.2-9 It involves protonation to II and a formal elimination of a proton and water to afford the aromatic compound III. The situation for cyclohexadiene-1,4-endoxides IV is more complex. After the opening of the endoxide to the carbenium ion V, there is no hydrogen available to accomplish the elimination. A formal reduction of V to VI must occur to allow dehydration to III (Scheme 2). One-pot procedures with combinations of an acid and a reducing agent, such as HOAc/Zn,10,11 HOAc/Fe,11 TFA/NaBH4,12 TiCl4/LiAlH4,13-15 and TiCl4/Zn,16 or reagents such as Fe2(CO)917,18 or TMSI19 have been used to perform this transformation. Alternatively, cyclohexadiene-1,4-endoxides IV have been transformed into arenes III in a two-operation process, involving a first reduction step to give I, followed by protonation and elimination of water.20-22
According to the above considerations acid-promoted opening of 3 could afford carbenium ions 5 and 6, which could lose water to afford endoxides 7 and 8, respectively. From these, a second protonation/opening sequence would afford carbenium ions 9 and 10, respectively (Scheme 3). DFT calculations at the B3LYP/6-31G(d) level show that the formation of 9 is thermodynamically favoured by 0.011711 Hartrees (7.3 kcal/mol). In any case, transformation of these compounds into benzo[a]perylene (4) requires a formal reduction and the elimination of water.
In our case, having used only HCl and EtOH as reagents for the transformation of 3 into 4, we thought that EtOH should be the actual reducing agent. In fact, though it is not usually considered as a reducing agent, EtOH acts in this way against strong oxidants and also in biological transformations, such as the reduction of NAD+ in liver, catalyzed by alcohol dehydrogenase.23 Closer to our transformation, the reduction of triphenylmethyl cation (11) by hydride transfer from ethanol has been reported in the literature (Scheme 4).24-26
The study of these reactions using HCl/CH3CD2OH had shown that the incorporation of deuterium occurs exclusively at the central atom to give 12. Two alternative mechanisms have been considered: a direct nucleophilic attack of hydride to the carbocation 1124,25 and the addition of ethanol to an ortho-position to give 13, followed by an intramolecular hydride transfer to 12 (Scheme 4).26
Extrapolation of this mechanisms to carbenium ions 9 and 10, led us to predict that the incorporation of deuterium should lead to intermediates 14 and 5, respectively, which after the elimination of DHO should afford non-deuterated benzo[a]pyrene 4 (Scheme 5). In fact, when we treated endoxide 3 with HCl and CH3CD2OH we isolated non-deuterated 4, as proved by NMR analysis.
The transformation of endoxide 3 into benzo[a]perylene (4) has also been achieved using HCl/MeOH or HCl/n-BuOH, but in lower yield.
Finally, we tried the aromatization of compound 16 obtained by a similar tandem cycloaddition. However, when we treated 16 with HCl/EtOH as above a new compound was obtained having a CO band at 1653 cm-1 in its IR spectrum and two singlets at 7.26 (1H) and 6.13 (1H) ppm in its 1H-NMR spectrum. This led us to proposed structure 17 for that compound, which was confirmed by X-ray diffraction studies, as shown in Figure 1.
We firstly considered that the formation of 17 could involve an initial protonation of 16 followed by opening to carbenium ion 18,27 deprotonation to 19 and retro-ene fragmentation to 20 (Scheme 6). Aromatization of the endoxide 20 with HCl/EtOH as above would afford 17.
However, an alternative mechanism for the transformation of 18 into 20, involving a hydride migration to give 21 followed by a fragmentation to give the final ketone, could not be discarded.28 To evaluate the feasibility of these mechanisms we carried out the reaction with DCl/CD3OD, assuming that if the first mechanism were in operation the alcohol 19 would undergo H/D exchange with the medium and the product 20 would incorporate deuterium. On the contrary, if 20 were formed by 1,2-migration of H, deuterium should not be incorporated. After doing the experiment, non-deuterated 17 was isolated, supporting the second mechanistic hypothesis.
