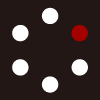
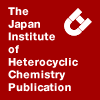
HETEROCYCLES
An International Journal for Reviews and Communications in Heterocyclic ChemistryWeb Edition ISSN: 1881-0942
Published online by The Japan Institute of Heterocyclic Chemistry
e-Journal
Full Text HTML
Received, 26th June, 2013, Accepted, 20th August, 2013, Published online, 28th August, 2013.
DOI: 10.3987/COM-13-S(S)51
■ Synthesis of 1,4-Disubstituted-1,2,3-trizazoles via Click Reaction in Micro Flow Reactor
Ming Lei,* Ruijun Hu, Yanguang Wang, and Hong Zhang
Department of Chemistry, Zhejiang University , 38 Zheda Road, Hangzhou, 310027, China
Abstract
A Cu (I)-catalyzed three-component click reaction of alkyl bromide, terminal alkyne and sodium azide was developed in micro flow reactor. Sodium ascorbate was added as additive to prevent the oxidative coupling of desired product with terminal alkyne. Under optimized condition, various 1,4-disubstituted-1,2,3-triazoles were obtained in 85-95% yield with exclusive 1,4-regioisomeric selectivity. The present smooth procedure greatly accelerated the reaction due to the excellent mixing and mass transfer in micro flow system.The Huisgen 1,3-dipolar cycloaddition of azides and alkynes,1 one of the most powerful click reactions which resulting in 1,2,3-triazoles has been widely used in various research areas, ranging from bioorganic and medicinal chemistry to materials science for its remarkable efficiency. The recently discovered copper catalyzed (CuAAC) version,2 which accelerates the reaction up to 107 times, has placed it in a class of its own and has enabled many novel applications ranging from drug discovery to surface science where rapid and reliable bond formation is required. In this fashion, a number of peptide- and protein-based bioconjugates have been successfully prepared.3 Moreover, one pot three-component procedure avoids isolation and handling of potentially unstable organic azides, as they are generated in situ, making this already powerful click process even more user-friendly and safe.4 Besides the advantages of its high efficiency, regioselectivity, and compatibility with reaction conditions, the heterocyclic moiety of 1,4-disubstituted 1,2,3-triazoles is stable under in vivo conditions.5 The triazole linker also introduces some degree of polarity into the tracer, adding a 1,2,3-triazole group is expected to be advantageous with regard to the pharmacological properties of the resulting radiotracer molecules. As a result of ongoing improvements in 1,3-dipolar cycloaddition chemistry and because of its versatility and short reaction times, “click” radiochemistry promises to become a widely used tool for preparation of radiotracers. Subsequently, this approach has been applied for radiolabeling of different substrates with [18F]-fluorine and other radioisotopes.6
Combinatorial chemistry as a high-throughput synthetic technology has became a powerful tool for the discovery of new drugs and increasing interests have been drawn onto the application of microfluidic chips in this field.7 Automated microreactor-based (microfluidic chip) continuous flow systems have the potential to greatly accelerate the production of small molecule libraries of drug-like structures (primarily heterocycles).8 Our previous work had demonstrated the use of microfluidic based reactors could eliminate the formation of the corresponding byproduct and ensure the desired transformation with very high selectivity and efficiency.9 In comparison with the classical batch systems, especially for the three-component cascade approach, the micro flow technique enhances accurate temperature control and efficient mixing. As part of our ongoing research to develop new synthetic methods for the generation of heterocycle libraries and radiolabelling imaging probes based on microfluidic reactor, we herein report the micro flow synthesis of 1,4-disubstituted 1,2,3-triazoles derivatives from corresponding alkyl bromides, sodium azide, and alkynes.
Our reactor is a microfluidic chip fabricated in soda-lime glass with channels produced by standard photolithography and wet etching techniques.10 The internal geometry of the micro flow reactor is 500 µm × 100 µm × 1000 mm. The structure of the reactor is illustrated in Scheme 1. PTFE tubing (inner diameter ID = 0.9 mm) was fitted to the inlet and outlet hole directly. The end of inlet tube was connected to a Harvard PHD 2000 syringe pump and the end of outlet tube was connected to a sample vial, which was used to collect the final solution.
