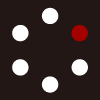
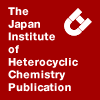
HETEROCYCLES
An International Journal for Reviews and Communications in Heterocyclic ChemistryWeb Edition ISSN: 1881-0942
Published online by The Japan Institute of Heterocyclic Chemistry
e-Journal
Full Text HTML
Received, 29th June, 2013, Accepted, 31st October, 2013, Published online, 22nd November, 2013.
DOI: 10.3987/COM-13-12764
■ A CU[I] CATALYZED MILD AND GENERAL SYNTHESIS OF 1,4-DISUBSTITUTED-1,2,3-TRIAZOLES FROM TERMINAL ACETYLENES AND IN SITU GENERATED ALKYL AZIDES
José Emilio de la Cerda-Pedro, Yoarhy Alejandro Amador-Sánchez, Mayra Cortés-Hernández, Jovana Pérez-Pérez, Susana Rojas-Lima,* and Heraclio López-Ruiz*
Chemistry Academic Area (AAQ), Autonomous University of Hidalog (UAEH), University City, Pachuca-Tulancingo Road Km 4.5, Mineral de la Reforma, Hidalogo, CP 42184, Mexico
Abstract
Cuprous cyanide, generated in situ from cupric sulfate and sodium cyanide, catalyzes the synthesis of 1,4-disubstituted-1,2,3-triazoles from in situ generated alkyl azides and mono-substituted alkynes in a one pot room temperature process.INTRODUCTION
The Cu(I)-catalyzed azide-alkyne cycloaddition reaction (CuAAC) has become the preferred synthetic route to 1,4-disubstituted 1,2,3-triazoles, some of which have interesting biological properties and/or applications in drug design.1-3 Because of the thermodynamic instability of Cu(I), Cu(II) is usually reduced in situ to Cu(I), by the addition of reducing agents, such as sodium ascorbate,4 glucose in the presence of Fehling’s reagent,5 or NaN3.6 Indeed, although longer reaction times are required when the Cu(II)/ascorbate process is used, we felt these finding had substantial implications and the potential for further development. In this article, we describe an efficient, room temperature synthesis of 1,4-disubstituted-1,2,3-triazoles from alkyl acetylenes and in situ generated alkyl azides, catalyzed by Cu(I)CN generated via the sodium cyanide reduction7 of CuSO4 (Scheme 1).
Although the generation of CuCN by means of the NaCN reduction of CuSO4 has been known for some time,7 as far as we are aware, Cu(I) generated in this manner has not been used to catalyze the formation of 1,4-disubstituted-1,2,3-triazoles from alkyl azides and mono-substituted acetylenes. We chose to investigate this very reasonable possibility.
RESULTS AND DISCUSSION
Optimization studies were carried out using phenylacetylene and benzyl azide (generated in situ from benzyl bromide and sodium azide) in the presence of varying amounts of CuSO4 and NaCN, at room temperature, in a 1:1:1.5 water:iPrOH:DMF solvent mixture (Table 1). The data clearly show that the best conditions for the formation of 3a involved the use of 36 mol% CuSO4 and 69 mol% NaCN (entry 9).
With the optimized conditions in hand, the scope of the reaction was explored by reacting various benzyl bromides (1a-c), alkyl bromides (1d and 1f) and allyl bromide (1e) with terminal alkynes (2a-j) in presence of NaN3. The results are summarized in Table 2. It is obvious that a wide variety of benzyl, and alkyl bromide possessing different functional groups reacted successfully. The reaction showed considerable tolerance for substituents in the phenylalkynes with both benzyl bromide (1a) and allyl bromide (1e) (Table 2). Both electron donating and attracting substituents in the phenylalkynes led to the desired products in high yields with little obvious differences in reaction times. In contrast, both the reaction of benzyl bromide with propargyl alcohol and ethyl bromoacetate with phenylacetylene gave rise to more modest yields of the expected products 3f and 3n, respectively.
The cuprous cyanide catalyzed cycloaddition process was also successfully applied to the azido compound 4 with phenylacetylene and three para substituted derivatives thereof (see Scheme 2). Compound 4 was prepared as described in the Experimental Section.
