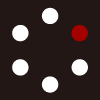
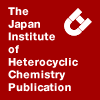
HETEROCYCLES
An International Journal for Reviews and Communications in Heterocyclic ChemistryWeb Edition ISSN: 1881-0942
Published online by The Japan Institute of Heterocyclic Chemistry
e-Journal
Full Text HTML
Received, 30th June, 2013, Accepted, 15th August, 2013, Published online, 21st August, 2013.
DOI: 10.3987/COM-13-S(S)71
■ Synthetic Studies on Furanosteroids. Functionalization of Ring A of the Viridin Core Skeleton
Kristen C. Mascall and Peter A. Jacobi*
6128 Burke Laboratory, Department of Chemistry, Dartmouth College, Hanover, New Hampshire 03755, U.S.A.
Abstract
The late-stage installation of an important carbonyl group on ring A of the viridin core skeleton is reported, via a two-step process consisting of benzylic bromination and Kornblum oxidation. The synthetic route described also provides access to several other interesting furanosteroid congeners.The furanosteroids represent a small family of natural products readily recognized by their novel pentacyclic skeleton.1 Compounds in this class bear structural resemblance to the larger family of steroids, with one unique modification – a furan ring fused at the C4 and C6 positions of the steroidal skeleton (Figure 1). The presence of the furan ring introduces significant strain in the scaffold, and is the site of important biological activity observed in these compounds.
Since their isolation, the furanosteroids have attracted attention because of their potent antifungal, anti-inflammatory and antibiotic properties.2 More recently, members of this class were shown to be potent inhibitors of phosphatidylinositol 3-kinases (PI-3K),3 an important family of enzymes involved in the intracellular signaling pathway essential for the growth and development of cells.4 It is believed that identification of selective PI-3K inhibitors can lead to the development of remedial agents for the treatment of patients with autoimmune disorders, as well as diseases characterized by rapid cell proliferation, such as cancer. Structure-activity relationship studies5 and site-directed mutagenesis6 revealed that wortmannin (2) irreversibly inhibits the PI-3K enzyme through a covalent bond formed by nucleophilic attack of a lysine residue on C21 of the furan ring, a position rendered highly electrophilic by the flanking carbonyl groups at C3 and C7 (Figure 2). Demethoxyviridin (3) was also found to inhibit mammalian PI-3K at very low concentrations (IC50 = 3.4 nM), comparable to wortmannin (IC50 = 7.1 nM).7 However, 2 and 3 also bind to many other targets in vivo,8 and the electrophilic furan ring is susceptible to facile hydrolysis. Therefore, there is a need for the development of furanosteroid analogs that can serve as more stable and selective PI-3K inhibitors.
For several years we have been developing new methodology for the synthesis of furanosteroids and related materials. In one approach, we reported the preparation of the truncated viridin core skeleton syn-4 (Figure 3c), utilizing an intramolecular Diels–Alder/retro Diels–Alder reaction of an alkyne oxazole as the key step to generate the benzofuran ring moiety (Figure 3a).9 This bis-heteroannulation strategy was also employed in the construction of viridin analog syn-510 and the wortmannin analog 611 (Figure 3c). Subsequently, we developed an improved synthesis of the viridin core skeleton, which featured the acid-promoted cyclodehydration of an aryloxyketone to prepare the benzofuran ring system (Figure 3b).12 This new route proved to be very efficient in providing syn-4 in nine steps from commercially available starting materials. With ready access to syn-4, we sought to explore the functionalization of ring A, in particular benzylic oxidation at C3 which would provide a model compound closely resembling demethoxyviridin (3). We believed that it was advantageous to install this key functional group at this late stage because it was shown that the presence of the C3 carbonyl group complicated earlier steps in the synthesis.