We first investigated adapting the model reaction into a continuous micro flow process for the synthesis of 1-benzyl-4-phenyl-1H-1,2,3-triazole (3a) using benzyl bromide (1a), phenylacetylene (2a) and sodium azide in the presence of CuI and sodium ascorbate at different flow rates and temperatures. Herein, sodium ascorbate was used as reducing agent to eliminate the formation of oxidative coupling byproduct.2a,2k Preliminary test were performed at 50 oC. The acetonitrile solution of benzyl bromide (0.03 M) mixed with phenylacetylene (0.03 M) and the aqueous solution containing sodium azide (0.045 M), sodium ascorbate (0.03 M), and CuI (0.003 M) were introduced respectively through syringe pump at identical flow rates changed from 5 μL/min to 15 μL/min. The results are summarized in Table 1. When the flow rates were respectively set up at 5, 10, 15 μL/min, the yields of product increased from 80% to 94% with the increase of flow rate (Table 1, entries 1–3). At 65 oC, when flow rates set up at 5, 10, 15 μL/min respectively, the reactions afforded the product with yields between 91% and 95% (Table 1, entries 4–6). While, further increasing the flow rate to 20 μL/min led slight decrease of yield (Table 1, entry 7). At 70 oC, significant drop of yields were observed at same flow rates (Table 1, entries 8–10). In general, at 65 oC and 15 μL/min flow rate, the reaction could afford best yield.
Subsequently, a series of 1,4-disubstituted 1,2,3-triazole derivatives were synthesized to investigate the scope and limitation of this reaction using the optimized micro flow procedure. As shown in Scheme 2, various alkyl bromides and alkynes could afford corresponding products in good to excellent yields with exclusive 1,4-regioisomeric selectivity. Benzyl bromides bearing electron-donating groups are more reactive than those bearing electron-withdrawing group. It is noteworthy that in the present micro flow reactor the reactions completed smoothly within several minutes. Moreover, by-product was not observed in all cases taking the advantage of enhanced mass transfer.
In conclusion, we have successfully developed a rapid and efficient process for the synthesis of 1,4-disubstituted 1,2,3-triazole derivatives using micro flow reactor. Under the optimized conditions, a series of 1,4-disubstituted 1,2,3-triazole derivatives were synthesized in 85–95% isolated yields. This process was also applied to similar reactions using aliphatic alkynes as the substrate. Compared with the classical batch systems, the present smooth procedure greatly accelerated the reaction due to the excellent mixing and mass transfer in micro flow system. These advances will facilitate the rapid synthesis of biologically compounds library and radio labeled imaging probes. Further exploration of the reactivity features of this methodology and applications to the synthesis of complex molecules and the drug discovery process are in progress.
EXPERIMENTAL
All chemicals were reagent grade and used as purchased without further purification unless otherwise stated. Column chromatography was performed using silica gel 60 (300-400 mesh). Melting points were obtained on a YANACO micro melting point apparatus and are uncorrected. 1HNMR spectra were recorded in CDCl3 using TMS as an internal reference with a Bruker Avance-400 spectrometer operating at 400 MHz.
Typical Procedure for the Synthesis of 1,4-Disubstituted 1,2,3-Triazoles in Microfluidic Chip.
1-Benzyl-4-phenyl-1H-1,2,3-triazole (3a). Acetonitrile solution (3 mL) of benzyl bromide (0.03 M) mixed with phenylacetylene (0.03 M) and the aqueous solution (3 mL) containing sodium azide (0.045 M), sodium ascorbate (0.03 M), and CuI (0.003 M) were transferred into separate gas-tight syringes. The syringes were placed in a Harvard PHD 2000 syringe pump which was set to deliver the reactants at identical flow rate of 15 μL/min. The output mixture was collected and then concentrated under vacuum. The residue was subjected to silica gel column chromatography with Pet–EA (2:1) as eluent to give 3a (20 mg, 95%); mp 128–129 ˚C (lit.,4c mp 129–129.5 ˚C); 1H NMR (400 MHz, CDCl3) δ = 5.58 (s, 2 H), 7.30–7.41 (m, 6 H), 7.68 (s, 1 H), 7.80–7.82 (m, 4 H).