The structure of most of the 1,2,3-triazoles was supported by the usual spectroscopic data, and that of triazoles 3d, 3e, 3i, 3o and 5c was established unequivocally by X-ray crystallography (Figure 1).8
Experiments were conducted to show that cuprous cyanide generated in situ by the reduction of Cu(II) sulfate with NaCN both catalyzes the cycloaddition of azides with acetylenes, and is superior in this regard to commercial CuCN.25 Firstly, as expected, commercial CuCN catalyzes the cycloaddion of benzyl azide (6), both generated in situ (experiment a, Scheme 3) or ex situ (experiment c), to phenylacetylene in yields of 84 % and 73 %, respectively, after 12 h at room temperature. Secondly, CuSO4 fails to effect this cycloaddition with in situ generated benzyl azide under the same conditions (experiment b). Thirdly, in situ generation of CuCN from copper (II) sulfate and sodium cyanide in the presence of benzyl azide and phenylacetylene resulted in the formation of 3a in 95% yield in 30 min (experiment d).
A possible mechanism for the catalytic CuSO4/CuCN reaction is delineated in Figure 2.6,26
CONCLUSIONS
We describe in this letter a facile, room temperature, high yield, one pot preparation of 1,4-disubstituted 1,2,3-triazoles from organic azides, generated in situ, and various monosubstituted alkynes. The cuprous cyanide was generated in situ from copper(II) sulfate and sodium cyanide, the reactions time was reduced from 12h to 30 min giving the triazole in 95% yield. Considering the operational ease with which these reactions can be carried out, and the inexpensive chemicals involved, we believe this protocol will be of great benefit to medicinal and synthetic organic chemistry.
EXPERIMENTAL
TLC was performed on Merck-DC-F254 plates, detection was made by shining UV light. Flash column chromatography was performed using Merck silica gel (230-240 mesh). Melting points were measured in open capillary tubes on a Büchi Melting Point B-540 apparatus and have not been corrected. 1H and 13C NMR spectra were recorded on a Varian VNMRS 400 (400 and 100 MHz) spectrometers. Chemical shifts (δ) are indicated in ppm downfield from internal TMS used as reference; the coupling constants (J) are given in Hz. IR spectra were measured on a Perkin Elmer GX FT-IR. Elemental analyses were performed on a Perkin-Elmer Series II CHNS/O Analyzer 2400. High resolution mass spectra were obtained with an agilent G1969A spectrometer.
General procedure for the preparation of 1,2,3-triazoles (3).
In a 25 mL round-bottomed flask containing a magnetic stirring bar was placed 100 mg of (1a-f) in 3.5 mL of mixture of 1 mL of H2O, 1 mL of isopropanol and 1.5 mL of DMF as solvent, followed by the addition of 2 eq of NaN3, 0.36 eq of CuSO4, 0.69 eq of NaCN, 1.0 equiv. of alkyne (2a-h), and 1 mL of Et3N. The resulting solution was stirred at room temperature for the time show in the Table 2. Followed by extraction with EtOAc (3 x 20 mL). The collected organic layers were dried with MgSO4 and the solvent was removed under vacuum to give the corresponding triazole. In all cases the crude product was purified by recrystallization General procedure for the preparation of 1,2,3-triazoles.
Compound 3a, 3b, 3c, 3d, 3e, 3f, 3g, 3h, 3i, 3j, 3l, 3m, 3n and 3s are known, and their spectra are consistent with literatures show in the Table 2.
1,4-Bis((4-(4-methoxyphenyl)-1H-1,2,3-triazol-1-yl)methyl)benzene (3k): The general procedure was followed using 0.50 mL (0.38 mmol) of α,α’-dibromo-p-xylene (1b), 0.155 g (1.51 mmol) of 4-ethynylanisol (2d) and 0.099 g (1.52 mmol) of NaN3 to give 0.143 g (83% yield) of 3k as white solid; mp 239-241 °C; IR (film) νmax/cm-1: 3121, 2101, 1614, 1499, 1251, 819; 1H NMR (DMSO-d6, 400 MHz) δ/ppm: 8.61(s, 2H), 7.79 (d, J = 8.8 Hz, 4H), 7.41 (s, 4H), 7.03(d, J = 8.8 Hz, 4H), 5.68 (s, 4H), 4.47 (s, 6H). 13C NMR (DMSO-d6, 100 MHz) δ/ppm: 159.3, 146.9, 136.4, 129.3, 128.6, 126.9, 123.6, 114.7, 55.6, 53.6. Anal. Calcd for C26H24N6O2: C, 69.01; H, 5.35; N, 18.57. Found C, 69.35; H, 5.14; N, 18.65.
