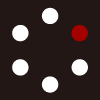
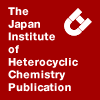
HETEROCYCLES
An International Journal for Reviews and Communications in Heterocyclic ChemistryWeb Edition ISSN: 1881-0942
Published online by The Japan Institute of Heterocyclic Chemistry
e-Journal
Full Text HTML
Received, 15th May, 2013, Accepted, 19th June, 2013, Published online, 27th June, 2013.
DOI: 10.3987/COM-13-12746
■ Synthesis, Characterization and Suppression of Impurities during Optimization of Dabigatran Etexilate
Yu Chen,* Jun Liang, Huansheng Chen, and Li Yuan
R&D Department, Shanghai AoBo Bio-pharmaceutical Technology Co., Ltd., Room 601, No. 1011, Halei Road, Zhangjiang High-Tech Park, Shanghai, 201203, China
Abstract
The synthetic methods for two impurities of dabigatran etexilate are firstly described, and both of two impurities are characterized by NMR and MS spectral data. The suppression of impurities as well as the optimization process of dabigatran etexilate is also disclosed.INTRODUCTION
Dabigatran etexilate (1, Figure 1) is the oral double pro-drug of dabigatran, developed by Boehringer Ingelheim with the brand name Pradaxa.1 This substance is a reversible thrombin inhibitor which is indicated to reduce the risk of stroke and systemic embolism in patients with non-valvular atrial fibrillation.2 Dabigatran etexilate (1) was first disclosed in WO 9837075,3 afterwards, although several improved processes for this drug have been developed,4 few concerned the impurities.5
During our exploration on process development of dabigatran etexilate (1), we found that according to the known processes, the purity of 1 could not reach the desired level formulated by ICH.6 Total impurities in about 1−1.5% content by HPLC analysis were observed even after the free base or salt such as MeSO3H salt of 1 was carefully recrystallized for three times.7 Three major impurities in the range of 0.1−0.9% each were separated and characterized as 2−4 (Figure 2). The first one, ethyl 2-[[4-[(n-hexyloxycarbonyl)aminoiminomethyl]phenylamino]methyl]-1-methyl-1H-benzimidazole-5-carboxylate (2), has a similar structure with dabigatran etexilate. This impurity was first reported in WO 2012152855.8 But according to that method, compound 2 was obtained as a minor byproduct and had to be separated from the reaction mixture by column chromatography in low content with the structure deduced only by HPLC. The second is ethyl 3-[[2-[(4-carbamimidoyl-phenylamino)-methyl]-1-methyl- 1H-benzimidazole-5-carbonyl]-pyridin-2-yl-amino]-propionate (3). It was presumptively from the hydrolysis of 1 in the reaction course or the purification operation at a higher temperature.9 In addition, we also found a new impurity, ethyl 3-[(2-methyl-1-methyl-1H-benzimidazol-5-carbonyl)-pyridin-2-yl- amino]-propionate (4). We know that the discovery and control of the impurities plays an important role in ensuring the quality and safety of the drug products. Herein, we report the convenient synthesis and characterization for impurities 2 and 4, which would provide sufficient quantities to satisfy the analytical need under regulatory requirements. Moreover, this would also help in the mechanistic aspect of the formation of these impurities to realize the optimization of dabigatran etexilate (1) with high purity as well as the control of all the impurities no more than 0.05% level.