Our initial studies began with the unnatural isomer anti-4, and we attempted to achieve benzylic oxidation in a single step using common reagents such as SeO2 and DDQ. However, no reaction was observed under these conditions. Benzylic oxidation with IBX13 and KMnO4/MnO214 were also unsuccessful, and we postulated that the C3 position was not sufficiently activated to undergo a formal four-electron oxidation in one step. In contrast, it was found that anti-4 reacted readily with NBS under Wohl−Ziegler conditions15 to give the corresponding brominated derivative anti-7 as an inconsequential mixture of diastereomers. Subsequent Kornblum oxidation (NaHCO3, DMSO)16 of the diastereomeric bromides afforded the model analog anti-8 in 46% yield over two steps (Scheme 1).
Satisfied with the two-step benzylic oxidation, we tried to achieve the same transformation with the natural isomer syn-4, but our initial bromination reactions met with failure. No reaction was observed with a slight excess of NBS, and higher equivalents resulted in decomposition. A milder bromination alternative (NBS, SiCl4)17 was then investigated, but this also led to rapid decomposition of syn-4.
In contrast to syn-4, the more robust acetate syn-9 reacted smoothly with NBS to give diastereomeric bromides syn-10 as a nearly 1:1 mixture in 51% combined yield (Scheme 2a). It was found that two equivalents of NBS were necessary for the bromination reaction to go to completion and the reaction temperature could be lowered from reflux to 80 – 85 °C without affecting the reaction rate. Although syn,syn-10 and syn,anti-10 were separable by chromatography, this purification was unnecessary since both diastereomers are converted to the same compound in the subsequent Kornblum oxidation. Thus, treatment of the diastereomeric mixture of syn-10 with NaHCO3 in DMSO yielded the desired ketone syn-11, albeit in poor yield (17%). One reason for the low isolated yield was the ease of elimination of acetic acid from syn-11 to produce enone 12 (Scheme 2b), a reaction that was most likely facilitated by the increased acidity of C2 protons after introduction of the C3 carbonyl group. In order to prevent the formation of 12, the number of equivalents of base in the reaction mixture had to be carefully controlled. Utilizing a slight excess of NaHCO3 (1.1 – 1.2 equiv), syn-11 was obtained, but with higher equivalents, 12 was isolated as the sole product.
From syn-11, we hoped to access the final demethoxyviridin model syn-8 by simple deprotection of the acetate group. However, treatment of syn-11 with K2CO3/MeOH afforded a single product in excellent yield (88%) whose structure was determined to be anti-13 (Scheme 3). The formation of anti-13 from syn-11 was believed to proceed through enone 12, easily generated under the basic conditions. Subsequent conjugate addition of MeOH to the α,β-unsaturated ketone from the more accessible α-face would give anti-13. This hypothesis was tested by subjecting 12 to the deprotection reaction conditions, whereupon the reaction proceeded rapidly to give anti-13 as the sole product. The stereochemistry at C1 was deduced by carefully analyzing the proton NMR coupling constants, and was later confirmed through 1D NOE studies. Furthermore, an analogous conjugate addition to enones was described by Hanson and co-workers in their synthetic studies on demethoxyviridin.18
Based upon our experience with syn-9, we now re-investigated the bromination of syn-4, and gratifyingly, the reaction was successful with a slight excess of NBS in CCl4 at the lower reaction temperature of 85 °C. We also found that a catalytic amount of AIBN accelerated the reaction. Thus, the desired bromide syn-7 was obtained in 92% yield as an inseparable mixture of diastereomers, which was inconsequential for the subsequent stereoconvergent step. Finally, Kornblum oxidation afforded the desired demethoxyviridin model syn-8 in 33% isolated yield (Scheme 4), and no elimination was observed as in the case of acetate syn-9. The product was carefully characterized using spectroscopic methods, and the structure was confirmed by X-ray crystallography (Scheme 4).