1-Allyl-4-(4-cyanophenyl)-1H-1,2,3-triazole (3o): The general procedure was followed using 0.071 mL (0.83 mmol) of allyl bromide (1e), 0.105 g (1.51 mmol) of 4-ethynylbenzonitrile (2c) and 0.106 g (1.62 mmol) of NaN3 to give 0.147 g (85% yield) of 3o as yellow solid; mp 103-105 °C; IR (film) νmax/cm-1: 3132, 2221, 1612, 1233, 726, 553; 1H NMR (CDCl3, 400 MHz) δ/ppm: 7.92 (d, J = 8.8 Hz, 2H) 7.85 (s, 1H), 7.68 (d, J = 8.4 Hz, 2H), 6.04 (ddt, J = 6.4 Hz, J = 10.0 Hz, J = 17.2 Hz, 1H), 5.38 (m, 2H), 5.03 (d, J = 6.4 Hz, 2H). 13C NMR (CDCl3, 100 MHz) δ/ppm: 146.2, 134.9, 132.7, 130.9, 126.0, 120.7, 120.5, 118.8, 111.5, 52.9. Anal. Calcd for C12H10N4: C, 68.56; H, 4.79; N, 26.65. Found: C, 68.48; H, 4.84; N, 26.67%.
1-Allyl-4-(4-aminophenyl)-1H-1,2,3-triazole (3p): The general procedure was followed using 0.071 mL (0.83 mmol) of allyl bromide (1e), 0.097 mL (0.83 mmol) of 4-ethynylaniline (2i) and 0.108 g (1.66 mmol) of NaN3 to give 0.121 g (73% yield) of 3p as brown solid mp 110-112 °C; IR (film) νmax/cm-1: 3442, 3313, 2232, 1617, 1503, 801; 1H NMR (CDCl3, 400 MHz) δ/ppm: 7.53 (s, 1H) 7.50 (d, J = 8.4 Hz, 2H), 6.61 (d, J = 8.4 Hz, 2H), 5.92 (ddt, J = 6.4 Hz, J = 10.0 Hz, J = 19.2 Hz, 1H), 5.25-5.17 (m, 2H), 4.86 (dt, J = 1.6 Hz, J = 6.4 Hz, 2H), 3.78 (a, 2H). 13C NMR (CDCl3, 100 MHz) δ/ppm: 148.3, 146.6, 131.4, 126.8, 120.8, 119.9, 118.3, 115.2, 52.6. Anal. Calcd for C11H12N4: C, 65.98; H, 6.04; N, 27.98. Found: C, 66.00; H, 6.13; N, 27.72%.
4-(4-Chlorophenyl)-1-ethyl-1H-1,2,3-triazole (3q): The general procedure was followed using 0.068 mL (0.92 mmol) of bromoethane (1f), 0.125 g (0.92 mmol) of 1-chloro-4-ethynylbenzene (2e) and 0.120 g (1.85 mmol) of NaN3 to give 0.145 g (76% yield) of 3q as yellow solid; mp 101-103 °C; IR (film) νmax/cm-1: 3116, 1637, 1459, 1094, 929, 525, 506; 1H NMR (CDCl3, 400 MHz) δ/ppm: 7.68 (m, 3H) 7.31 (d, J = 9.2 Hz, 2H), 4.38 (q, J = 7.6 Hz, 2H) 1.52 (t, J = 7.6 Hz, 3H). 13C NMR (CDCl3, 100 MHz) δ/ppm: 146.7, 133.7, 129.1, 128.9, 126.8, 119.0, 45.4, 15.5. Anal. Calcd for C10H10ClN3: C, 57.84; H, 4.85; N, 20.24. Found: C, 58.16; H, 4.88; N, 20.38%.
1-Allyl-4-(4-methoxyphenyl)-1H-1,2,3-triazole (3r): The general procedure was followed using 0.071 mL (0.83 mmol) of allyl bromide (1e), 0.108 mL (0.83 mmol) of 4-ethynylanisole (2d) and 0.108 g (1.62 mmol) of NaN3 to give 0.159 g (89% yield) of 3r as white solid; mp 89-91 °C; IR (film) νmax/cm-1: 3099, 2934, 1618, 1502, 1249, 1031, 821, 528; 1H NMR (CDCl3, 400 MHz) δ/ppm: 7.64 (d, J = 9.2 Hz, 2H), 7.57 (s, 1H), 6.84 (d, J = 8.8 Hz, 2H), 5.95 (ddt, J = 6.4 Hz, J = 10.0 Hz, J = 17.2 Hz, 1H), 5.25-5.19 (m, 2H), 4.89 (dt, J = 1.2 Hz, J = 6.4 Hz, 2H), 3.73 (s, 3H,). 13C NMR (CDCl3, 100 MHz) δ/ppm: 159.5, 147.8, 131.4, 129.6, 123.3, 120.1, 118.7, 114.1, 55.3, 52.7. Anal. Calcd for C12H13N3O C, 66.96; H, 6.09; N, 19.52. Found: C, 67.31; H, 6.06; N, 19.14%.