RESULTS AND DISCUSSION
We began with the synthesis of ethyl 3-[[2-[(4-cyanophenylamino)-methyl]-1-methyl-1H- benzimidazole-5-carbonyl]-pyridin-2-yl-amino]-propionate (7), which was obtained from the reaction of ethyl 3-[[3-amino-4-(methylamino)benzoyl](pyridin-2-yl)amino]-propionate (5) with [(4-cyanophenyl)- amino]acetic acid (6) according to the known method (Scheme 1).3,4a-b
During the development, we found that alcoholysis impurity 9 was easily produced through the Pinner reaction10-amination of 7 according to the published method for 1. Specially, the higher temperature in Pinner reaction was used, the more alcoholysis impurity was produced. In the end, the acylated derivative 2 would be brought to dabigatran etexilate (1) which led to a hard purification because of the structure-similarity. Therefore, in order to synthesize 2 (Scheme 2), we carried out Pinner reaction at a higher temperature (35 °C) for 2 days on purpose to make 7 be transformed to 8-HCl completely. The obtained oil compound 8-HCl which was observed to be a little unstable, underwent amination quickly to give another oil crude ethyl 2-[[4-(aminoiminomethyl)phenylamino]methyl]-1-methyl-1H- benzimidazole-5-carboxylate (9). However, oil crude 9 must be purified by column chromatography before acylation, assuring to obtain high-qualified 2. Unfortunately, the high polarity of 9 led to a very hard separation. Attempt to transform this oil 9 to its HCl salt also gave an oil.
We found the key point laid in the low purity of 7 prepared from the present method. In terms of the present method,3,4a-b 7 was formed in excess of HOAc as solvent at a high temperature. After the reaction, several impurities were formed, one of which, was the main impurity identified as 4 by NMR and MS. Notably, 7 was obtained as a semisolid which was found not to be easy for purification by recrystallization. Consequently, low purity of 7 caused more impurities in the following reactions. Because impurity 4 was brought to the final molecule, either the impurity 2 or dabigatran etexilate (1), we had to make sure that compound 7 had enough purity first.
To overcome the problem, we employed ethyl acetate as solvent with 1 equiv of HOAc after the condensation of 5 with 6. The reaction proceeded very well with the greatly reduced amount of impurity 4.11 As soon as the ring-closing reaction finished, succinic acid was added directly into the mixture, and its salt (10) precipitated easily in 82% yield with 99.7% purity (impurity 4 was not detected12). It meant that 10 had enough purity not to be recrystallized further. The succinic acid form of 10 was superior to its oxalic acid one reported in WO 2009111997,13 which needed further recrystallization to acquire higher purity (97.8%) at the cost of yield (57.2%). Moreover, the great reduction of HOAc helps reducing acidic corrosion as well as strengthening environmental protection. Subsequently, 10 was dissolved in 7 mol/L (7N) HCl-EtOH solution at 35 oC for 2 days. Due to the enough purity of 10, solid precipitated from the mixture. This solid was treated with 4.5 mol/L (4.5N) NH3-EtOH solution immediately after filtration. After further treatment with HCl-EtOH solution, compound 9 was obtained in the form of salt to precipitate as a white solid in 64% yield from 10. Finally, 2 was synthesized by the acylation of 9-HCl with n-hexyl chloroformate. The whole route for 2 started from 5 or 6 in 31% overall yield without separation by chromatography column for every step (Scheme 3).
Evidently, the formation of 4 was from the ring-closing of residual compound 5 with HOAc. Therefore, after refluxed reaction of 5 in HOAc as solvent, 4 could be obtained in good yield (Scheme 4). Of course, using our improved method, impurity 4 could be completely eliminated in 10.
Next, we turned our attention to suppress impurity 2. According to the known method,8 impurity 2 existed in the final product (1) in about 10% content, which led to very careful purification to get a high quality product. In order to decrease the content of impurity 2 as much as possible in the crude final product, we explored the Pinner reaction which was responsible for the formation of this impurity (Scheme 5).
First we examined the effect of different acidic level with 7N HCl-EtOH solution on the Pinner reaction at room temperature (20−25 °C). The results were showed in Table 1. When 20 equiv of HCl was used, the reaction proceeded a little slowly with more 10 left (Table 1, entry 1). The reaction was not improved when the reaction time was extended but alcoholysis impurity 8 was slightly increased (Table 1, entry 2). 40 equiv of HCl gave a better result, more 10 was converted with moderate amount of 8 produced in 12 h (Table 1, entry 4). Shortening the time led to less 8, but, the transformation of 10 was also suppressed (Table 1, entry 3). The reaction was accelerated when more equiv of HCl was used, but more 8 was quickly formed even in a shorter time (Table 1, entries 5−8).
