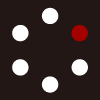
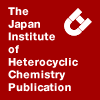
HETEROCYCLES
An International Journal for Reviews and Communications in Heterocyclic ChemistryWeb Edition ISSN: 1881-0942
Published online by The Japan Institute of Heterocyclic Chemistry
e-Journal
Full Text HTML
Received, 1st July, 2013, Accepted, 26th July, 2013, Published online, 5th August, 2013.
DOI: 10.3987/COM-13-S(S)77
■ Potential DNA Bis-Intercalating Agents. Synthesis and Antitumor Activity of N,N'-(Methylenedi-4,1-cyclohexanediyl-bis(9-acridinamine) Isomers
Gordon W. Gribble,* Michael D. Mosher, Gary D. Jaycox, Michael Cory, and Terri A. Fairley
6128 Burke Laboratory, Department of Chemistry, Dartmouth College, Hanover, New Hampshire 03755, U.S.A.
Abstract
We describe the synthesis and antitumor activity of three novel isomeric bis-acridines 14-16 possessing a non-aromatic, semi-rigid dicyclohexylmethylene tether.INTRODUCTION
For several decades there has been considerable interest in the design, synthesis, and biological evaluation of potential DNA polyintercalators for the treatment of cancer and other diseases.1,2 A prototypical compound is ditercalinium (1), which has entered clinical trials as an anticancer agent.3 Moreover, nature has contributed a set of naturally occurring DNA bis-intercalators, the quinoxaline antitumor antibiotics, typified by echinomycin (2).4 The planar acridine ring system is an excellent DNA intercalator and many investigations feature acridines and bis-acridines as potential anticancer and other agents.5,6 At least one acridine drug, amsacrine (3), is used to treat human cancer.7
In our previous work we described the synthesis and preliminary antitumor activity of a series of novel, conformationally restricted bis(9-aminoacridines) 4, geometrically designed so as to encompass a two base-pair DNA sequence (four base-pair "sandwich").8 Unlike conventional flexible tethers, our diphenylmethane tether was intended to minimize unfavorable entropic consequences and self-stacking interactions,9 and also to deter "creeping" along the DNA, which drastically lowers ligand residence lifetimes at any one site.10 DNA-ligand residence lifetimes are correlated with in vivo antitumor activity for many DNA intercalators.11 In our screening of bis-acridines 4 in vitro in the L-1210 murine leukemia cell line, we found growth inhibition of ID50 = 0.081–0.090 µM.8 Consistent with manual docking of these and other bis-acridines with CPK models of DNA is that 5–8 are less active in the L-1210 screen (0.27–0.60 µM), as is 9-aminoacridine (9). Only 4, with a single pivot atom and a para, para-linkage is able to achieve a bis-intercalative binding with the B form of DNA.
RESULTS AND DISCUSSION
In an attempt to improve both the antitumor activity and solubility of this novel class of bis-acridines, we now describe the synthesis, purification, and preliminary antitumor activity and DNA binding of the isomeric bis(aminocyclohexyl)methane acridines 14–16 (Scheme 1). We anticipated that the more basic 9-alkylaminoacridines12 would have improved water solubility over the less basic 9-arylaminoacridines, although we did not explicitly measure their water solubilities. The reaction of 9-chloroacridine (10) with the commercial mixture of 4,4'-dicyclohexyldiaminomethane isomers 11–13 (75% trans, trans; 5% cis, cis; and 20% cis, trans) gave the expected mixture of isomers 14–16. However, since these could not be readily separated by silica chromatography, we separated and purified the individual diamines 11–13.13,14 Thus, the commercial diamine mixture was distilled and fractionally crystallized to separate most of the dominant trans, trans 11, which was acetylated to form the bis-acetamide. The remaining mixture of mainly 12 and 13 was acetylated to give the corresponding bis-acetamides, which were separated by fractional crystallization. All three air-stable bis-acetamides were recrystallized at least four times (to constant melting point), hydrolyzed with concentrated hydrochloric acid, and finally purified by distillation to give separate diamines 11–13. These were distinguished by proton NMR according to the literature chemical shifts of the amino methine protons.14 The diamines were allowed to react with 9-chloroacridine (10) in warm phenol to afford the individual bis-acridines 14–16, in 70%, 28%, and 79% yields, respectively, which were characterized by elemental analyses and spectral data. Notably, each isomer could be distinguished by the chemical shift of the corresponding amino methine proton. Thus, trans, trans 14 shows a multiplet at 4.5 ppm (axial protons), cis, cis 15 at 4.75 ppm (equatorial protons), and cis, trans 16 shows multiplets at both positions. The clarity of this region in these NMR spectra confirms the purity of 14–16.