1-Allyl-4-(phenoxymethyl)-1H-1,2,3-triazole (3t): The general procedure was followed using 0.143 mL (1.65 mmol) of allyl bromide (1e), 0.168 g (1.27 mmol) of (prop-2-yn-yloxy)benzene (2h) and 0.214 g (3.28 mmol) of NaN3 to give 0.28 g (87% yield) of 3t as brown oil; (3t) IR (film) νmax/cm-1: 2924, 1738, 1600, 1491, 1226, 1010, 757; 1H NMR (CDCl3, 400 MHz) δ/ppm: 7.49 (s, 1H), 7.13-7.09 (m, 2H), 6.84-6.80 (m, 3H), 5.81 (ddt, J = 6.4 Hz, J = 10.4 Hz, J = 19.2 Hz, 1H), 5.16-5.06 (m, 2H), 5.00 (s, 2H), 4.75 (dt, J = 1.6 Hz, J = 6.4 Hz, 2H). 13C NMR (CDCl3, 100 MHz) δ/ppm: 153.1, 144.7, 131.2, 129.5, 122.9, 121.1, 120.0, 114.7, 61.7, 52.6. Anal. Calcd for C12H13N3O: C, 66.96; H, 6.09. Found: C, 67.01; H, 6.31%.
5-Azido-2-(2-methoxyphenyl)benzoxazole (4)
In a 250 mL hydrogenation flask was placed 300 mg of 5-nitro-2-(2-methoxyphenyl)benzoxazole,24 60 mg of Pd/C and 25 mL of CH2Cl2. The flask was pressurized to 60 psi of H2 for 5 h. The reaction mixture was filtered over Celite and concentrated in a rotary evaporator to afford the corresponding amine in 85% yield (227 mg). This product was transfer to a 100 mL of round bottom flask with a magnetic bar and 10 mL of HCl (10%). The resulting solution was allowed to react for 45 min before the addition of 0.11 g (1.5 equiv) of NaN3 and the reaction mixture was stired at room temperature fo 2 h. The reaction was quenched with brine and the product was extracted with EtOAc (3 x 25 mL). The collected organic layers were dried with Na2SO4 and the solvent was removed under vacuum to give 0.27 g (92%) of 5-azido-2-(2-methoxyphenyl)benzoxazole (4) as yellow solid; mp 98-99 °C; IR (CH2Cl2) νmax/cm-1: 2118, 3082, 1631, 1265; 1H NMR(CDCl3, 400 MHz) δ/ppm: 8.13 (ddd, J = 7.5 Hz, J = 2.3 Hz, J = 0.5 Hz, 1H), 7.55 (dd, J = 8.6 Hz, J = 0.6 Hz, 1H), 7.53 (m, 1H), 7.53 (dd, J = 2.3 Hz, J = 0.5 Hz, 1H), 7.11 (m, 1H), 7.17 (d, J = 7.5 Hz, 1H), 7.01 (dd, J = 8.6 Hz, J = 2.3 Hz, 1H), 4.01 (s, 3H). 13C NMR (CDCl3, 100 MHz) δ/ppm: 163.3, 158.5, 148.0, 143.4, 136.9, 133.3, 131.5, 120.9, 116.6, 115.9, 112.3, 111.4, 110.3, 56.4. Anal. Calcd for C14H10N4O2: C, 63.15; H, 3.79. Found: C, 63.38; H, 4.06%.
General procedure for the preparation of 1,2,3-triazoles (5a-d).