Then we compared different reaction time and temperature with 40 equiv of HCl to obtain a best condition. From Table 2, we could see that lowing temperature reduced 8 with moderate yield of 11, although more 10 left (Table 2, entries 1−2). Pleasingly, the reaction took place a little slowly but very well at 5−10 °C. The desired product 11 was produced in good yield with trace of 8 in 30 h (Table 2, entries 3−5). Further extension of time did not promote the reaction (Table 2, entry 6). The lower temperature resulted in the slower reaction. For example, it took 48 h to acquire a good result when the reaction was carried out at 0−5 oC (Table 2, entries 7−8).
The synthesis and purification of dabigatran etexilate (1) turned out to be much easier after the optimization of Pinner reaction was finished. The transformation of 10 to 3 could be realized in one pot. Compound 3 finally reacted with n-hexyl chloroformate in Et3N-DCM system instead of water-containing system widely used by published processes to produce dabigatran etexilate (1). Because of good suppression of alcoholysis impurity in Pinner reaction, its derivative 2 was detected in only 0.27% by HPLC in the reaction mixture. After simple purification, dabigatran etexilate (1) was obtained in 77% yield with 99.9% purity, and each impurity was detected no more than 0.05% by HPLC (Scheme 6).
In the final step, the reagents and solvents using either in the reaction or the purification course have to be dried in advance. Consequently, the hydrolysis of 1 could be avoided (Scheme 7).
EXPERIMENTAL
General. Melting points were recorded on an RY-1 melting point apparatus and are uncorrected. 1H (400 MHz) and 13C (100 MHz) NMR spectra were recorded on a Bruker Avance 400 spectrometer in CDCl3, DMSO-d6 or CD3CO2D using tetramethylsilane (TMS) as internal standards. J values are given in hertz. Mass spectra were recorded on a Finnigan MAT-95/711 spectrometer. Elemental analysis was performed on an MOD-1106 instrument. HPLC analysis was performed by a standard method on a Agilent ZORBAX SB-Phenyl, 250 mm × 4.6 mm (5 µm), λ = 220 nm, with acetonitrile buffer (2.04 g of KH2PO4 in 1.0 L of water and pH 3.0 with ortho phosphoric acid. The HPLC analysis data are reported in area % and are not adjusted to weight %.
Ethyl 3-[[2-[(4-cyanophenylamino)-methyl]-1-methyl-1H-benzimidazole-5-carbonyl]-pyridin-2-yl-amino]-propionate succinic acid salt (10).