We were somewhat surprised to find that the in vitro antitumor activity of 14–16 against L-1210 murine leukemia is essentially identical (Table 1), and the weighted average (0.51 µM) is close to that determined for the mixture of 14–16 (0.60 µM). Also, the DNA melting temperature (∆Tm) data reveal that all three isomers are equipotent in their binding to DNA. The magnitude of the ∆Tm values suggests that the mode of binding is bis-intercalative. We were initially inclined to believe, from an examination of CPK space filling models of DNA and 14–16, that the cis, cis isomer 15 would be the most effective bis-intercalator, but it seems clear from the ∆Tm data that all three bis-acridines 14–16 are sufficiently flexible so as to bis-intercalate DNA, which can also adjust to the requirements of intercalation. The energy gained in DNA complexation must override the energy costs in forcing unfavorable boat and/or twist-boat cyclohexyl conformations for bis-intercalation to occur. 9-Aminoacridine (9) is included as a control compound. Analysis of the thermal denaturation studies in poly AT and poly GC DNA indicates that the bis-acridines have a greater impact on denaturation temperature, and potentially a larger binding constant, with the AT rich regions of genomic DNA. This impact is much greater for the purported bis-intercalators than for the mono-intercalating ethidium bromide as evidenced by the ratio of poly AT/poly GC ∆Tm values (average 3.34 ratio for the bis-acridines vs. 1.87 ratio for ethidium bromide). This result lends further support that two intercalation events occur in the interaction of our bis-acridines with duplex DNA.
In conclusion, we have described the first examples of non-aromatic, semi-rigid tethered bis-acridines that display antitumor activity in the in vitro L-1210 murine leukemic screen. Given the expected increased basicity of the acridine ring nitrogen, it should be possible to prepare water-soluble salts and alkylated derivatives of 14–16 for in vivo screening. Such salts should be significantly more soluble than the free-bases.
EXPERIMENTAL
Melting points were determined in open capillary tubes with a Büchi 510 melting point apparatus and are uncorrected. Nuclear magnetic resonance spectra were recorded on a Varian XL-300 multinuclear Fourier transform instrument and are reported in parts per million from tetramethylsilane on the δ scale. The internal standard was trifluoroacetic acid, unless otherwise noted, which shows a resonance at 11.50 δ. Data are reported as follows: chemical shift [multiplicity (s = singlet, m = multiplet), integration]. Mass spectra were recorded either using fast atom bombardment (FAB) provided by the NIH Regional Facility located at the Massachusetts Institute of Technology (Cambridge, MA), or electron impact (20 eV) courtesy of Dr. M. Cory at Burroughs-Wellcome. Samples on which exact masses were measured exhibited no significant peaks at m/e greater than that of the parent. Combustion analyses were performed by Atlantic Microlabs (Norcross, AL). Attempts to remove THF solvent from 14–16 were unsuccessful. UV-visible spectra were recorded on a Hewlett-Packard 8451A Diode-Array Spectrophotometer from 300 nm to 820 nm. Extinction coefficients (ε) were not determined. The λmax values reported indicate the largest absorption in the range studied. Absorptions between 300 nm and 190 nm are not reported. Flash chromatography was performed on silica gel (Kieselgel 60, 230–400 mesh ASTM) from EM Science. Analytical thin layer chromatography (TLC) was carried out on 200 µM Kieselgel 60 F254 coated plastic sheets (Merck). The spots were visualized, after use of a mobile phase (ethyl ether, unless otherwise noted), with 254 nm UV light or by spraying with concentrated H2SO4 and heating. All reactions were carried out under a nitrogen or argon atmosphere in oven-dried flasks unless otherwise stated. The calf thymus DNA and polynucleotides were obtained from commercial sources and quantified using spectroscopy. The highest grade commercial quality was used.
