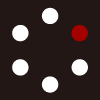
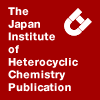
HETEROCYCLES
An International Journal for Reviews and Communications in Heterocyclic ChemistryWeb Edition ISSN: 1881-0942
Published online by The Japan Institute of Heterocyclic Chemistry
e-Journal
Full Text HTML
Received, 28th June, 2013, Accepted, 5th August, 2013, Published online, 14th August, 2013.
DOI: 10.3987/COM-13-S(S)58
■ A Deoxypodophyllotoxin-Based Sensor for Highly Selective Recognition of Hg2+ Ion
Juanjuan Wang, Yuanyuan Yan, and Hui Xu*
Laboratory of Pharmaceutical Design & Synthesis, College of Life Sciences, Northwest A & F University, Xinong Road 22#, Yangling, Shaanxi, 712100, China
Abstract
A new deoxypodophyllotoxin-based probe 1 was prepared. Based on a selective and irreversible Hg2+-promoted desulfurization reaction, compound 1 exhibited high selectivity towards Hg2+ ion over other metal ions, accompanied by transformation of an arylthiocarbamate moiety (green-yellow) to an arylcarbamate one (colorless). The present study may open up a new opportunity for development of natural-product-based probes for selective detection of some specific metal ions.INTRODUCTION
Podophyllotoxin (2, Scheme 1), a naturally occurring cyclolignan, is the main secondary metabolite isolated from the roots and rhizomes of Podophyllum species such as P. hexandrum and P. peltatum. Recently, extensive chemical structural modifications focused on compound 2 as anticancer,1 insecticidal,2 and antifungal3 agents have been conducted. In continuation of our program aimed at the discovery and development of novel podophyllotoxin-based insecticidal agents,4 we fortunately found that a new phenazine derivative of 4-deoxypodophyllotoxin (3)5 exhibited strong fluorescence.
On the other hand, mercury ions can cause great damage to the nervous system of human beings at very low concentrations, and could be bioaccumulated through the food chain, so mercury ions are considered to a highly toxic and dangerous pollutants for human beings.6 Nowadays, it is still very desirable to explore selective and sensitive chemosensors for detection of Hg2+ ion over other heavy and transition metal (HTM) ions. Recently, different kinds of excellent sensors for recognition of Hg2+ ion over other HTM ions have been developed with good performance.7 Moreover, many attractive probes for detection of Hg2+ ion over other HTM ions based upon the specific mercury-promoted desulfurization reaction have also been described.8
Therefore, as part of our ongoing research program in developing selective chemosensors for detection of Hg2+ ion by a specific mercury-promoted desulfurization reaction,9 in this paper, as shown in Scheme 1, we designed and tried to prepare a new thiourea derivative (5) by reaction of 3 with phenylisothiocyanate (4) under microwave irradiation. Interestingly, instead of 5, an unexpected arylthiocarbamate derivative (1) was obtained in a 40% yield. The chemical structure of 1 was well characterized by 1H and 13C NMR spectroscopy, and mass spectrometry (MS).10 To the best of our knowledge, this is the first time to report the natural-product-based probe for recognition of Hg2+ ion based on the specific mercury-promoted desulfurization reaction.
RESULTS AND DISCUSSION
As described in Figure 1, the optical properties of 1 were examined in the absence or presence of various heavy and transition metal species. Compound 1 exhibited an absorption band at 384 nm. The metal species was then added to the solution of 1 respectively. As expected, the other tested metal ions, such as Ca2+, Ni2+, Fe3+, Ba2+, Zn2+, Cd2+, Co2+, Pb2+, Mn2+, Mg2+, K+, Cs+ and Ag+, showed almost no change. Only when Hg2+ ion was added to the solution of 1, a 38 nm red-shift in the wavelength region from 384 to 422 nm was observed.