To a stirred solution of 6 (51.6 g, 0.29 mol) and CDI (47.5 g, 0.29 mol) in THF (1.2 L) was added 5 (100.0 g, 0.29 mol) at 25 °C. The mixture was stirred for 24 h at 25 °C. EtOAc (1.2 L) was added after THF was evaporated. Then the organic solution was washed successively with saturated aq sodium hydrogen carbonate (1 L) and brine (1 L), dried with anhydrous Na2SO4. HOAc (17.4 g, 0.29 mol) was added to above solution after filtration, and the mixture was heated at 70 °C for 12 h. The solution was washed successively with saturated aq sodium hydrogen carbonate (500 mL) and brine (500 mL), dried with anhydrous Na2SO4. Succinic acid (34.2 g, 0.29 mol) was added after filtration. The solid was collected after precipitation completely, washed with EtOAc (100 mL) and dried at 50 °C under vacuum to give 10 as a pale-green solid (142.9 g, yield: 82%, purity by HPLC 99.7%, retention time: 18.1 min). Mp 136−138 °C. 1H NMR (DMSO-d6, 400 MHz): δ = 1.10 (t, J = 7.2 Hz, 3H), 2.39 (s, 4H), 2.65 (t, J = 7.2 Hz, 2H), 3.73 (s, 3H), 3.95 (q, J = 7.2 Hz, 2H), 4.20 (t, J = 7.2 Hz, 2H), 4.57 (d, J = 5.6 Hz, 2H), 6.79 (d, J = 8.4 Hz, 2H), 6.87 (d, J = 8.0 Hz, 1H), 7.10 (t, J = 7.2 Hz, 1H), 7.14 (d, J = 8.4 Hz, 1H), 7.23 (t, J = 8.4 Hz, 1H), 7.38 (d, J = 8.4 Hz, 1H), 7.42−7.47 (m, 3H), 7.52 (dt, J = 7.6 Hz, 1.6 Hz, 1H), 8.36 (d, J = 2.8 Hz, 1H), 12.12 (br s, 2H); 13C NMR (DMSO-d6, 100 MHz): δ = 14.3, 29.3, 30.3, 33.5, 44.9, 60.5, 97.3, 109.9, 112.8, 120.0, 120.9, 121.7, 122.6, 123.4, 129.9, 133.8, 137.7, 138.3, 141.2, 149.1, 152.2, 153.7, 156.5, 170.8, 171.5, 174.2 (one carbon-peak at high field was hidden in the peaks of DMSO)15; ESI-MS (m/z) 483 [M + H]+.
Ethyl 2-[[4-(aminoiminomethyl)phenylamino]methyl]-1-methyl-1H-benzimidazole-5-carboxylate hydrochloride (9-HCl).
10 (140.0 g, 0.23 mol) was dissolved in 7 mol/L HCl-EtOH solution (2.5 L) in a three-neck flask equipped with a drying tube. The mixture was stirred at 35 °C for 2 days. The precipitated solid was suspended in EtOH (1 L) immediately after filtration, and saturated NH3-EtOH solution (1 L) was then added at 0 °C. The solvent was removed, after the mixture was stirred at 0 °C for 24 h. EtOH (500 mL) was added to the residue then was evaporated to eliminate NH3 completely. The residue was slurried in water (100 mL) to form a crude solid. After dried, this soild was treated with saturated HCl-EtOH solution (1 L), and the mixture was stirred for 12 h. White solid was collected after filtration, washed with EtOH (100 mL) and dried at 60 °C under vacuum to give 9-HCl as an off-white solid (58.2 g, yield: 64%, purity by HPLC 99.2%, retention time: 5.0 min). Mp 172−174 °C. 1H NMR (DMSO-d6, 400 MHz): δ = 1.34 (t, J = 7.2 Hz, 3H), 3.88 (s, 3H), 4.32 (q, J = 7.2 Hz, 2H), 4.74 (d, J = 5.2 Hz, 2H), 6.90 (d, J = 9.2 Hz, 2H), 7.57 (t, J = 5.2 Hz, 1H), 7.66−7.69 (m, 3H), 7.89 (dd, J = 8.8 Hz, 1.6 Hz, 1H), 8.20 (d, J = 1.6 Hz, 1H), 8.70 (s, 2H), 8.91 (s, 2H); 13C NMR (DMSO-d6, 100 MHz): δ = 14.7, 30.7, 60.9, 110.5, 112.2, 113.6, 120.8, 123.7, 123.9, 130.2, 139.8, 141.6, 153.6, 154.5, 164.9, 166.7 (one carbon-peak at high field was hidden in the peaks of DMSO)15; ESI-MS (m/z) 352 [M + H]+.
Ethyl 2-[[4-[(n-hexyloxycarbonyl)aminoiminomethyl]phenylamino]methyl]-1-methyl-1H-benzimidazole-5-carboxylate (2).