9-Chloroacridine (10) (method A): A magnetically stirred solution of N-phenylanthranilic acid (5.45 g, 26 mmol) in phosphorus oxychloride (100 mL, 1.07 mol) was treated with concentrated sulfuric acid (0.5 mL), then brought to reflux for 12 h. The solution was cooled to rt, added slowly to cracked ice (650 g), and made slightly alkaline with concentrated ammonium hydroxide. A light tan solid was collected by filtration, washed with water, and air dried for 24 h. Flash chromatography (silica gel/75% hexane, 20% THF, 5% triethylamine) afforded 4.67 g (84% yield) of 10 as fine yellow needles having one medium Rf spot by TLC; mp 117–119 °C (lit.15 mp 119–120 °C); 1H NMR (CDCl3): δ 8.50–8.10 (m, 4H), 7.95–7.50 (m, 4H); IR (THF): 3075, 1622, 1562, 1438, 1404, 1324, 1287, 1014, 831, 767, 647, 608 cm-1; Vis (CHCl3): λmax (ε) 360.5 nm (10,000); MS EI: m/e (relative intensity) 215 (33, M++2), 213 (100, M+), 178 (21), 177 (16). Anal. Calcd for C13H8ClN: C, 73.07; H, 3.77; N, 6.55; Cl, 16.59. Found: C, 72.99; H, 3.80; N, 6.55; Cl, 16.58.
9-Chloroacridine (10) (method B): A magnetically stirred solution of 9-acridanone (4.01 g, 21 mmol) in phosphorus oxychloride (105 mL, 1.13 mol) was brought to reflux for 12 h. The solution was cooled to rt, added slowly to cracked ice (650 g), and made slightly alkaline with concentrated ammonium hydroxide. A light tan solid was collected by filtration, washed with water, and air dried for 24 h. Flash chromatography (silica gel/75% hexane, 20% THF, 5% triethylamine) afforded 3.97 g (89% yield) of 10 as fine yellow needles which was identical to material prepared by method A as judged by TLC, mp, and spectral data.
N,N'-(Methylenedi-4,1-cyclohexanediyl)bis[9-acridinamine] (mix of 14–16): A magnetically stirred solution of 9-chloroacridine (10) (0.51 g, 2.39 mol) and 4,4'-diaminodicyclohexylmethane (0.13 g, 0.62 mmol) was warmed at 80 °C in phenol (20 g, 0.21 mol) for 4 h. After being cooled to rt, the solidified yellow-orange reaction mixture was dissolved in chloroform (100 mL) and extracted with 0.1 N NaOH (2 x 100 mL), water (3 x 100 mL), and brine (1 x 100 mL). The organic phase was separated, dried over anhydrous sodium sulfate, and then concentrated in vacuo to afford, after flash chromatography (silica gel/95% ethyl ether, 5% triethylamine), a bright yellow, hygroscopic solid. The solid was recrystallized from THF/hexane and dried in vacuo at 40 °C for 2 d to give 0.25 g (71%) of 14–16 as a yellow powder showing a single, very low Rf spot by TLC; 1H NMR (60 MHz, CF3COOD): δ 8.45–7.20 (m, 16H), 2.70–1.25 (m, 22H); IR (THF): 3578, 3508, 3352, 1631, 1565, 1526, 1428, 1356, 1077, 766 cm-1; Vis (CHCl3): λmax (ε) 398.5 nm (15,400); MS FAB: m/e 565 (MH+). Anal. Calcd for C39H40N4•1/2 H2O: C, 81.64; H, 7.20; N, 9.76. Found: C, 81.53; H, 7.39; N, 9.36. In vitro L1210: ID50 = 0.09 µM (Vermont).