In conclusion, the desired functionalization of ring A of the viridin core skeleton syn-4 was realized via a two-step bromination/Kornblum oxidation sequence to give demethoxyviridin analog syn-8. Although ketone formation was not achieved in a single step from syn-4, the final synthetic pathway gave valuable insight into the reactivity of ring A, and provided access to other compounds which might not have been synthesized otherwise.
EXPERIMENTAL
All reactions were carried out under nitrogen atmosphere with dry solvents in anhydrous conditions,
unless otherwise noted. Reagents were used as received without further purification unless otherwise noted. Dichloromethane was passed through a column of activated alumina under positive pressure of nitrogen prior to use. Carbon tetrachloride (≥99.5% anhydrous) was distilled before use. N-Bromosuccinimide was recrystallized from water. Melting points are uncorrected. Infrared spectra were recorded on NaCl plates either neat or as thin films prepared with CH2Cl2. 1H and 13C NMR spectra were recorded at 300 or 500 MHz. Resonances are reported in parts per million (ppm) downfield from TMS and were referenced to the residual solvent peak (1H; CDCl3: δ7.27; DMSO-d6: 2.50) or the solvent resonances (13C; CDCl3: δ77.23; DMSO-d6: 39.52). Data are reported as follows: (integration; multiplicity: s = singlet, d = doublet, t = triplet, q = quartet, m = multiplet; coupling constant (J) in Hertz (Hz)). Gas chromatography-mass spectrometry was performed on a Supelco SLB-5ms capillary column. Elemental analyses were performed by Atlantic Microlab Inc., and high resolution mass spectrometry was performed at the University of Illinois, Urbana-Champaign Mass Spectrometry Laboratory. X-Ray data collection was conducted at the Michigan State University Center for Crystallographic Research by Dr. Richard Staples.
(1R,10bR)-3-Bromo-2,3-dihydro-1-hydroxy-10b-methyl-1H-phenanthro[10,1-bc]furan-6(10bH)-one (syn-7). In a dry 25 mL rb flask, 87.0 mg (0.34 mmol, 1.0 equiv) of syn-4 (insoluble in CCl4) was dissolved in dry CH2Cl2 (4.0 mL), and then the flask was charged with dry CCl4 (4.0 mL). To the yellow solution was added 71.5 mg (0.40 mmol, 1.2 equiv) of NBS, followed by 11.9 mg (0.07 mmol, 0.2 equiv) of AIBN. The reaction mixture was heated to 85 °C under nitrogen, and reaction progress was monitored by TLC (1:1 hexanes:EtOAc). After 1 h, starting material was still present and a spatula tip of additional NBS was added. After heating for 3.5 h, the reaction mixture was cooled to rt and diluted with CH2Cl2. The organic layer was washed with saturated aqueous Na2S2O3 solution and the aqueous layer was back-extracted with CH2Cl2. The combined organic layers were washed with brine and dried over MgSO4 and concentration gave the crude product mixture as a brown oil. Purification by flash chromatography (20.3 g SiO2, 3:1 hexanes:EtOAc) afforded an inseparable mixture of diastereomeric bromide syn-7 as a yellow oil (104.3 mg, 92%). Rf = 0.48 (1:1 hexanes:EtOAc). Each diastereomer was characterized as its corresponding acetate.
(1R,10bR)-3-Bromo-2,3,6,10b-tetrahydro-10b-methyl-6-oxo-1H-phenanthro[10,1-bc]furan-1-yl acetate (10). To a solution of 46.6 mg (0.16 mmol, 1.0 equiv) of syn-9 in dry CCl4 (3.0 mL) and dry CH2Cl2 (1.1 mL) were added sequentially 68.5 mg (0.38 mmol, 2.4 equiv) of NBS and 5.5 mg (0.03 mmol, 0.2 equiv) of AIBN. The reaction mixture was heated at 81 °C under nitrogen until complete by TLC (1:1 hexanes:EtOAc). On completion (5 h), the mixture was cooled to rt and diluted with CH2Cl2. The organic layer was washed with saturated aqueous Na2S2O3 solution and the aqueous layer was back-extracted with CH2Cl2. The combined organic layers were washed with brine and dried over MgSO4. Concentration gave the diastereomeric mixture of bromides 10 as a yellow oil (69.5 mg). Separation by flash chromatography (17.2 g SiO2, 10:1 hexanes:EtOAc) afforded (syn, syn)-10 as pale yellow crystals (15.3 mg, 26%) and (syn, anti)-10 as a pale yellow solid (15.0 mg, 25%).