1-(4-Bromobenzyl)-4-phenyl-1H-1,2,3-triazole (3b): mp 152–153 ˚C (lit.,4c mp 152–152.5 ˚C); 1H NMR δ = 5.53 (s, 2 H), 7.17 (d, J = 8.4 Hz, 2 H), 7.22–7.41 (m, 3 H), 7.51 (d, J = 8.4 Hz, 2 H), 7.67 (s, 1 H), 7.80 (d, J = 8.4 Hz, 2 H).
1-(4-Nitrobenzyl)-4-phenyl-1H-1,2,3-triazole (3c): mp 155–157 ˚C (lit.,4c mp 156–157 ˚C); 1H NMR δ = 5.65 (s, 2 H), 7.33–7.37 (m, 3 H), 7.42 (d, J = 8.8 Hz, 2 H), 7.77 (s, 1 H), 7. 79 (d, J = 8.5 Hz, 2 H), 8.21 (dd, J = 8.8, 1.9 Hz, 2 H).
1-(4-Methylbenzyl)-4-phenyl-1H-1,2,3-triazole (3d): mp 110–112 ˚C (lit.,4c mp 110 ˚C); 1H NMR δ = 2.38 (s, 3 H), 5.55 (s, 2 H), 7.28–7.43 (m, 7 H), 7.65 (s, 1 H), 7.80–7.82 (m, 2 H).
4-Phenyl-1-(2-phenylethyl)-1H-1,2,3-triazole (3e): mp 138–139 ˚C (lit.,4c mp 139 ˚C); 1H NMR δ = 3.24 (t, J = 7.2 Hz, 2 H), 4.63 (t, J = 7.2 Hz, 8 H), 7.15 (d, J = 7.6 Hz, 2 H), 7.26–7.44 (m, 6 H), 7.47 (s, 1 H), 7.66 (t, J = 8.1 Hz, 2 H), 7.78 (d, J = 7.1 Hz, 2 H), 7.88 (d, J = 7.6 Hz, 2 H).
2-(1-Benzyl-1H-1,2,3-triazol-4-yl)-2-propanol (3f): mp 77–78 ˚C (lit.,4c mp 77 ˚C); 1H NMR δ = 1.57 (s, 6 H), 3.19 (s, 1H), 5.43 (s, 2 H), 7.21–7.36 (m, 5 H), 7.39 (s, 1 H).
(1-Benzyl-1H-1,2,3-triazol-4-yl)methanol (3g): mp 78–79 ˚C (lit.,4c mp 78–78.5 ˚C); 1H NMR δ = 4.65 (s, 2 H), 5.41 (s, 2 H), 7.18–7.29 (m, 5 H), 7.45 (s, 1 H).
1-Benzyl-4-methoxycarbonyl-1,2,3-triazole (3h): mp 104–106 ˚C (lit.,4f mp 104–107 ˚C); 1H NMR δ = 3.81 (s, 3 H), 5.65 (s, 2 H), 7.40–7.42 (m, 5 H), 8.90 (s, 1 H).
1-Benzyl-4-butyl-1H-1,2,3-triazole (3i):4c oil; 1H NMR δ = 0.81 (t, J = 7.0 Hz, 3 H), 1.18–1.56 (m, 4 H), 2.35–2.63 (m, 2 H), 5.38 (s, 2 H), 7.02–7.26 (m, 5 H), 7.38 (s, 1 H).
Ethyl 2-(4-Phenyl-1H-1,2,3-triazol-1-yl)acetate (3j): mp 93–94 ˚C (lit.,2h mp 94–95 ˚C); 1H NMR δ = 1.32 (t, J = 7.2 Hz, 3 H ), 4.31 (q, J = 7.2 Hz, 2 H), 5.22 (s, 2 H), 7.25–7.90 (m, 5 H), 7.91 (s, 1 H).