In a 50 mL round-bottomed flask containing a magnetic stirring bar was placed 133 mg (1 eq.) of 5-azido-2-(2-methoxyphenyl)benzoxazole (4) in 3.5 mL of mixture of 1 mL of H2O, 1 mL of isopropanol and 1.5 mL of DMF as solvent, followed by the addition of 1 eq of alkyne (2a,b,d,e), 0.36 eq of CuSO4, 0.69 eq of NaCN, and 1.5 mL of Et3N. The resulting solution was stirred at room temperature for 24 h. Followed by extraction with EtOAc (3 x 25 mL). The collected organic layers were dried with MgSO4 and the solvent was removed under vacuum to give the corresponding triazole. After removal of the solvent, the crude residue was purified by column chromatography (EtOAc/hexane) to afford 1,2,3-triazoles 5a-d.
2-(2-Methoxyphenyl)-5-(4-phenyl-1H-1,2,3-triazol-1-yl)benzoxazole (5a): The general procedure was followed using 0.333 g (0.5 mmol) of 5-azido-2-(2-methoxyphenyl)benzoxazole (4) and 0.055 mL (0.5 mmol) of phenylacetylene (2a) to give 0.123 g (67% yield) of 5a as orange solid; mp 132-133 °C; IR followed using 0.333 g (0.5 mmol) of 5-azido-2-(2-methoxyphenyl)benzoxazole (4) and 0.055 mL (0.5 mmol) of phenylacetylene (2a) to give 0.123 g (67% yield) of 5a as orange solid; mp 132-133 °C; IR (CH2Cl2) νmax/cm-1: 2938, 2838, 1656, 1607, 1498, 1478, 1263, 1026, 766, 756, 964; 1H NMR(CDCl3, 400 MHz) δ/ppm: 8.15 (s, 1H), 8.09 (m, 2H), 7.86 (d, J = 7.4 Hz, 2H), 7.76 (d, J = 8.6 Hz, 1H), 7.65 (d, J = 8.6), 7.48 ( t, J = 7.5 Hz, 1H), 7.40 (t, J = 7.5 Hz, 2H), 7.30 (t, J = 7.3 Hz, 1H), 7.05 (t, J = 8.7 Hz, 2H), 3.97 (s, 3H). 13C NMR (CDCl3, 100 MHz) δ/ppm: 163.6, 158.6, 150.1, 148.4, 143.0, 134.0, 133.5, 131.4, 130.1, 128.9, 128.4, 125.8, 120.8, 118.3, 118.2, 115.3, 112.4, 112.1, 111.4, 56.2. HRMS (ESI-TOF) m/z calcd for C22H16N4O2 [M+H]+: 369.1307, found m/z 369.1348.
5-(4-(4-Chlorophenyl)-1H-1,2,3-triazol-1-yl)-2-(2-methoxyphenyl)benzoxazole (5b): The general procedure was followed using 0.333 g (0.5 mmol) of 5-azido-2-(2-methoxyphenyl)benzoxazole (4) and 0.068 g (0.5 mmol) of 1-cloro-4-etynylbenzene (2e) to give 0.170 g (85% yield) of 5b as white solid; mp 213-214 °C; IR (CH2Cl2) νmax/cm-1: 2926, 2842, 1621, 1604, 1588, 1551, 1483, 1311, 1271, 1040, 795, 753; 1H NMR (DMSO-d6, 400 MHz) δ/ppm: 9.35 (s, 1H), 8.32 (dd, J = 2.0 Hz, J = 0.7 Hz, 1H), 8.05 (d, J = 1.8 Hz, 1H), 8.03 (d, J = 1.7 Hz, 1H), 8.01 (d, J = 0.7 Hz, 1H), 7.99 (d, J = 0.7 Hz, 1H), 7.97 (d, J = 2.1 Hz, 1H), 7.95 (d, J = 2.1 Hz, 1H), 7.94 (t, J = 2.1 Hz, 1H), 7.93 – 7.91 (m, 1H), 7.61 (ddd, J = 8.5 Hz, J = 7.3 Hz, 1.8 Hz, 1H), 7.57–7.55 (m, 1H), 7.55–7.53 (m, 1H), 7.27 (d, J = 7.9 Hz, 1H), 7.14 (td, J = 7.6 Hz, 1.0 Hz, 1H), 3.92 (s, 2H). 13C NMR (DMSO-d6, 400 MHz) δ/ppm: 163.8, 158.6, 150.2, 146.7, 142.5, 134.3, 134.1, 133.1, 131.6, 129.5, 127.4, 121.2, 121.0, 118.4, 115.2, 113.3, 112.4, 112.0, 56.5. HRMS (ESI-TOF) m/z calcd for C22H15ClN4O2 [M+H]+: 403.0962, found m/z 403.0958.