Figure 2 showed the fluorescent intensity ratio (I0/I) of 1 in the presence of Ca2+, Ni2+, Cs+, Ba2+, Fe3+, Cd2+, Mn2+, Cu2+, Pb2+, Mg2+, Ag+, K+, Co2+, Zn2+, and Hg2+ ions, respectively. It is noteworthy that only when Hg2+ ion was added to the solution of 1, a 14.6-fold decrease of the fluorescent intensity was observed at 517 nm. It was because an arylthiocarbamate moiety of 1 was transformed to an arylcarbamate one in the presence of Hg2+ ion (Scheme 2), accompanied by transformation of a strong fluorescence (green-yellow, inset of Figure 2) to a weak fluorescence (colorless). Whereas the addition of other metal ions such as Ca2+, Ni2+, Cs+, Ba2+, Fe3+, Cd2+, Mn2+, Cu2+, Pb2+, Mg2+, Ag+, K+, Co2+, and Zn2+, did not lead to a significant change of the fluorescent intensity. The evidence for the proposed reaction was demonstrated by 1H and 13C NMR spectra. As depicted in the partial 1H NMR spectra of Figure 3, when 1.2 equiv of Hg2+ was added to the solution of 1, the proton of NH was sharply shifted from 11.7 (1, (a)) to 7.41 ppm (6, (b), protons of benzene ring and NH were overlapped). Similarly, as shown in Figure 4, when 1.2 equiv of Hg2+ was added to the solution of 1, the carbon chemical shift of C=S (arylthiocarbamate, δ: 187.69 (1, (a))) was clearly decreased to 151.19 ppm (C=O, 6, (b)). Solid evidence of the binding mode came from comparing the ESI-MS spectra of 1 and 6. As described in Figure 5, the peak at m/z 528.35 (b) was corresponding to [6+H]+, whereas probe 1 exhibited only a peak at m/z 544.25 (a) corresponding to [1+H]+.
The titration reaction curve of 1 toward the Hg2+ ion was investigated as shown in Figure 6. The fluorescent intensity of 1 gradually decreased in response to the increases in the concentration of the added Hg2+ ion, and the titration reaction curve showed a steady decrease until a plateau was reached when 1.0 equiv of Hg2+ ion was added.
To investigate the utility of 1 as an ion-selective fluorescent sensor for Hg2+ ion, the cross-contamination experiments were conducted in the presence of Hg2+ ion at 2×10-5 M mixed with other metal ions at 2×10-4 M, respectively. It demonstrated that the selectivity of 1 towards Hg2+ ion was almost not affected by other competitive ions such as Ca2+, Ni2+, Cs+, Ba2+, Fe3+, Cd2+, Mn2+, Cu2+, Pb2+, Mg2+, Ag+, K+, Co2+, and Zn2+ (Figure 7).
Additionally, the possible mechanism for synthesis of 1 was also proposed (Scheme 3). The thiourea 5 was firstly synthesized by reaction of 3 with 4 under microwave irradiation. Compound 5 was then transformed to the deoxypodophyllotoxin-derived isothiocyanate (7) by the reversible reaction, which further reacted with ethanol to offer the deoxypodophyllotoxin-derived thiocarbamate (1).
In conclusion, a new and highly Hg2+-selective deoxypodophyllotoxin-like natural-product-based sensor 1 was prepared. Based on the Hg2+-promoted selective desulfurization reaction, probe 1 exhibited high selectivity towards Hg2+ ion over other competitive metal ions, accompanied by transformation of an arylthiocarbamate moiety (green-yellow) to an arylcarbamate one (colorless). It was demonstrated by the 1H, 13C NMR and MS spectroscopy. To the best of our knowledge, it is the first probe based on a natural product moiety (deoxypodophyllotoxin) for recognition of Hg2+ ion. Importantly, it is noteworthy that, due to a broad spectrum of chemical and functional diversity of natural products, this study may open up a new opportunity for development of natural-product-based probes for selective detection of some specific metal ions.
EXPERIMENTAL
All solvents and reagents were used as obtained from commercial sources without further purification. Zn(ClO4)2·6H2O, Fe(ClO4)3·6H2O, Ca(ClO4)2·4H2O, Mg(ClO4)2, Ba(ClO4)2, Pb(ClO4)2, Cd(ClO4)2, Cu(ClO4)2·6H2O, Ni(ClO4)2·6H2O, Mn(ClO4)2·6H2O, KClO4, CsClO4, Co(ClO4)2·6H2O, Hg(ClO4)2 and AgPF4 were investigated as sources for metal ions. Analytical thin-layer chromatography (TLC) and preparative thin-layer chromatography (PTLC) were performed with silica gel plates using silica gel 60 GF254 (Qingdao Haiyang Chemical Co., Ltd.). Microwave irradiation was performed in a CEM Discover Synthesis Unit (CEM Corp., Matthews, NC, made in USA). Nuclear magnetic resonance spectra (NMR) were recorded on a Bruker Avance DMX 400 MHz instrument in DMSO-d6 (1H at 400 MHz and 13C at 100 MHz) using TMS (tetramethylsilane) as the internal standard. Electron impact ionizationmass spectra (EI-MS) were carried out with HP 5988 instrument. Fluorescent spectra were determined on a Hitachi F-4500 spectrophotometer. UV-visible spectra were determined on a Hitachi U-3310 spectrophotometer.