Separation of trans, trans-(11), cis, cis-(12), and cis, trans-(13) isomers of 4,4'-diaminodicyclohexylmethane: The commercial waxy paste was vacuum distilled (170–172 °C, 2–3 Torr) to give approximately 45 g of a colorless oil. The oil was dissolved in an equal volume of petroleum ether and allowed to crystallize. In this manner, colorless rods of 11 (10.9 g) were obtained. They were recrystallized from hexane twice more to give 6.0 g of 11 as rods and needles: mp 59–62 °C (lit.12 mp 64–65.4 °C). The remaining petroleum ether contained the other two isomers as well as a small amount of 11. The solvent was removed in vacuo and the oil was acetylated: To a flask containing the liquid isomer (33.9 g) was slowly added, with stirring, water (40 mL) and glacial acetic acid (40 mL). The temperature was kept between 50–60 °C. Then after all of the isomer had dissolved, acetic anhydride (70 mL) was added and the solution stirred at 50–60 °C for 1 h. Water (80 mL) was added to the solution and after 15 min the resultant sandy ppt was filtered, washed with water, and labeled fraction A. The mother liquor was cooled to rt and after 2 h filtered, the sandy ppt washed with water, and labeled fraction B. The mother liquor was diluted with water (200 mL) and refrigerated overnight. The sandy crystals that formed were filtered, washed with water, and labeled fraction C. The mother liquor was further diluted to 1 L and after refrigeration overnight gave a small amount of sandy crystals, which were filtered, washed with water, and labeled fraction D. The mother liquor was concentrated to 100 mL on a steam bath, and the ppt that formed after cooling was filtered, washed with water, and labeled fraction E. Each fraction was recrystallized from ethanol-water four times (each time the mother liquor was concentrated and another fraction isolated and labeled), to give feathery white needles. The fractions (8.5 g) melting at 272–278 °C were determined to be, by comparison to the literature values, the trans, trans-diacetyl derivative. The fractions (13.0 g) melting at 225–228 °C were determined to be the cis, trans- derivative, and those (0.86 g) at 240–243 °C were determined to be the cis, cis derivative, by comparison to the literature13 melting points. The free diamines of the cis, trans and cis, cis derivatives were then prepared. The trans, trans derivative was not transformed to the free diamine because it had been previously isolated from the crude mixture by fractional crystallization.
4,4'-cis, trans-Diaminodicyclohexylmethane (13): To the cis, trans-diacetyl derivative (4.42 g, 15.4 mmol) was added concentrated hydrochloric acid (50 mL) and the solution was refluxed for 24 h with stirring. Then, more hydrochloric acid (25 mL) was added and the solution was refluxed for 9 h. This was repeated once more, and finally the solution was allowed to stand at rt for 2 d. Neutralization of the clear solution with 50% aqueous sodium hydroxide gave a fluffy hydrate which was extracted with n-butanol (5 x 50 mL). The n-butanol extract was evaporated to a small volume and then washed with water to remove the salts. The remaining n-butanol was removed by distillation in vacuo to give a brown oil (3.0 mL). The oil was purified by distillation in vacuo (200 °C, 4 Torr) to give a colorless oil that when refrigerated overnight crystallized to give 13 as a waxy white solid (2.52 g, 79%): mp 26–28 °C (lit.13 mp 35.7–36.9 °C). This was used without further purification.
cis, cis-4,4'-Diaminodicyclohexylmethane (12): The cis, cis-diamine was prepared exactly as described above, to give after distillation in vacuo (200 °C, 4 Torr) an oil that crystallized on standing to give 12 as a waxy white solid (0.21 g, 79%): mp 57–59 °C (lit.13 mp 60.5–61.9 °C). This was used without further purification.