(Syn, syn)-10: Rf = 0.62 (1:1 hexanes:EtOAc). Mp 159 – 160 °C (dec.). 1H-NMR (500 MHz, CDCl3): 8.38 (1H, d, J = 8.5 Hz), 7.82 (1H, d, J = 1.0 Hz), 7.76 (1H, d, J = 8.0 Hz), 7.55 (1H, t, J = 8.0 Hz), 7.48 (1H, t, J = 8.5 Hz), 5.32 (1H, dt, J = 8.0 Hz, 1.0 Hz), 5.11 (1H, dd, J = 11.5 Hz, 4.5 Hz), 2.98 (1H, ddd, J = 14.5 Hz, 8.5 Hz, 4.5 Hz), 2.78 (1H, ddd, J = 14.5 Hz, 11.5 Hz, 8.5 Hz), 2.29 (3H, s), 1.78 (3H, s). 13C-NMR (500 MHz, CDCl3): 172.34, 169.90, 147.45, 146.91, 145.17, 142.44, 133.07, 132.83, 128.51, 127.89, 126.93, 123.73, 74.03, 40.02, 37.82, 32.96, 27.47, 21.67. IR (cm-1): 2926 (m), 1744 (s), 1675 (vs), 1598 (w), 1453 (m), 1427 (m), 1371 (m), 1228 (vs), 1024 (m), 914 (m), 766 (m), 629 (w). HRMS (EI) calc. for C18H15BrO4: 374.0154; found: 374.0148.
(Syn, anti)-10: Rf = 0.54 (1:1 hexanes:EtOAc). 1H-NMR (500 MHz, CDCl3): 8.37 (1H, d, J = 8.0 Hz), 7.79 (1H, d, J = 1.0 Hz), 7.74 (1H, d, J = 7.5 Hz), 7.54 (1H, t, J = 7.5 Hz), 7.48 (1H, t, J = 8.0 Hz), 5.65 (1H, dd, J = 10.5 Hz, 4.5 Hz), 5.56 (1H, ddd, J = 4.5 Hz, 2.0 Hz, 1.0 Hz), 2.84 (1H, ddd, J = 16.0 Hz, 10.5 Hz, 4.5 Hz), 2.69 (1H, ddd, J = 16.0 Hz, 4.5 Hz, 2.0 Hz), 2.31 (3H, s), 1.60 (3H, s). 13C-NMR (500 MHz, CDCl3): 172.48, 169.74, 148.10, 147.05, 142.09, 133.16, 132.87, 132.83, 128.61, 127.81, 126.36, 124.41, 71.68, 40.08, 37.94, 34.93, 28.66, 21.78.
Identification of the stereochemistry was determined by NOESY1D (500 MHz, CDCl3): (Syn, syn)-10 showed strong NOE signals between the methine CH (5.32 ppm, H3) and the CH (2.98 ppm, H2α), between the methine CH (5.32 ppm, H3) and the CH (5.11 ppm, H1), and between the methine CH (5.32 ppm, H3) and the furan CH (7.82 ppm, H5). (Syn, anti)-10 showed NOE signals between the methine CH (5.65 ppm, H3) and the CH3 (1.61 ppm, H10a), between the methine CH (5.65 ppm, H3) and the CH (2.70 ppm, H2α), between the methine CH (5.65 ppm, H3) and the CH (2.84 ppm, H2β), and between the methine CH (5.65 ppm, H3) and the furan CH (7.79 ppm, H5).