2-(2-Methoxyphenyl)-5-(4-(4-methoxyphenyl)-1H-1,2,3-triazol-1-yl)benzoxazole (5c): The general procedure was followed using 0.333 g (0.5 mmol) of 5-azido-2-(2-methoxyphenyl)benzoxazole (4) and 0.065 mL (0.5 mmol) of 4-ethynylanisol (2d) to give 0.169 g (85% yield) of 5c as red solid; mp 172-173 °C; IR (CH2Cl2) νmax/cm-1: 2938, 2842, 1617, 1601, 1548, 1495, 1484, 1251, 1178, 1028, 834, 792, 750, 702; 1H NMR (CDCl3, 400 MHz) δ/ppm: 8.07 (dd, J = 7.9 Hz, J = 1.7 Hz, 1H), 8.12 (m, 1H), 8.11 (s, 1H), 7.84 (m, 1H), 7.82 (m, 1H), 7.79 (dd, J = 8.0 Hz, J = 2.2 Hz, 1H), 7.68 (d, J = 8.8 Hz, 1H), 7.52 (ddd, J = 8.3 Hz, J = 7.4 Hz, J = 1.8 Hz, 1H), 7.12-7.07 (m, 2H), 6.98 (m, 1H), 6.96 (m, 1H), 4.02 (s, 3H), 3.83 (s, 3H). 13C NMR (CDCl3, 100 MHz) δ/ppm: 163.9, 160.0, 158.9, 150.3, 148.6, 143.2, 134.3, 133.7, 131.7, 127.4, 123.1, 121.1, 118.4, 117.6, 115.6, 114.6, 112.6, 112.4, 111.6, 56.5, 55.6. HRMS (ESI-TOF) m/z calcd for C23H18N4O3 [M+H]+: 399.1457, found m/z 399.1452.
5-(4-(4-Fluorophenyl)-1H-1,2,3-triazol-1-yl)-2-(2-Methoxyphenyl)benzoxazole (5d): The general procedure was followed using 0.333 g (0.5 mmol) of 5-azido-2-(2-methoxyphenyl)benzoxazole (4) and 0.057 mL (0.5 mmol) of 1-ethynyl-4-fluorobenzene (2d) to give 0.133 g (67% yield) of 5d as white solid; mp 223-225 °C; IR (CH2Cl2) νmax/cm-1: 2929, 2847, 1654, 1601, 1495, 1483, 1265, 1036, 1024, 789, 750, 711; 1H NMR (CDCl3, 400 MHz) δ/ppm: 8.18 (dd, J = 8.0 Hz, J = 1.8 Hz, 1H), 8.18, (s, 1H), 8.14 (d, J = 2.1 Hz, 1H), 7.94-7.88 (m, 2H), 7.84 (dd, J = 8.7 Hz, J = 2.2 Hz, 1H), 7.74 (d, J = 8.7 Hz, 1H), 7.56 (ddd, J = 8.3 Hz, J = 7.5 Hz, J = 1.8 Hz, 1H), 7.20 (m, 4H), 4.06 (s, 3H). 13C NMR (CDCl3, 100 MHz) δ/ppm: 164.4, 164.1, 162.0, 159.0, 150.5, 148.0, 143.4, 134.3, 133.9, 131.8, 128.0, 127.9, 126.7, 121.2, 118.7, 118.3, 116.4, 116.2, 115.7, 112.9, 112.5, 111.8. 56.6. HRMS (ESI-TOF) m/z calcd for C22H15FN4O2 [M+H]+: 187.1257, found m/z 387.1253.
ACKNOWLEDGEMENTS
We are indebted to Dr. Joseph M. Muchowski for friendly, helpful discussions and to CONACyT for financial support (grant CB-2009-01-135172).