To the mixture of 9-HCl (58.2 g, 0.15 mol) and Et3N (151.6 g, 1.5 mol) in DCM (1.5 L) was added n-hexyl chloroformate (123.5 g, 0.75 mol). The insoluble solid was removed by filtration after the mixture was refluxed for 2 h. The filtrate was washed with water (1 L), dried with anhydrous Na2SO4, filtered and concentrated to afford oil. The oil was distilled under vacuum at 1 mmHg to remove the excessive n-hexyl chloroformate. The residue was finally crystallized in EtOAc (400 mL) to give 2 as a white solid after dried at 40 °C under vacuum (42.4 g, yield: 59%, purity by HPLC 99.3%, retention time: 20.2 min). Mp 107−109 °C. 1H NMR (DMSO-d6, 400 MHz): δ = 0.86 (t, J = 7.2 Hz, 3H), 1.28−1.36 (m, 9H), 1.58 (t, J = 7.2 Hz, 2H), 3.87 (s, 3H), 3.98 (t, J = 6.8 Hz, 2H), 4.32 (q, J = 7.2 Hz, 2H), 4.68 (d, J = 5.6 Hz, 2H), 6.81 (d, J = 9.2 Hz, 2H), 7.05 (t, J = 5.2 Hz, 1H), 7.64 (d, J = 8.8 Hz, 1H), 7.83 (d, J = 8.4 Hz, 2H), 7.89 (d, J = 8.4 Hz, 1H), 8.23 (s, 1H), 8.66 (br s, 1H), 9.18 (br s, 1H); 13C NMR (DMSO-d6, 100 MHz): δ = 14.3, 14.6, 22.5, 25.7, 29.0, 30.4, 31.5, 60.9, 64.6, 110.3, 111.9, 120.9, 121.7, 123.7, 123.9, 129.7, 139.9, 141.9, 152.0, 154.7, 164.8, 166.7, 167.0 (one carbon-peak at high field was hidden in the peaks of DMSO)15; ESI-MS (m/z) 480 [M + H]+. Anal. Calcd for C26H33N5O4: C, 65.12; H, 6.94; N, 14.60. Found: C, 65.23; H, 6.89; N, 14.51.
Ethyl 3-[(2-methyl-1-methyl-1H-benzimidazole-5-carbonyl)-pyridin-2-yl-amino]-propionate (4).
A solution of 5 (102.7 g, 0.3 mol) in HOAc (1 L) was refluxed for 12 h. Saturated aq sodium hydrogen carbonate (500 mL), EtOH (2 L) were successively added after HOAc was evaporated, when stirring to produce a gray solid. This gray solid was collected after filtration, washed with water (500 mL) and EtOH (500 mL) and dried at 60 °C under vacuum to give 4 (82.5 g, yield: 75%, purity by HPLC 99.6%, retention time: 4.5 min). Mp 169−171 °C. 1H NMR (DMSO-d6, 400 MHz): δ = 1.12 (t, J = 7.2 Hz, 3H), 2.47 (s, 3H), 2.68 (t, J = 7.2 Hz, 2H), 3.67 (s, 3H), 3.97 (q, J = 7.2 Hz, 2H), 4.23 (t, J = 7.2 Hz, 2H), 6.85 (d, J = 8.0 Hz, 1H), 7.10−7.14 (m, 2H), 7.34 (d, J = 8.0 Hz, 2H), 7.53 (dt, J = 8.0, 1.6 Hz, 1H), 8.40 (dd, J = 4.8, 1.2 Hz, 1H); 13C NMR (CD3CO2D,16 100 MHz): δ = 11.3, 13.5, 30.7, 32.7, 45.4, 60.9, 111.5, 115.8, 123.0, 123.5, 125.4, 131.4, 132.7, 134.1, 139.7, 148.6, 153.9, 154.8, 170.5, 172.2; ESI-MS (m/z) 367 [M + H]+. Anal. Calcd for C20H22N4O3: C, 65.56; H, 6.05; N, 15.29. Found: C, 65.69; H, 5.99; N, 15.10.
Ethyl 3-[[2-[(4-carbamimidoyl-phenylamino)-methyl]-1-methyl-1H-benzimidazole-5-carbonyl]pyridin-2-yl-amino]-propionate (3).