(1R,10bR)-1,2-Dihydro-1-hydroxy-10b-methyl-10bH-phenanthro[10,1-bc]furan-3,6-dione (syn-8). A solution of 90.0 mg (0.27 mmol, 1.0 equiv) of bromide syn-7 (diastereomeric mixture) in dry DMSO (5.0 mL) was prepared at rt under nitrogen, and 24.1 mg (0.29 mmol, 1.1 equiv) of NaHCO3 was added. The reaction mixture was stirred overnight at rt under nitrogen, then diluted with aqueous HCl solution and extracted twice with EtOAc. The combined organic extracts were washed with water and brine, then dried over MgSO4 and concentrated to a brown oil (81.7 mg). Purification by flash chromatography (13.3 g SiO2, 3:1 to 2:1 hexanes:EtOAc) afforded the title compound as a yellow solid (23.6 mg, 33%). Recrystallization from hexanes/CH2Cl2 produced crystals suitable for X-ray defraction. Rf = 0.27 (1:1 hexanes:EtOAc). 1H-NMR (500 MHz, DMSO-d6): 8.82 (1H, s), 8.44 (1H, d, J = 8.0 Hz), 8.19 (1H, d, J = 7.5 Hz), 7.68 (1H, t, J = 8.0 Hz), 7.53 (1H, t, J = 7.5 Hz), 6.07 (1H, d, J = 6.0 Hz), 4.34 (1H, ddd, J = 11.0 Hz, 6.0 Hz, 5.0 Hz), 3.06 (1H, dd, J = 18.0 Hz, 11.0 Hz), 2.73 (1H, dd, J = 18.0 Hz, 5.0 Hz), 1.55 (3H, s). 13C-NMR (500 MHz, DMSO-d6): 191.08, 172.31, 150.38, 149.68, 146.63, 146.04, 133.30, 132.82, 129.50, 127.90, 127.46, 123.97, 71.71, 47.25, 41.41, 25.76. IR (cm-1): 3437 (br w), 2924 (m), 1731 (s), 1673 (s), 1599 (w), 1528 (w), 1461 (w), 1267 (m), 1188 (m), 982 (w), 737 (s). HRMS (EI) calcd. for C16H12O4: 268.0736; found: 268.0728. Structure confirmed by X-ray. Deposition number CCDC-956572 for compound syn-8. Free copies of the data can be obtained via http://www.ccdc.cam.ac.uk/conts/retrieving.html.
(1R,10bR)-2,3,6,10b-Tetrahydro-10b-methyl-3,6-dioxo-1H-phenanthro[10,1-bc]furan-1-yl acetate (syn-11). A clear yellow solution of 7.0 mg (0.019 mmol, 1.0 equiv) of the diastereomeric mixture of bromides syn-10 in anhydrous DMSO (1.2 mL) was prepared at rt under nitrogen. To the solution was added 2.0 mg (0.024 mmol, 1.2 equiv) of NaHCO3. The reaction mixture was stirred at rt for 22 h, then diluted with water and acidified with 1 M aqueous HCl. The aqueous phase was extracted twice with Et2O and the combined organic extracts were washed with brine. After drying over MgSO4, concentration gave a brown oil (7.7 mg). Purification by preparative TLC (1:1 hexanes:EtOAc) gave syn-11 as a yellow solid (1.0 mg, 17%). Rf = 0.57 (1:1 hexanes:EtOAc). 1H-NMR (500 MHz, CDCl3): 8.43 (1H, d, J = 7.5 Hz), 8.27 (1H, s), 7.86 (1H, d, J = 8.0 Hz), 7.62 (1H, t, J = 7.5 Hz), 7.54 (1H, t, J = 8.0 Hz), 5.44 (1H, dd, J = 10.5 Hz, 5.5 Hz), 3.20 (1H, dd, J = 18.5 Hz, 5.5 Hz), 2.96 (1H, dd, J = 18.5 Hz, 10.5 Hz), 2.31 (3H, s), 1.75 (3H, s). IR (cm-1): 2924 (w), 1747 (s), 1704 (s), 1676 (vs), 1598 (w), 1529 (m), 1372 (w), 1227 (s), 1039 (m), 982 (m), 766 (w). HRMS (ESI) calcd. for C18H14O5: 311.0919; found: 311.0921 [M+H]+.