References
1. For special issue to application of click chemistry themed, ed. by M. G. Finn and V. V. Fokin, Chem. Soc. Rev., 2010, 39, 1231. CrossRef
2. (a) M. Melda and C. W. Tornøe, Chem. Rev., 2008, 108, 2952; CrossRef (b) V. D. Bock, H. Hiemstra, and J. H. van Maarseveen, Eur. J. Org. Chem., 2006, 51; CrossRef (c) V. V. Rostovtsev, L. G. Green, V. V. Fokin, and K. B. Sharpless, Angew. Chem. Int. Ed., 2002, 41, 2596; CrossRef (d) C. W. Tornøe, C. Christensen, and M. Meldal, J. Org. Chem., 2002, 67, 3057. CrossRef
3. (a) W. G. Lewis, L. G. Green, F. Grynszpan, Z. Radiӕ, P. R. Carlier, P. Taylor, M. G. Finn, and K. B. Sharpless, Angew. Chem. Int. Ed., 2002, 41, 1053;; CrossRef (b) G. C. Tron, T. Pirali, R. A. Billington, P. L. Canonico, G. Sorba, and A. A. Genazzani, Med. Res. Rev., 2008, 28, 278; CrossRef (c) T. Lee, M. Cho, S.-Y. Ko, H.-J. Youn, D. J. Baek, W.-J. Cho, C.-Y. Kang, and S. Kim, J. Med. Chem., 2007, 50, 585; CrossRef (d) S. K. Maurya, D. R. Gollapalli, S. Kirubakaran, M. Zhang, C. R. Johnson, N. N. Benjamin, L. Hedstrom, and G. D. Cuny, J. Med. Chem., 2009, 52, 4623; CrossRef (e) H. C. Kold and K. B. Sharpless, Drug Discovery Today, 2003, 8, 1128; CrossRef (f) F. Puig-Basagoiti, M. Qing, H. Dong, B. Zhang, G. Zou, Z.Yuan, and P.-Y. Shi, Antiviral Res., 2009, 83, 71; CrossRef (g) R. Guezguez, K. Bougrin, K. E. Akri, and R. Benhida, Tetrahedron Lett., 2006, 47, 4807. CrossRef
4. C.-Z. Tao, X. Cui, J. Li, A.-X. Liu, L. Liu, and Q.-X. Guo, Tetrahedron Lett., 2007, 48, 3525. CrossRef
5. M. A. García, Z. G. Ríos, J. Gonzáles, V. M. Pérez, N. Lara, A. Fuentes, C. Gonzáles, D. Corona, and E. Cuevas-Yañes, Lett. Org. Chem., 2011, 8, 701. CrossRef
6. S. Mohammed, A. K. Padala, B. A. Dar, B. Singh, B. Sreedhar, R. A. Vishwakarma, and S. B. Bharate, Tetrahedron, 2012, 68, 8156. CrossRef
7. (a) A. F. Holleman and E. Wiberg, Inorganic Chemistry, Academic Press, 1995, p. 1256; (b) B. J. Hathaway, Comprehensive Coordination Chemistry, ed. by G. Wilkinson, Vol. 5, Pergamon Press, Oxford, 1987, p. 729; (c) S. F. Huang, H. H. Wei, and Y. Wang, Polyhedron, 1997, 16, 1747. CrossRef
8. Crystallographic data (excluding structure factors) for the structures in this paper has been deposited with the Cambridge Crystallographic Data Centre as a Supplementary Publication Numbers, CCDC 924280 No. for 3d, CCDC 924282 No. for 3e, CCDC 924279 No. for 3i, CCDC 924281 No. for 3o, and CCDC 925540 No for 5c. Copy of the data can be obtained, free of charge, on application to CCDC, 12 Union Road, Cambridge CB2 1EZ, UK [fax: +44(0) 1223 336033 or e-mail: deposit@ccdc.cam.ac.uk].