In summary, calculations and experimental data for the aromatization of endoxide 3 with HCl/EtOH suggest a mechanism involving the formation of intermediary carbenium ions, its reduction and the elimination of water. A mechanistic proposal involving a hydride donation from the solvent has been discussed.
EXPERIMENTAL
Benzo[a]perylene (4).1 Concentrated HCl solution (50 μL, 37.5% in water) was added to a solution of adduct 3 (50 mg, 0.15 mmol) in EtOH, MeOH or n-BuOH (3 mL), and the mixture was stirred under argon in the absence of light at 78 ºC for 5 h. Then, H2O (1 mL) was added and the mixture was concentrated under reduced pressure. The residue was purified by column chromatography (under argon in the absence of light; SiO2; 9:1 hexane/CH2Cl2), affording benzo[a]perylene derivatives (4). Yield: 72% in EtOH, 32% in MeOH, 31% in n-BuOH.
p-Tolyl(2-(9-(p-tolyl)-7H-benzo[de]anthracen-7-yl)phenyl)metanone (17). Concentrated HCl solution (140 μL, 0.16 mmol, 37.5% in water) was added to a solution of endoxide 16 (42 mg, 0.08 mmol) in EtOH (5 mL), and the mixture was stirred under argon in the absence of light at 78 °C for 4 h. Then, H2O (1 mL) was added and the mixture was concentrated under reduced pressure. The residue was purified by column chromatography (under argon in the absence of light; SiO2; 9:1 hexane/CH2Cl2), affording ketone 17 (30 mg, 75% yield) as a green solid, mp 213-216 ºC; IR 1653 cm-1; 1H NMR (400 MHz, CDCl3) δ 8.13 (d, J = 7.9 Hz, 2H), 7.93 (J = 8.1 Hz, 2H), 7.77 (J = 8.2 Hz, 1H), 7.67 (J = 7.7 Hz, 1H), 7.58-7.50 (m, 2H), 7.48 (d, J = 7.2 Hz, 1H), 7.45-7.42 (m, 1H), 7.41-7.29 (m, 5H), 7.262 (s, 1H), 7.17 (d, J = 8.0 Hz, 2H), 7.15-7.10 (m, 2H), 6.99-6.96 (m, 1H), 6.13 (s, 1H), 2.50 (s, 3H), 2.38 (s, 3H) ppm; 13C NMR (100 MHz, CDCl3), δ 198.2 (C), 147.0 (C), 144.5 (C), 140.1 (C), 139.0 (C), 137.3 (C), 137.1 (C), 137.03 (C), 137.00 (C), 135.4 (C), 133.7 (C), 130.8 (2CH), 130.7 (CH), 130.6 (CH), 130.3 (C), 130.0 (C), 129.4 (2CH), 129.3 (2CH), 128.7 (CH), 127.8 (CH), 127.7 (CH), 127.6 (C), 127.1 (CH), 126.5 (2CH), 126.3 (CH), 126.0 (CH), 125.9 (CH), 125.2 (CH), 124.9 (CH), 123.7 (CH), 118.9 (CH), 44.9 (CH), 21.6 (CH3), 20.9 (CH3) ppm. LRMS (EI), m/z (%): 500 (63, M+), 408 (100), 379 (48); HRMS (EI) calculated for C38H28O: 500.2140, found: 500.2139; X-Ray Diffraction Studies: Deposition number CCDC-942555 for compound 17. Free copies of the data can be obtained via http://www.ccdc.cam.ac.uk/conts/retrieving.html (or from the Cambridge Crystallographic Data Centre, 12 Union Road, Cambridge, CB2 1EZ, UK; Fax: +44 1223 336033; e-mail: deposit@ccdc.cam.ac.uk).