(1S,10bR)-1,2-Dihydro-1-hydroxy-10b-methyl-10bH-phenanthro[10,1-bc]furan-3,6-dione (anti-8). To a solution of 6.0 mg (0.023 mmol, 1.0 equiv) of anti-4 in anhydrous CCl4 (1.0 mL) was added 4.5 mg (0.025 mmol, 1.1 equiv) of NBS. The reaction mixture was heated at 110 °C in a sealed vial until complete by TLC (1:1 hexanes:EtOAc). On completion (5 h), the reaction mixture was cooled to rt and diluted with CH2Cl2. The organic layer was washed with 20% w/v aqueous Na2S2O3 solution and the aqueous layer was back-extracted with CH2Cl2. The combined organic layers were washed with brine and dried over MgSO4. Concentration gave the diastereomeric mixture of bromide anti-7 as a brown oil (6.4 mg). The crude material was dissolved in dry DMSO (1.0 mL) and 2.5 mg (0.03 mmol, 1.7 equiv) of NaHCO3 was added. The orange reaction mixture was stirred at rt overnight under nitrogen, then acidified with 1 M aqueous HCl solution and diluted with water. After extracting twice with Et2O, the organic extracts were washed with brine, dried over MgSO4 and concentrated to a brown oil (3.0 mg). Purification by preparative TLC (1:1 hexanes:EtOAc) afforded anti-8 as a pale yellow solid (2.2 mg, 46%). Rf = 0.22 (1:1 hexanes:EtOAc). 1H-NMR (500 MHz, CDCl3): 8.45 (1H, d, J = 7.5 Hz), 8.27 (1H, s), 7.71 (1H, t, J = 8.0 Hz), 7.64 (1H, d, J = 8.0 Hz), 7.56 (1H, t, J = 7.5 Hz), 5.02 (1H, dd, J = 3.0 Hz, 2.5 Hz), 3.16 (1H, dd, J = 18.5 Hz, 3.0 Hz), 2.98 (1H, dd, J = 18.5 Hz, 2.5 Hz), 1.64 (3H, s). 13C-NMR (500 MHz, CDCl3): 189.60, 172.30, 148.60, 145.28, 145.10, 134.63, 133.38, 129.49, 128.37, 127.66, 124.73, 122.14, 74.40, 44.26, 42.84, 30.91. IR (cm-1): 3430 (br w), 2919 (m), 1732 (s), 1671 (s), 1598 (w), 1528 (w), 1465 (w), 1181 (m), 973 (w), 735 (w). HRMS (EI) calcd. for C16H12O4: 268.0736; found: 268.0733.