9. (a) H. López-Ruiz, J. E. de la Cerda-Pedro, S. Rojas-Lima, I. Pérez-Pérez, B. V. Rodríguez-Sanchez, R. Santillan, and O. Coreño, ARKIVOC, 2013, iii, 139; (b) T. R. Chan, R. Hilgraf, K. B. Sharpless, and V. V. Fokin, Org. Lett., 2004, 6, 2853; CrossRef (c) B. H. Lipshutz and B. R. A. Taft, Angew. Chem. Int. Ed., 2006, 45, 8235; CrossRef (d) F. Alonso, Y. Moglie, G. Radivoy, and M. Yus, Adv. Synth. Catal., 2010, 352, 3208; CrossRef (e) V. Bénéteau, A. Olmos, T. Boningari, J. Sommer, and P. Pale, Tetrahedron Lett., 2010, 51, 3673; CrossRef (f) T. Suzuka, K. Ooshiro, and K. Kina, Heterocycles, 2010, 81, 601; CrossRef (g) C. Shao, X. Wang, J. Xu, J. Zhao, Q. Zhang, and Y. Hu, J. Org. Chem., 2010, 75, 7002; CrossRef (h) I. Jlalia, H. Elamari, F. Meganem, J. Herscovici, and C. Girard, Tetrahedron Lett., 2008, 49, 6756; CrossRef (i) P. Li, L. Wang, and Y. Zhang, Tetrahedron, 2008, 64, 10825; CrossRef (j) S. Díez-González and S. P. Nolan, Angew. Chem. Int. Ed., 2008, 47, 8881; CrossRef (k) A. Feldman, B. Colasson, and V. V. Fokin, Org. Lett., 2004, 6, 3897; CrossRef (l) H. López-Ruiz, M. Cortés-Hernández, S. Rojas-Lima, and H. Höpfl, J. Mex. Chem. Soc., 2011, 55, 168; (m) L. Durán Pachon, J. H. van Maarseveen, and G. Rothenberg, Adv. Synth. Catal., 2005, 347, 811. CrossRef
10. H. Sharghi, R. Khalifeh, and M. M. Doroodmand, Adv. Synth. Catal., 2009, 351, 207. CrossRef
11. C. T. Lee, S. Huang, and B. H. Lipshutz, Adv. Synth. Catal., 2009, 351, 3139. CrossRef
12. C. Shao, R. Zhu, S. Luo, Q. Zhang, X. Wang, and Y. Hu, Tetrahedron Lett., 2011, 52, 3782. CrossRef
13. C. Shao, X. Wang, J. Xu, J. Zhao, Q. Zhang, and Y. Hu, J. Org. Chem., 2010, 75, 7002. CrossRef
14. L. Harman, M. H. Lescure, N. Candelon, M. Duttine, D. Lastécouères, and J. M. Vincent, Tetrahedron Lett., 2012, 53, 1417. CrossRef
15. K. Namitharan, M. Kumarraja, and K. Pitchumani, Chem. Eur. J., 2009, 15, 2755. CrossRef
16. Y. Wang, J. Liu, and C. Xia, Adv. Synth. Catal., 2011, 353, 1534. CrossRef
17. H. A. Orgueira, D. Fokas, Y. Isome, P. Chan, and C. Baldino, Tetrahedron Lett., 2005, 46, 2911. CrossRef
18. I. Jlalia, H. Elamari, F. Meganem, J. Herscovici, and C. Girard, Tetrahedron Lett., 2008, 49, 6756. CrossRef
19. F. Alonso, Y. Moglie, G. Radivoy, and M. Yus, Tetrahedron Lett., 2009, 50, 2358. CrossRef
20. F. Alonso, Y. Moglie, G. Radivoy, and M. Yus, Org. Biomol. Chem., 2011, 9, 6385. CrossRef
21. H. A. Stefani, H. A. Canduzini, and F. Manarin, Tetrahedron Lett., 2011, 52, 6086. CrossRef
22. M. A. García, J. Gonzáles, J. Trujillo-Reyes, R. A. Morales-Luckie, V. Sánchez-Mendieta, C. Gonzáles, A. Fuentes, and E. Cuevas-Yañes, Lett. Org. Chem., 2012, 9, 160. CrossRef
23. Y. M. A. Yamada, S. M. Sarkar, and Y. Uozumi, J. Am. Chem. Soc., 2012, 134, 9285. CrossRef
24. (a) H. López-Ruiz, H. Briseño-Ortega, S. Rojas-Lima, R. Santillán, and N. Farfán, Tetrahedron Lett., 2010, 51, 2633; CrossRef (b) H. López-Ruiz, H. Briseño-Ortega, S. Rojas-Lima, R. Santillán, and N. Farfán, Tetrahedron Lett., 2011, 52, 4308. CrossRef
25. (a) M. Liu and O. Reiser, Org. Lett., 2011, 13, 1102; CrossRef (b) G. A. Malander and J. Ham, Org. Lett., 2006, 8, 2767; CrossRef (c) Y.-B. Zhao, Z.-Y. Yan, and Y.-M. Liang, Tetrahedron Lett., 2005, 47, 1545; CrossRef (d) Z.-Y. Yan, Y.-B. Zhao, M.-J. Fan, W.-M. Liu, and Y.-M. Liang, Tetrahedron, 2005, 61, 9331; CrossRef (e) Z. Gonda and Z. Novák, Dalton Trans., 2010, 39, 726. CrossRef
26. B. T. Worrell, J. A. Malik, and V. V. Fokin, Science, 2013, 340, 457. CrossRef