N,N'-trans, trans-Methylenedi-4,1-cyclohexanediyl-bis(9-acridinamine) (14): To a mixture of warm (55 °C) phenol (10.5 g) and 9-chloroacridine (1.21 g, 5.66 mmol) was added trans, trans-4,4'-dicyclohexyldiaminomethane (11) (0.36 g, 1.74 mmol). The orange solution was stirred at 55–65 °C for 20 h. The reaction mixture was cooled to rt, taken up in CHCl3 (100 mL), washed with 0.1 M NaOH (5 x 200 mL), water (2 x 100 mL), and brine (100 mL), and then dried (MgSO4). The orange organic phase was then filtered of drying agent and adsorbed onto silica gel. Short path flash chromatography (silica gel/hexane, Et2O, triethylamine, 5:20:1) gave 14 as orange-yellow powder. Recrystallization followed by drying in vacuo gave 0.68 g (70%) of 14 as a bright yellow powder with a single very low Rf spot by TLC. Mp 240-242 °C; UV-vis (CHCl3) λ = 400 nm, 418 nm, 440 nm; 1H-NMR: (TFA-d1) δ 8.3–7.5 (M, 16H); 4.5 (S, 2H); 2.6–1.2 (M, 20H); Anal. Calcd for C39H40N4 + THF (C4H8O): C, 81.09%; H, 7.59%; N, 8.80%. Found: C, 80.57%; H, 7.62%; N, 8.75%; HRMS Calcd for (M++1) C39H41N4: 565.3331; found, 565.3340.
N,N'-cis, cis-Methylenedi-4,1-cyclohexanediyl-bis(9-acridinamine) (15): To a yellow solution of phenol (10 g) and 9-chloroacridine at 65 °C was added cis, cis-4,4'-dicyclohexyldiaminomethane (12) (0.15 g, 0.727 mmol). The mixture was stirred at 65–75 °C for 24 h. Then after cooling the orange-yellow mixture to rt, it was taken up in CHCl3 (100 mL). The orange solution was washed with 0.1 M NaOH (5 x 100 mL), water (2 x 100 mL), and brine (100 mL), and dried (MgSO4). After adsorption onto silica gel, the mixture was subjected to short path flash chromatography (silica gel/Et2O, triethylamine (5%)) which gave a greenish yellow powder. Recrystallization (THF, hexane) and drying in vacuo afforded 0.115 g (28%) 15 as a bright yellow powder with a single, very low Rf spot by TLC. Mp 232–234 °C; UV-vis (CHCl3): λ = 400, 418, 440 nm; 1H-NMR (TFA-d1): δ 8.3–7.4 (M, 16H); 4.75 (S, 2H); 2.3–1.3 (M, 20H); Anal. Calcd for C39H40N4 + 1 THF (C4H8O): C, 81.09%; H, 7.59%; N, 8.80%. Found: C, 80.75%; H, 7.65%; N, 8.63%; HRMS Calcd for (M++1) C39H41N4: 565.3331; found, 565.3354.
N,N'-cis, trans-Methylenedi-(4,1-cyclohexanediyl)-bis(9-acridin-amine) (16): To a warm (65 °C) solution of phenol (10 g) and 9-chloroacridine (1.08 g, 5.05 mmol) was added cis, trans-4,4'-dicyclohexyldiaminomethane (13) (0.26 g, 1.26 mmol). The orange-yellow solution was stirred at 65 °C for 20.5 h before cooling to rt. The solidified mixture was then taken up in CHCl3 (100 mL), washed with 0.1 M NaOH (5 x 100 mL), water (2 x 100 mL), and brine (100 mL), and then dried (MgSO4). After adsorption onto silica gel, the mixture was subjected to short path flash chromatography (silica gel/Et2O, triethylamine (5%)) which afforded a yellow powder. Recrystallization (THF/hexane) and drying in vacuo overnight gave 0.56 g (79%) of 16 as a bright yellow powder, showing a single very low Rf spot by TLC. Mp 197–198 °C; UV-vis (CHCl3): λ = 400, 418, 440 nm; 1H-NMR (TFA-d1): δ 8.4–7.4 (M, 16H); 4.8 (S, 1H); 4.45 (S, 1H); 2.4–1.2 (M, 20H); Anal. Calcd for C39H40N4 + ½ THF (C4H8O): C, 82.15%; H, 7.33%; N, 9.44%. Found: C, 81.89%; H, 7.30%; N, 9.77%; HRMS Calcd for C39H41N4 (M++1): 565.3331; found, 565.3342.