(R)-10b-Methyl-10bH-phenanthro[10,1-bc]furan-3,6-dione (12). In a dry, nitrogen-flushed 10 mL rb flask, 13.7 mg (0.036 mmol, 1.0 equiv) of bromide syn-10 (diastereomeric mixture) was combined with 6.2 mg (0.074 mmol, 2.1 equiv) of NaHCO3. The flask was charged with dry DMSO (1.5 mL), and the resulting yellow reaction mixture was stirred at rt under nitrogen for 22 h. The reaction mixture was diluted with water and acidified with 1 M aqueous HCl solution. After extracting twice with Et2O, the organic layer was washed with brine and dried over MgSO4. Concentration gave a brown oil (6.6 mg). Purification by preparative TLC (1:1 hexanes:EtOAc) gave enone 12 as a yellow solid (2.4 mg, 27%). Rf = 0.53 (1:1 hexanes:EtOAc). 1H-NMR (500 MHz, CDCl3): 8.42 (1H, d, J = 7.5 Hz), 8.28 (1H, s), 7.74 (1H, d, J = 8.0 Hz), 7.70 (1H, d, J = 10.0 Hz), 7.67 (1H, t, J = 8.0 Hz), 7.53 (1H, t, J = 7.5 Hz), 6.39 (1H, d, J = 10.0 Hz), 1.74 (3H, s). 13C-NMR (500 MHz, CDCl3): 179.96, 172.24, 149.19, 148.10, 147.05, 145.82, 144.94, 133.47, 133.05, 131.05, 129.44, 128.03, 124.48, 122.13, 41.16, 40.31. IR (cm-1): 1662 (s), 1524 (m), 1458 (w), 1341 (w), 1181 (m), 1111 (w), 984 (m), 814 (m). HRMS (EI) calcd. for C16H10O3: 250.0630; found: 250.0628.
(1S,10bR)-1,2-Dihydro-1-methoxy-10b-methyl-10bH-phenanthro[10,1-bc]furan-3,6-dione (anti-13). Method A – In a clean dry 15 mL rb flask, 5.0 mg (0.016 mmol, 1.0 equiv) of syn-11 and 2.7 mg (0.019 mmol, 1.2 equiv) of K2CO3 were combined. The flask was charged with MeOH (1.5 mL) and distilled water (0.15 mL) and the bright orange reaction mixture was stirred at rt while monitoring by TLC (1:1 hexanes:EtOAc). On completion (2.5 h), the reaction mixture was acidified with a few drops of 1 M aqueous HCl solution, and MeOH was removed in vacuo. The aqueous residue was diluted with water and extracted twice with Et2O. The organic extracts were combined, washed with brine and dried over MgSO4. Concentration gave an orange oil which was purified by preparative TLC (1:1 hexanes:EtOAc) to afford methyl ether anti-13 as a yellow solid (4.0 mg, 88%). Rf = 0.42 (1:1 hexanes:EtOAc). 1H-NMR (500 MHz, CDCl3): 8.41 (1H, d, J = 7.5 Hz), 8.24 (1H, s), 7.65 (1H, t, J = 7.5 Hz), 7.56 (1H, d, J = 8.0 Hz), 7.51 (1H, t, J = 8.0 Hz), 4.45 (1H, dd, J = 3.0 Hz, 2.5 Hz), 3.19 (3H, s), 3.14 (1H, dd, J = 18.5 Hz, 2.5 Hz), 3.02 (1H, dd, J = 18.5 Hz, 3.0 Hz), 1.60 (3H, s). 13C-NMR (500 MHz, CDCl3): 189.84, 172.68, 148.42, 146.92, 145.38, 133.76, 132.82, 128.95, 127.59, 124.75, 122.28, 83.51, 57.88, 42.13, 40.39, 31.29. IR (cm-1): 2917 (w), 1698 (m), 1667 (s), 1528 (m), 1352 (w), 1184 (w), 1090 (m), 968 (m), 897 (w), 776 (w). HRMS (ESI) calcd. for C17H14O4: 283.0970; found: 283.0969 [M+H]+.
Identification of the stereochemistry was determined by NOESY1D (500 MHz, CDCl3): Anti-13 showed NOE signals between the methine CH (4.45 ppm, H1) and the CH3 (1.60 ppm, H10a), between the methine CH (4.45 ppm, H1) and the C2 methylene protons (3.02 ppm, 3.14 ppm, H2α and H2β), between the methine CH (4.45 ppm, H1) and the methyl ether CH3 (3.19 ppm, H17) and between the methine CH (4.45 ppm, H1) and the aromatic CH (7.56 ppm, H11).