10 (2.40 Kg, 4 mol, synthesized from 5 mol of 6 with the same method mentioned above) was dissolved in 7 mol/L HCl-EtOH solution (22.8 L) at 5−10 °C, and the mixture was stirred at 5−10 °C for 30 h. Subsequently, 4.5 mol/L NH3-EtOH solution (44 L) was added slowly at 0 °C, and the mixture was stirred for 24 h at 0 °C. After the solid was removed by filtration, the filtrate was concentrated to afford oil, which was then dissolved in a mixture of EtOH (10 L) and the solution of K2CO3 (828 g, 6 mol) in H2O (2 L). The mixture was stirred at 25 °C for 24 h, then concentrated to 20% of the total volume. Solid was precipitated when H2O (5 L) was added. This white solid was collected and dried at 70 °C under vacuum to give 3 (1.70 Kg, yield: 85%, purity by HPLC 98.7%, retention time: 5.5 min). Mp 268−270 °C. 1H NMR (DMSO-d6, 400 MHz): δ = 1.12 (t, J = 7.2 Hz, 3H), 2.68 (t, J = 7.2 Hz, 2H), 3.78 (s, 3H), 3.97 (q, J = 7.2 Hz, 2H), 4.23 (t, J = 7.2 Hz, 2H), 4.63 (s, 2H), 6.84 (d, J = 8.8 Hz, 2H), 6.90 (d, J = 8.0 Hz, 1H), 7.11−7.16 (m, 2H), 7.34 (br, s, 1H), 7.40 (d, J = 8.4 Hz, 1H), 7.48 (d, J = 0.8 Hz, 1H), 7.55 (dt, J = 8.0 Hz, 2.0 Hz, 1H), 7.65 (d, J = 8.8 Hz, 2H), 8.39 (dd, J = 8.8 Hz, 1.6 Hz, 1H), 8.72 (br s, 2H); 13C NMR (DMSO-d6, 100 MHz): δ = 14.4, 30.6, 33.5, 44.8, 60.5, 110.0, 112.1, 113.5, 120.0, 121.8, 122.5, 123.3, 129.7, 130.2, 137.7, 138.4, 141.2, 149.1, 153.5, 153.9, 156.4, 164.9, 170.8, 171.5 (one carbon-peak at high field was hidden in the peaks of DMSO)15; ESI-MS (m/z) 500 [M + H]+.
Dabigatran etexilate (1).
All the solvents as well as regents were dried in advance. To the mixture of 3 (1.70 Kg, 3.4 mol) and Et3N (687.0 g, 6.8 mol) in DCM (16 L) was added n-hexyl chloroformate (625.0 g, 3.8 mol). The mixture was refluxed for 3 h, then cooled to 10 °C and washed with water (10 L) and brine (10 L) successively at that temperature. The separated organic layer was dried with anhydrous Na2SO4, filtered and concentrated to afford oil. The oil was dissolved in a refluxed EtOAc (10 L) to give a solid when cooled down. This solid was dried at 50 °C under vacuum to give 1 as a white solid (1.64 Kg, yield: 77%, purity by HPLC 99.9%, retention time: 19.1 min). Mp 127−128 °C (lit. mp 128−129 °C).4b 1H NMR (DMSO-d6, 400 MHz): δ = 0.87 (t, J = 6.4 Hz, 3H), 1.12 (t, J = 7.2 Hz, 3H), 1.25−1.35 (m, 6H), 1.54−1.61 (m, 2H), 2.68 (t, J = 6.8 Hz, 2H), 3.77 (s, 3H), 3.90−4.00 (m, 4H), 4.22 (t, J = 6.8 Hz, 2H), 4.60 (d, J = 4.8 Hz, 2H), 6.76 (d, J = 8.4 Hz, 2H), 6.89 (d, J = 8.0 Hz, 1H), 6.96 (t, J = 4.4 Hz, 1H), 7.10−7.18 (m, 2H), 7.40 (d, J = 8.4 Hz, 1H), 7.47 (s, 1H), 7.54 (t, J = 8.0 Hz, 1H), 7.79 (d, J = 8.4 Hz, 2H), 8.37−8.40 (m, 1H), 8.65 (br s, 1H) , 9.14 (br s, 1H); 13C NMR (CDCl3, 100 MHz): δ = 14.0, 14.1, 22.5, 25.6, 28.9, 29.7, 31.5, 33.3, 40.5, 44.7, 60.5, 65.3, 108.8, 111.9, 120.1, 121.1, 122.4, 122.8, 123.5, 129.1, 129.7, 137.2, 137.4, 141.0, 148.9, 150.5, 152.5, 156.0, 165.0, 167.7, 171.0, 171.6; ESI-MS (m/z) 628 [M + H]+. Anal. Calcd for C34H41N7O5: C, 65.05; H, 6.58; N, 15.62. Found: C, 65.13; H, 6.65; N, 15.50.