1-Octyl-4-phenyl-1H-1,2,3-triazole (3k): mp 77–78 ˚C (lit.,4f mp 78–79 ˚C); 1H NMR δ = 0.86 (t, J = 7.1 Hz, 3 H), 1.15–1.23 (m, 10 H), 1.89 (t, J = 7.1 Hz, 2 H), 4.42 (t, J = 7.1 Hz, 2 H), 7.35 (m, 1 H), 7.47 (m, 2 H), 7.86 (m, 2 H), 8.58 (s, 1 H).
4-Methoxycarbonyl-1-octyl-1H-1,2,3-triazole (3l): mp 78–80 ˚C (lit.,4f mp 78–80 ˚C); 1H NMR δ = 0.87 (t, J = 7.2 Hz, 3 H), 1.15–1.22 (m, 10 H), 1.89 (m, 2 H), 3.83 (s, 3 H), 4.43 (t, J = 7.2 Hz, 2 H), 8.81 (s, 1 H).
ACKNOWLEDGEMENTS
Financial support of this work from National Science and Technology Support Program (No. 2012BAI13B06) is gratefully acknowledged.
References
1. R. Huisgen In 1,3-dipolar cycloaddition chemistry; ed. by A. Padwa; Wiley: New York, 1984.
2. (a) V. V. Rostovstev, L. G. Green, V. V. Fokin, and K. B. Sharpless, Angew. Chem. Int. Ed., 2002, 41, 2596; CrossRef (b) V. O. Rodionov, V. V. Fokin, and M. G. Finn, Angew. Chem. Int. Ed., 2005, 44, 2210; CrossRef (c) B. H. Lipshutz and B. R. Taft, Angew. Chem. Int. Ed., 2006, 45, 8235; CrossRef (d) S. Díez-González, A. Correa, L. Cavallo, and S. P. Nolan, Chem. Eur. J., 2006, 12, 7558; CrossRef (e) T. R. Chan, R. Hilgraf, K. B. Sharpless, and V. V. Fokin, Org. Lett., 2004, 6, 2853; CrossRef (f) C. Girard, E. Önen, M. Aufort, S. Beauvière, E. Samson, and J. Herscovici, Org. Lett., 2006, 8, 1689; CrossRef (g) S. Chassaing, M. Kumarraja, A. S. S. Sido, P. Pale, and J. Sommer, Org. Lett., 2007, 9, 883; CrossRef (h) F. Alonso, Y. Moglie, G. Radivoy, and M. Yus, Eur. J. Org. Chem., 2010, 1875; (i) C. Z. Tao, X. Cui, J. Li, A. X. Liu, L. Liu, and Q. X. Guo, Tetrahedron Lett., 2007, 48, 3525; CrossRef (j) I. S. Park, M. S. Kwon, Y. Kim, J. S. Lee, and J. Park, Org. Lett., 2008, 10, 497; CrossRef (k) J. E. Hein and V. V. Fokin, Chem. Soc. Rev., 2010, 39, 1302. CrossRef
3. (a) A. Devadoss and C. E. D. Chidsey, J. Am. Chem. Soc., 2007, 129, 5370; CrossRef (b) L. V. Lee, M. L. Mitchell, S. J. Huang, V. V. Fokin, K. B. Sharpless, and C. H. Wong, J. Am. Chem. Soc., 2003, 125, 9588; CrossRef (c) T. S. Seo, Z. Li, H. Ruparel, and J. Ju, J. Org. Chem., 2003, 68, 609; CrossRef (d) Q. Wang, T. R. Chan, R. Hilgraf, V. V. Fokin, K. B. Sharpless, and M. G. Finn, J. Am. Chem. Soc., 2003, 125, 3192; CrossRef (e) H. Struthers, B. Spingler, T. L. Mindt, and R. Schibli, Chem. Eur. J., 2008, 14, 6173; CrossRef (f) J. Shen, R. Woodward, J. P. Kedenburg, X. W. Liu, M. Chen, L. Y. Fang, D. X. Sun, and P. G. Wang, J. Med. Chem., 2008, 51, 7417; CrossRef (g) J. F. Lutz and Z. Zarafshani, Adv. Drug Delivery Rev., 2008, 60, 958. CrossRef