Procedure for determining L1210 activity: The individual compounds were tested by Professor Miles P. Hacker, Department of Pharmacology, University of Vermont. L1210 cells (105) maintained as suspension cultures in McCoy's 5A medium (containing 10% horse serum, glutamine, penicillin, and streptomycin) were exposed to known concentrations (0.01, 0.1, and 10 µg/mL in DMSO) of the compounds in an atmosphere of 10% carbon dioxide and 90% air at 37 °C. After 72 h of continuous exposure the population of each culture was determined by a Coulter Counter (Model ZBF, Hialeah, FL) and compared to the control. The ratio of exposed cell population to control cell population was used to calculate the percent cell growth relative to control.8 This was used to calculate the IC50 value (the drug concentration required to inhibit cell growth by 50% of control).
Procedure for determination of ∆Tm values: The thermal denaturation studies were done by the method of Cory16 on a Varian 2290 UV-visible spectrophotometer with a heating rate of 18 °C/h. The five cuvettes were on a 2 min cycle time with a 5 s dwell time. ∆Tm were calculated from the printout using the difference between the compound Tm and the DNA. The calf thymus DNA had a Tm of 56.8 °C.
ACKNOWLEDGEMENTS
This investigation was supported by the National Institutes of Health, Merck Sharp and Dohme Reserch Laboratories, and the Research Committee of Dartmouth College. We also thank Dr. Catherine E. Costello and Chenhui Zeng (Massachusetts Institute of Technology) for high-resolution and FAB mass spectra (NIH Resource Grant FR00317 from the Division of Research Facilities and Resources). We wish to acknowledge Professor Miles Hacker, University of Vermont, for the L-1210 data.
References
1. For reviews, see (a) L. P. G. Wakelin, Med. Res. Rev., 1986, 6, 275; CrossRef (b) W. D. Wilson and R. L. Jones, 'Intercalation in Biological Systems,' ed. by M. S. Wittingham and A. J. Jacobson, Intercalation Chemistry, Academic Press, New York, 1982, pp. 445-501; (c) J.-B. Le Pecq and B. P. Roques, 'DNA Binding and Biological Properties of Bis and Tris Interacting Molecules,' ed. by M. G. Simic, L. Grossman, and A. C. Upton, Mechanisms of DNA Damage and Repair, Plenum Press, New York, 1986, pp. 219-230; (d) S. Neidle and M. J. Waring, 'Molecular Aspects of Anti-Cancer Drug Action,' MacMillan Press, London, 1983; (e) P. L. Hamilton and D. P. Arya, Nat. Prod. Rep., 2012, 29, 134; CrossRef (f) B. C. Baguley, Horizons Can. Res., 2012, 48, 47.