Ethyl 3-[[2-[[ethoxy(imino)methyl-phenylamino]-methyl]-1-methyl-1H-benzimidazole-5-carbonyl]pyridin-2-yl-amino]-propionate (11).
10 (6.0 g, 0.01 mol) was dissolved in 7 mol/L HCl-EtOH solution (57 mL) at 5−10 °C, and the mixture was stirred at 5−10 °C for 30 h. Saturated aq sodium hydrogen carbonate (300 mL) was slowly added at 0 °C, then EtOAc (300 mL) was added, and the separated water layer was extracted with EtOAc (150 mL) again. The combined organic layer was washed with brine (150 mL), dried with anhydrous Na2SO4, filtered and concentrated to afford a crude sample. This crude sample was purified via silica gel column chromatography using DCM and MeOH (from 1:10 to 1:1) as eluent to give recovered free base of 10 first (0.29 g, yield: 6%) and then 11 (4.7 g, yield: 89%) as a yellow foam-solid. Mp 105−108 °C. 1H NMR (DMSO-d6, 400 MHz): δ = 1.12 (t, J = 7.2 Hz, 3H), 1.34 (t, J = 6.8 Hz, 3H), 2.68 (t, J = 7.2 Hz, 2H), 3.77 (s, 3H), 3.97 (q, J = 7.2 Hz, 2H), 4.23 (t, J = 7.2 Hz, 2H), 4.29 (q, J = 7.2 Hz, 2H), 4.60 (d, J = 5.6 Hz, 2H), 6.79 (d, J = 8.8 Hz, 2H), 6.89 (d, J = 8.4 Hz, 1H), 7.10−7.18 (m, 3H), 7.40 (d, J = 8.8 Hz, 1H), 7.47 (d, J = 1.2 Hz, 1H), 7.54 (dt, J = 8.0 Hz, 1.6 Hz, 1H), 7.69 (d, J = 8.8 Hz, 2H), 8.40 (dd, J = 8.8 Hz, 1.2 Hz, 1H), 9.59 (br s, 1H); 13C NMR (DMSO-d6, 100 MHz): δ = 14.4, 14.4, 30.4, 33.5, 44.8, 60.5, 63.8, 109.9, 112.2, 116.9, 120.0, 121.7, 122.6, 123.3, 129.6, 129.8, 137.7, 138.3, 141.3, 149.2, 152.3, 154.1, 156.5, 167.4, 170.8, 171.5 (one carbon-peak at high field was hidden in the peaks of DMSO)15; ESI-MS (m/z) 529 [M + H]+. Anal. Calcd for C29H32N6O4: C, 65.89; H, 6.10; N, 15.90. Found: C, 65.99; H, 6.21; N, 15.83.
ACKNOWLEDGEMENTS
We thank Prof. Dr. Dawei Ma for his generous support and constant encouragement. We are also grateful for the cooperation from the colleagues of analytic department.
References
1. European Medicines Agency. CHMP assessment reports for pradaxa [2011-08-23]. http://www.emea.europa.eu/docs/en_GB/document_library/EPAR_-_Summary_for_the_public/human/000829/WC500041060.pdf.