Compound 3 was prepared according to our previous paper.5
Synthesis of compound 1. A mixture of 3 (0.2 mmol) and phenylisothiocyanate (4, 0.3 mmol) in absolute EtOH (2 mL) was placed in a 10-mL glass tube, which was sealed with a septum and placed into the microwave cavity, and reacted at microwave irradiation at 120 oC with an output power of 50 W for 30 min. Then the vessel was allowed to cool to a temperature below 50 oC before removal from the microwave cavity and opening to the atmosphere. The mixture was concentrated and purified by PTLC to give 43.6 mg (40% yield) of 1 as a yellow solid. Mp 167-169 oC; [α]20D = +54 (c 2.5 mg/mL, acetone); 1H NMR (400 MHz, DMSO-d6) δ: 11.70 (s, 1H, NH), 7.68-8.05 (m, 3H), 7.36 (s, 1H), 6.94 (s, 1H), 6.89 (s, 1H), 6.64 (s, 1H), 6.00 (s, 2H, OCH2O), 4.87 (d, J = 4.4 Hz, 1H, H-1), 4.63 (s, 2H, CH2CH3), 4.39-4.43 (m, 1H, H-11), 3.99-4.04 (m, 4H, 3′-OCH3, H-11), 3.22-3.27 (m, 1H, H-4), 3.08-3.11 (m, 1H, H-2), 2.69-2.84 (m, 2H, H-3, 4), 1.40 (t, J = 6.8 Hz, 3H, CH2CH3); 13C NMR (100 MHz, DMSO-d6) δ: 187.7, 175.2, 153.3, 146.7, 146.2, 145.1, 142.2, 142.0, 140.6, 135.7, 131.6, 130.1, 129.4, 128.8, 121.9, 117.0, 111.5, 110.1, 108.7, 101.1, 71.8, 65.1, 55.9, 46.0, 43.7, 32.7, 32.0, 30.1, 14.2; MS (EI), m/z (%) 544.25 ([M+H]+, 100).
ACKNOWLEDGEMENTS
This work was supported by National Natural Science Foundation of China (No. 31171896), and the Special Funds of Central Colleges Basic Scientific Research Operating Expenses (YQ2013008). We also thank to Prof. H. L. Zhang for the NMR experiments.
References
1. (a) Y. J. You, Curr. Pharm. Des., 2005, 11, 1695; CrossRef (b) H. Xu, M. Lv, and X. Tian, Curr. Med. Chem., 2009, 16, 327. CrossRef
2. (a) M. Miyazawa, M. Fukuyama, K. Yoshio, T. Kato, and Y. Ishikawa, J. Agric. Food Chem., 1999, 47, 5108; CrossRef (b) Y. Inamori, M. Kubo, Y. Kato, H. Tsujibo, M. Sakai, and M. Kozawa, Chem. Pharm. Bull., 1986, 34, 2542; CrossRef (c) X. D. Di, Y. Q. Liu, Y. Q. Liu, X. Y. Yu, H. Xiao, X. Tian, and R. Gao, Pestic. Biochem. Physiol., 2007, 89, 81. CrossRef
3. K. A. Kumar, S. K. Singh, B. S. Kumar, and M. Doble, Cent. Eur. J. Chem., 2007, 5, 880. CrossRef
4. (a) H. Xu, J. J. Wang, H. J. Sun, M. Lv, X. Tian, X. J. Yao, and X. Zhang, J. Agric. Food Chem., 2009, 57, 7919; CrossRef (b) Y. Wang, Y. H. Shao, Y. Y. Wang, L. L. Fan, X. Yu, X. Y. Zhi, C. Yang, H. Qu, X. J. Yao, and H. Xu, J. Agric. Food Chem., 2012, 60, 8435; CrossRef (c) Y. Guo, Y. Yan, X. Yu, Y. Wang, and H. Xu, J. Agric. Food Chem., 2012, 60, 7016; CrossRef (d) H. Xu, Q. Wang, and Y. Guo, Chem. Eur. J., 2011, 17, 8299. CrossRef