2. For recent examples, see (a) Y. Chu, V. Lynch, and B. L. Iverson, Tetrahedron, 2006, 62, 5536; CrossRef (b) Y. Chu, S. Sorey, D. W. Hoffman, and B. L. Iverson, J. Am. Chem. Soc., 2007, 129, 1304; CrossRef (c) L. D. Van Vliet, T. Ellis, P. J. Foley, L. Liu, F. M. Pfeffer, R. A. Russell, R. N. Warrener, F. Hollfelder, and M. J. Waring, J. Med. Chem., 2007, 50, 2326; CrossRef (d) T. Gaslonde, S. Michel, M. Koch, B. Pfeiffer, S. Léonce, A. Pierré, and F. Tillequin, Chem. Pharm. Bull., 2007, 55, 734; CrossRef (e) B. B. Hasinoff, H. Liang, X. Wu, L. J. Guziec, F. S. Guziec, Jr., K. Marshall, and J. C. Yalowich, Bioorg. Med. Chem., 2008, 16, 3959; CrossRef (f) L. M. Wilhelmsson, N. Kingi, and J. Bergman, J. Med. Chem., 2008, 51, 7744; CrossRef (g) J. Galisteo, P. Navarro, L. Campayo, M. J. R. Yunta, F. Gómez-Contreras, J. A. Villa-Pulgarin, B. G. Sierra, F. Mollinedo, J. Gonzalez, and E. Garcia-España, Bioorg. Med. Chem., 2010, 18, 5301; CrossRef (h) R. Zhang, X. Wu, J. C. Yalowich, and B. B. Hasinoff, Bioorg. Med. Chem., 2011, 19, 7023; CrossRef (i) D. H. Paik and T. T. Perkins, Angew. Chem. Int. Ed., 2012, 51, 1811; CrossRef (j) K. Duskova, S. Sierra, M.-J. Fernández, L. Gude, and A. Lorente, Bioorg. Med. Chem., 2012, 20, 7112. CrossRef
3. (a) For a review of ditercalinium and other bis-pyridocarbazoles, see G. W. Gribble, 'The Alkaloids,' Vol. 39, ed. by A. Brossi, Academic Press, New York, 1990, pp. 239-352; (b) For a leading reference on ditercalinium and novel dimers in the 7H-pyrido[4,3-c]carbazole series, see C. Garbay-Jaureguiberry, M. C. Barsi, A. Jacquemin-Sablon, J. B. Le Pecq, and B. P. Roques, J. Med. Chem., 1992, 35, 72; CrossRef (c) For its mode of action, see M. Okamaoto, T. Ohsato, K. Nakada, K. Isobe, J. N. Spelbrink, J.-I. Hayashi, N. Hamasaki, and D. Kang, Curr. Genet., 2003, 43, 364. CrossRef
4. For an excellent review of these natural products, see S. Dawson, J. P. Malkinson, D. Paumier, and M. Searcey, Nat. Prod. Rep., 2007, 24, 109. CrossRef
5. For recent reviews, see (a) G. P. Moloney, D. P. Kelly, and P. Mack, Molecules, 2001, 6, 230; CrossRef (b) G. Gellerman, Org. Prep. Proc. Int., 2012, 44, 187; CrossRef (c) R. Kumar, M. Kaur, and M. Kumari, Acta Pol. Pharm. Drug Res., 2012, 69, 3; (d) B. C. Baguley, L. P. G. Wakelin, J. D. Jacintho, and P. Kovacic, Curr. Med. Chem., 2003, 10, 2643. CrossRef