ACKNOWLEDGEMENTS
Financial support from the Spanish Ministry of Economy and Competitiviness (MINECO, CTQ2010-18202, CTQ2010-18208), Xunta de Galicia (10PXIB2200222PR) and FEDER is gratefully acknowledged. Generous allocation of computer time was kindly provided by the Centro de Supercomputación de Galicia (CESGA). A. C. thanks the MEC for the award of a FPU fellowship. We thank Benjamin T. King por helpful discussions.
References
1. A. Criado, D. Peña, A. Cobas, and E. Guitián, Chem. Eur. J., 2010, 16, 9736. CrossRef
2. M. P. Cava and F. M. Scheel, J. Org. Chem., 1967, 32, 1304. CrossRef
3. J. Luo and H. Hart J. Org. Chem., 1987, 52, 3631. CrossRef
4. T. Ghosh and H. Hart, J. Org. Chem., 1989, 54, 5073. CrossRef
5. S. W. Thomas, III, T. M. Long, B. D. Pate, S. R. Kline, E. L. Thomas, and T. M. Swager, J. Am. Chem. Soc., 2005, 127, 17976. CrossRef
6. J. Rybácek, J. Závada, and P. Holy, Synthesis, 2008, 3615. CrossRef
7. H. Qu and C. Chi, Org. Lett., 2010, 12, 3360. CrossRef
8. J. E. Rainbolt and G. P. Miller, J. Org. Chem., 2007, 72, 3020. CrossRef
9. B.-J. Pei, W.-H. Chan, and A. W. M. Lee, J. Org. Chem., 2010, 75, 7332. CrossRef
10. G. Wittig, E. Knauss, and K. Neithammer, Justus Liebigs Ann. Chem., 1960, 630, 10. CrossRef
11. D. Chun, Y. Cheng, and F. Wudl, Angew. Chem. Int. Ed., 2008, 47, 8380. CrossRef
12. G. W. Gribble, W. J. Kelly, and M. P. Sibi, Synthesis, 1982, 143. CrossRef
13. C. H. Wong, C. W. Hung, and H. N. C. Wong, J. Organomet. Chem., 1988, 342, 9. CrossRef
14. B.-J. Pei, W.-H. Chan, and A. W. M. Lee, Org. Lett., 2011, 13, 1774. CrossRef
15. Y.-L. Chen, C.-K. Hau, H. Wang, H. He, M.-S. Wong, and A. W. M. Lee, J. Org. Chem., 2006, 71, 3512. CrossRef
16. D. K. P. Ng, Y.-O.Yeung, W. K. Chan, and S.-C. Yu, Tetrahedron Lett., 1997, 38, 6701. CrossRef
17. W. M. Best, P. A. Collins, R. K. McCulloch, and D. Wege, Aust. J. Chem., 1982, 35, 843. CrossRef
18. J. Basset, M. Romero, T. Serra, and M. D. Pujol, Tetrahedron, 2012, 68, 356. CrossRef
19. J. G. Smith, S. S. Welankiwar, B. S. Shantz, E. H. Lai, and N. G. Chu, J. Org. Chem., 1980, 45, 1817. CrossRef
20. G. Wittig and L. Pohmer, Chem. Ber., 1956, 89, 1334. CrossRef
21. M. S. Newman and J. A. Cella, J. Org. Chem., 1973, 38, 3482. CrossRef
22. H. Hart and D. Ok, J. Org. Chem., 1986, 51, 976. CrossRef
23. H. Theorell and J. S. M. McKee, Nature, 1961, 192, 47. CrossRef
24. P. D. Barlett and J. D. McCollum, J. Am. Chem. Soc., 1956, 78, 1441. CrossRef
25. G. A. Olah and J. J. Svoboda, J. Am. Chem. Soc., 1973, 95, 3794. CrossRef
26. P. Huszthy, K. Lempert, G. Simig, and J. Tamás, J. Chem. Soc., Perkin Trans. 2, 1982, 1671. CrossRef
27. DFT calculations at the B3LYP/6-31G(d) level shown that 18 (-1615.71133 au) is thermodynamically more stable than the cation formed by protonation on the left endoxide (-1615.70731 au).
28. This mechanistic proposal has been suggested by a referee.