4. (a) P. Appukkuttan, W. Dehaen, V. V. Fokin, and E. V. der Eycken, Org. Lett., 2004, 6, 4223; CrossRef (b) A. K. Feldman, B. Colasson, and V. V. Fokin, Org. Lett., 2004, 6, 3897; CrossRef (c) H. Sharghi, R. Khalifeh, and M. M. Doroodmanda, Adv. Synth. Catal., 2009, 351, 207; CrossRef (d) D. Yang, N. Fu, Z. L. Liu, Y. Li, and B. H. Chen, Synlett, 2007, 278; CrossRef (e) D. Kumar and V. B. Reddy, Synthesis, 2010, 1687; CrossRef (f) K. Kacprzak, Synlett, 2005, 943; CrossRef (g) A. R. Bogdan and N. W. Sach, Adv. Synth. Catal., 2009, 351, 849; CrossRef (h) F. Alonso, Y. Moglie, G. Radivoy, and M. Yusa, Adv. Synth. Catal., 2010, 352, 3208. CrossRef
5. B. H. M. Kuijpers, S. Groothuys, A. C. Soede, P. Laverman, O. C. Boerman, F. L. van Delft, and F. P. J. T. Rutjes, Bioconjugate. Chem., 2007, 18, 1847. CrossRef
6. (a) U. Sirion, H. J. Kim, J. H. Lee, J. W. Seo, B. S. Lee, S. J. Lee, S. J. Oh, and D. Y. Chi, Tetrahedron Lett., 2007, 48, 3953; CrossRef (b) M. Glaser and E. G. Robins, J. Labelled Compd. Radiopharm., 2009, 52, 407; CrossRef (c) C. Mamat, T. Ramenda, and F. R. Wuest, Mini-Rev. Org. Chem., 2009, 6, 21; CrossRef (d) M. Lei, M. Tian, and H. Zhang, Curr. Med. Imaging Rev., 2012, 8, 302. CrossRef
7. (a) L. Kang, B. G. Chung, R. A. Langer, and A. Khademhosseini, Drug Discovery Today, 2008, 13, 1; CrossRef (b) C. O. Kappe and D. Dallinger, Nature Rev. Drug Discovery, 2006, 5, 51; CrossRef (c) C. Wiles and P. Watts, Expert Opin. Drug Discovery, 2007, 11, 1487. CrossRef
8. (a) B. P. Mason, K. E. Price, J. L. Steinbacher, A. R. Bogdan, and D. T. McQuade, Chem. Rev., 2007, 107, 2300; CrossRef (b) I. R. Baxendale, J. J. Hayward, S. Lanners, S. V. Ley, and C. D. Smith, 'Microreactors in Organic Synthesis and Catalysis,' ed. by T. Wirth, Wiley-VCH, Weinheim, 2008; (c) W. Ehrfeld, V. Hessel, and H. Lowe, 'Microreactors: New Technology for Modern Chemistry,' Wiley, Weinheim, 2000; CrossRef (d) C. M. Griffiths-Jones, M. D. Hopkin, D. Jönsson, S. V. Ley, D. J. Tapolczay, E. Vickerstaffe, and M. Ladlow, J. Comb. Chem., 2007, 9, 422. CrossRef
9. (a) R. J. Hu, M. Lei, H. S. Xiong, X. Mu, Y. G. Wang, and X. F. Yin, Tetrahedron Lett., 2008, 49, 387; CrossRef (b) M. Lei, W. Tian, R. J. Hu, W. Li, and H. Zhang, Synthesis, 2012, 44, 2519; CrossRef (c) M. Lei, W. Li, R. J. Hu, W. Tian, Y. G. Wang, and H. Zhang, Heterocycles, 2012, 86, 777. CrossRef
10. J. Gao, X. F. Yin, and Z. L. Fang, Lab Chip, 2004, 4, 47. CrossRef