6. For recent examples, see (a) Z. Yan, S. Sikri, D. L. Beveridge, and A. M. Baranger, J. Med. Chem., 2007, 50, 4096; CrossRef (b) S.-S. Wang, Y.-J. Lee, S.-C. Hsu, H.-O. Chang, W.-K. Yin, L.-S. Chang, and S.-Y. Chou, Bioorg. Med. Chem., 2007, 15, 735; CrossRef (c) C. Martins, M. Gunaratnam, J. Stuart, V. Makwana, O. Greciano, A. P. Reszka, L. R. Kelland, and S. Neidle, Bioorg. Med. Chem. Lett., 2007, 17, 2293; CrossRef (d) Z. Ma, J. R. Choudhury, M. W. Wright, C. S. Day, G. Saluta, G. L. Kucera, and U. Bierbach, J. Med. Chem., 2008, 51, 7574; CrossRef (e) H. T. N. Thi, C.-Y. Lee, K. Teruya, W.-Y. Ong, K. Doh-ura, and M.-L. Go, Bioorg. Med. Chem., 2008, 16, 6737; CrossRef (f) Z. He, X. Bu, A. Eleftheriou, M. Zihlif, Z. Qing, B. W. Stewart, and L. P. G. Wakelin, Bioorg. Med. Chem., 2008, 16, 4390; CrossRef (g) N. Desbois, M. Gardette, J. Papon, P. Labarre, A. Maisonial, P. Auzeloux, C. Lartigue, B. Bouchon, E. Debiton, Y. Blache, O. Chavignon, J.-C. Teulade, J. Maublant, J.-C. Madelmont, N. Moins, and J.-M. Chezal, Bioorg. Med. Chem., 2008, 16, 7671; CrossRef (h) A. Kumar, K. Srivastava, S. R. Kumar, S. K. Puri, and P. M. S. Chauhan, Bioorg. Med. Chem. Lett., 2009, 19, 6996; CrossRef (i) A. Chilin, G. Marzaro, C. Marzano, L. Dalla Via, M. G. Ferlin, G. Pastorini, and A. Guiotto, Bioorg. Med. Chem., 2009, 17, 523; CrossRef (j) Y.-T. Fu, B. R. Keppler, J. Soares, and M. B. Jarstfer, Bioorg. Med. Chem., 2009, 17, 2030; CrossRef (k) L. C. Eiter, N. W. Hall, C. S. Day, G. Saluta, G. L. Kucera, and U. Bierbach, J. Med. Chem., 2009, 52, 6519; CrossRef (l) X. Luan, C. Gao, Q. Sun, C. Tan, H. Liu, Y. Jin, and Y. Jiang, Chem. Lett., 2011, 40, 728. CrossRef
7. M. A. Horstmann, W. A. Hassenpflug, U. Zur Stadt, G. Escherich, G. Janka, and H. Kabisch, Haematologia, 2005, 90, 1701.
8. G. D. Jaycox, G. W. Gribble, and M. P. Hacker, J. Heterocycl. Chem., 1987, 24, 1405. CrossRef
9. (a) J. Barbet, B. P. Roques, S. Combrisson, and J.-B. Le Pecq, Biochemistry, 1976, 15, 2642; CrossRef (b) N. Capelle, J. Barbet, P. Dessen, S. Blanquet, B. P. Roques, and J.-B. Le Pecq, Biochemistry, 1979, 18, 3354. CrossRef
10. (a) W. A. Denny, G. J. Atwell, B. C. Baguley, and L. P. G. Wakelin, J. Med. Chem., 1985, 28, 1568; CrossRef (b) W. A. Denny, B. C. Baguley, B. F. Cain, and M. J. Waring, 'Molecular Aspects of Anti-Cancer Drug Action,' ed. by S. Neidle and M. J. Waring, MacMillan, New York, 1983, Chapter 1.
11. J. Feigon, W. A. Denny, W. Leupin, and D. R. Kearns, J. Med. Chem., 1984, 27, 450. CrossRef
12. A. Albert and R. Goldacre, J. Chem. Soc., 1946, 706. CrossRef
13. A. E. Barkdoll, H. W. Gray, and W. Kirk, Jr., J. Am. Chem. Soc., 1951, 73, 741. CrossRef
14. G. E. Hahn, P. Kusch, V. Rossbach, and H. Zahn, Makromol. Chem., 1985, 186, 297. CrossRef
15. A. Albert and B. Ritchie, Org. Synth., 1942, 22, 5.
16. (a) M. Cory, D. D. McKee, J. Kagan, D. W. Henry, and J. A. Miller, J. Am. Chem. Soc., 1985, 107, 2528; CrossRef (b) M. Cory, R. R. Tidwell, and T. A. Fairley, J. Med. Chem., 1992, 35, 431. CrossRef