5. J. Wang, X. Yu, X. Zhi, and H. Xu, J. Agric. Food Chem., 2013, 61, 6336. CrossRef
6. (a) H. N. Kim, W. X. Ren, J. S. Kim, and J. Yoon, Chem. Soc. Rev., 2012, 41, 3210; CrossRef (b) H. N. Kim, M. H. Lee, H. J. Kim, J. S. Kim, and J. Yoon, Chem. Soc. Rev., 2008, 37, 1465; CrossRef (c) I. Onyido, A. R. Norris, and E. Buncel, Chem. Rev., 2004, 104, 5911. CrossRef
7. For selected examples see: (a) A. B. Descalzo, R. Martinez-Manez, R. Radeglia, K. Rurack, and J. Soto, J. Am. Chem. Soc., 2003, 125, 3418; CrossRef (b) G. Pelossof, R. Tel-Vered, X. Q. Liu, and I. Willner, Chem. Eur. J., 2011, 17, 8904; CrossRef (c) T. Kang, S. M. Yoo, I. Yoon, S. Lee, J. Choo, S. Y. Lee, and B. Kim, Chem. Eur. J., 2011, 17, 2211; CrossRef (d) B. Tang, B. Y. Ding, K. H. Xu, and L. L. Tong, Chem. Eur. J., 2009, 15, 3147; CrossRef (e) X. F. Guo, X. H. Qian, and L. H. Jia, J. Am. Chem. Soc., 2004, 126, 2272; CrossRef (f) Z. Guo, W. Zhu, M. Zhu, X. Wu, and H. Tian, Chem. Eur. J., 2010, 16, 14424; CrossRef (g) H. N. Kim, W. X. Ren, and J. S. Kim, Chem. Soc. Rev., 2004, 41, 3210; CrossRef (h) H. N. Kim, S. Nam, K. M. K. Swamy, Y. Jin, X. Chen, Y. Kim, S. Kim, S. Park, and J. Yoon, Analyst, 2011, 136, 1339. CrossRef
8. For selected examples see: (a) M. H. Lee, S. W. Lee, S. H. Kim, C. Kang, and J. S. Kim, Org. Lett., 2009, 11, 2101; CrossRef (b) M. H. Lee, B.-K. Cho, J. Yoon, and J. S. Kim, Org. Lett., 2007, 9, 4515; CrossRef (c) J.-S. Wu, I.-C. Hwang, K. S. Kim, and J. S. Kim, Org. Lett., 2007, 9, 907; CrossRef (d) M. G. Choi, Y. H. Kim, J. E. Namgoong, and S.-K. Chang, Chem. Commun., 2009, 3560; CrossRef (e) W. Jiang and W. Wang, Chem. Commun., 2009, 3913; CrossRef (f) X. Zhang, Y. Xiao, and X. Qian, Angew. Chem. Int. Ed., 2008, 47, 8025; CrossRef (g) X. Cheng, S. Li, H. Jia, A. Zhong, C. Zhong, J. Feng, J. Qin, and Z. Li, Chem. Eur. J., 2012, 18, 1691; CrossRef (h) X. Cheng, Q. Li, C. Li, J. Qin, and Z. Li, Chem. Eur. J., 2011, 17, 7276. CrossRef
9. (a) H. L. Dai and H. Xu, Bioorg. Med. Chem. Lett., 2011, 21, 5141; CrossRef (b) Y. Yan, Z. Che, X. Yu, X. Zhi, J. Wang, and H. Xu, Bioorg. Med. Chem., 2013, 21, 508; CrossRef (c) H. L. Dai, Y. Y. Yan, Y. Guo, L. L. Fan, Z. P. Che, and H. Xu, Chem. Eur. J., 2012, 18, 11188; CrossRef (d) Y. Yan, Y. Zhang, and H. Xu, ChemPlusChem., 2013, 78, 628. CrossRef