2. M. Sanford and G. L. Plosker, Drugs, 2008, 68, 1699. CrossRef
3. N. Hauel, U. Ries, H. Priepke, W. Wienen, and J. M. Stassen, W. O. patent 9837075, 1998; CAN 129:216617.
4. (a) N. Hauel, H. Priepke, U. Ries, J. M. Stassen, and W. Wienen, U.S. patent 6,087,380, 2000; CAN 129:189325; (b) N. H. Hauel, H. Nar, H. Priepke, U. Ries, and J. M. Stassen, J. Med. Chem., 2002, 45, 1757; CrossRef (c) P. Lustig and J. Jirman, W. O. patent 2010045900, 2010; CAN 152:501376; (d) G. Zerban, A. Hausherr, R. Hamm, G. Koch, K. Schlarb, H. P. Schmitt, and B. Weyell, W. O. patent 2007071743, 2007; CAN 147:118241.
5. R. M. Satyanarayana, C. Nagaraju, R. S. Thirumalai, S. Eswaraiah, R. A. Kodanda, and S. Raghuram, W. O. patent 2012077136, 2012; CAN 157:104681.
6. ICH Q3A Impurities in New Drug Substances, R2; International Conference on Harmonisation of Technical Requirements for Registration of Pharmaceuticals for Human Use (ICH): Geneva, Switzerland, October 2006; http://www.ich.org/fileadmin/Public_Web_Site/ICH_Products/Guidelines/Quality/Q3A_R2/Step4/Q3A_R2__Guideline.pdf.
7. The crude compound 1 synthesized according to the method listed in references 3 and 4 was tried for purification. After screening of the solvent, EtOAc was proved to be best. HPLC showed that the three main impurities: impurity 4 (0.21%), impurity 3 (0.13%) and impurity 2 (0.82%) still existed after three recrystallization of crude 1 in EtOAc. On the contrary, our process for 1 was shown to be well impurities-controlled even just detected for the reaction mixture before purification. Finally, high purity was obtained through only one recrystallization in EtOAc. For detailed HPLC spectrum, Please see supporting information.
8. M. J. Serra, L. E. Duran, and L. Rao, W. O. patent 2012152855, 2012; CAN 157:708589.
9. For example, when compound 1 with 0.05% of impurity 3 was dissolved in dried DCM, the content of 3 kept unchanged after 24 h, so did 3 in DCM which was mixed with 50% (v/v) H2O below 10 °C after 24 h. However, 3 was increased to about 0.5% at 40 °C after 24 h in above-mentioned mixed solvent. It showed that it was necessary for drying the solvents and reagents in advance to avoid the hydrolysis of 1 in the reaction or purification course because of heating.
10. R. Goger and D. G. Neilson, Chem. Rev., 1961, 61, 179. CrossRef
11. Impurity 4 was decreased from 7.83% to 0.71% by HPLC in the reaction mixture after the ring-closing was finished, when 1 equiv of HOAc was used at 70 °C instead of excessive HOAc as the solvent at refluxed temperature.
12. If the ring-closing took place in HOAc as solvent according to the known process, at least three times recrystallization was necessary for 10 to eliminate impurity 4 completely, even if in the form of succinic acid.
13. J. Jirman, J. Richter, and P. Lustig, W. O. patent 2009111997, 2009; CAN 151:358757.
14. The detailed operation for the represented example was shown in supporting information.
15. Our compounds had little solubility in most solvents but DMSO, so DMSO-d6 was used as deuterated solvent. However, the “lack” of one carbon-peak at high field was observed for most compounds. The peak of NHC was supposed to be hidden in the peaks of DMSO. Luckily, dabigatran etexilate (1) was easily dissolved in CDCl3. Instead of 13CNMR in DMSO-d6, the “missing” carbon appeared in CDCl3. Please see Supporting Information.
16. Compound 4 was little soluble in almost all the solvents except HOAc, so 13C NMR had to be run in CD3CO2D to get a good spectra. Compound 4 might exits in the form of salt in CD3CO2D.