(1S,10bR)-1,2-Dihydro-1-methoxy-10b-methyl-10bH-phenanthro[10,1-bc]furan-3,6-dione (anti-13). Method B – To a pale yellow solution of 2.4 mg (0.010 mmol, 1.0 equiv) of enone 12 in MeOH (0.5 mL) was added distilled water (50 µL), followed by 2.2 mg (0.016 mmol, 1.6 equiv) of K2CO3. The resulting bright pink reaction mixture was stirred at rt until complete by TLC (1:1 hexanes:EtOAc). On completion (55 min), the reaction mixture was acidified with a few drops of 1 M aqueous HCl solution, and MeOH was removed in vacuo. The aqueous residue was diluted with water and extracted twice with Et2O. The organic extracts were combined, washed with brine and dried over MgSO4. Concentration afforded methyl ether anti-13 as a yellow solid (2.0 mg, 78%).
ACKNOWLEDGEMENTS
Financial support of this work by the National Science Foundation, CHE-0646876, and Dartmouth College is gratefully acknowledged.
References
1. J. R. Hanson, Nat. Prod. Rep., 1995, 12, 381. CrossRef
2. (a) P. W. Brian, P. J. Curtis, H. G. Hemming, and G. L. F. Norris, Trans. Brit. Mycol. Soc., 1957, 40, 365; CrossRef (b) D. Wiesinger, H. U. Gubler, W. Haefliger, and D. Hauser, Experientia, 1974, 30, 135. CrossRef
3. P. Wipf and R. J. Halter, Org. Biomol. Chem., 2005, 3, 2053. CrossRef
4. G. Powis, R. Bonjouklian, M. M. Berggren, A. Gallegos, R. Abraham, C. Ashendel, L. Zalkow, W. F. Matter, J. Dodge, G. Grindey, and C. J. Vlahos, Cancer Res., 1994, 54, 2419.
5. B. H. Norman, J. Paschal, and C. J. Vlahos, Bioorg. Med. Chem. Lett., 1995, 5, 1183. CrossRef
6. M. P. Wymann, G. Bulgarelli-Leva, M. J. Zvelebil, L. Pirola, B. VanHaesebroeck, M. D. Waterfield, and G. Panayotou, Mol. Cell. Biol., 1996, 16, 1722.
7. R. Woscholski, T. Kodaki, M. McKinnon, M. D. Waterfield, and P. J. Parker, FEBS Lett., 1994, 342, 109. CrossRef
8. M. J. Cross, A. Stewart, M. N. Hodgkin, D. J. Kerr, and M. J. O. Wakelam, J. Biol. Chem., 1995, 270, 25352. CrossRef
9. E. H. Sessions and P. A. Jacobi, Org. Lett., 2006, 8, 4125. CrossRef
10. E. O. Onyango and P. A. Jacobi, J. Org. Chem., 2012, 77, 7411. CrossRef
11. E. H. Sessions, R. T. O’Connor Jr., and P. A. Jacobi, Org. Lett., 2007, 9, 3221. CrossRef
12. K. C. Mascall and P. A. Jacobi, Tetrahedron Lett., 2012, 53, 1620. CrossRef
13. K. C. Nicolaou, P. S. Baran, and Y.-L. Zhong, J. Am. Chem. Soc., 2001, 123, 3183. CrossRef
14. A. Shaabani, P. Mirzaei, S. Naderi, and D. G. Lee, Tetrahedron, 2004, 60, 11415. CrossRef
15. Initial Wohl−Ziegler bromination reactions were performed in the absence of a radical initiator.
16. N. Kornblum, W. J. Jones, and G. J. Anderson, J. Am. Chem. Soc., 1959, 81, 4113. CrossRef
17. T. A. Salama and Z. Novák, Tetrahedron Lett., 2011, 52, 4026. CrossRef
18. J. R. Hanson, M. A. O’Leary, H. J. Wadsworth, and B. L. Yeoh, J. Chem. Soc., Perkin Trans. 1, 1985, 1311. CrossRef