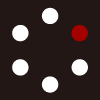
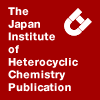
HETEROCYCLES
An International Journal for Reviews and Communications in Heterocyclic ChemistryWeb Edition ISSN: 1881-0942
Published online by The Japan Institute of Heterocyclic Chemistry
e-Journal
Full Text HTML
Received, 30th June, 2013, Accepted, 31st July, 2013, Published online, 14th August, 2013.
DOI: 10.3987/COM-13-S(S)70
■ Formation of Unexpected Heterocyclic Products from Pyrolysis of Thiocarbonyl Stabilised Phosphonium Ylides
R. Alan Aitken,* Graeme Barker, Lee P. Cleghorn, Euan J. Reid, and Sheryl S. Roberts
School of Chemistry, University of St. Andrews, North Haugh, St Andrews, Fife KY 16 9ST, U.K.
Abstract
Chiral phthalimido thioxo-stabilised phosphonium ylides, prepared starting from (S)-alanine and (S)-phenylalanine, undergo intramolecular Wittig reaction upon pyrolysis leading to the previously unknown pyrrolo[2,1-a]isoindol-5-one-2-thiones, rather than the expected P to S migration of a phenyl group. Pyrolysis of thioxo-stabilised ylides with three different groups on phosphorus gave some evidence of the required migration, but led in one case to unexpected formation of a 1,4-benzoxaphosphininium salt.INTRODUCTION
Thermal extrusion of Ph3PO from oxo-stabilised phosphonium ylides 1 provides a useful route for synthesis of substituted alkynes 2,1 and has recently been used to access such diverse systems as acetylenic amino acid derivatives,2 rings fused carbazoles,3 and polycyclic thieno[2,3-b]pyridine derivatives (Scheme 1).4 In contrast, the corresponding reaction of thioxo-stabilised ylides 3 has been much less studied, but we recently reported that, while most such compounds undergo extrusion of Ph3PS to give alkynes 2, those with R1 = H instead undergo rearrangement with transfer of one phenyl group to afford the Z-1-sulfanyl-2-phosphanylalkenes 4.5 Since these were shown to be potentially useful hemi-labile ligands for transition metals, we have investigated the formation of chiral analogues which might be useful in asymmetric catalysis. In this paper, two separate approaches are described: pyrolysis of thioxo-stabilised ylides bearing an amino acid-derived chiral auxiliary, and pyrolysis of thioxo-stabilised ylides derived from P-stereogenic phosphines. In each case unexpected thermal processes intervened leading to unusual and interesting heterocyclic products.
RESULTS AND DISCUSSION
The first approach was based on the fact that suitably N-protected amino acid derivatives can be used to acylate phosphonium ylides and the resulting oxo-stabilised ylides undergo thermal extrusion of Ph3PO to give chiral products without significant racemisation. Thus, we showed previously that N-alkoxycarbonyl ylides 5 could be prepared and converted into the alkynes 6 using flash vacuum pyrolysis (FVP) at 600 °C (Scheme 2).2,6 Cyclic amino acid-derived stabilised ylides were also prepared similarly.7 In an earlier study, the phthalimidoacyl ylides 7 were prepared, but unexpectedly they underwent an intramolecular Wittig reaction upon FVP at 500 °C to give the pyrroloisoindolediones 8, while at the higher temperature of 750 °C, the ester group was lost to afford products 9.8 We have also observed the competitive intramolecular extrusion of Ph3PO between an ylide function and β and δ carbonyl groups, leading in the latter case to transient formation of a cyclobutene.9,10
Although it was expected that the corresponding thioxo-stabilised ylides would show similar behaviour, no such compounds appear to have been reported before. Our planned synthesis relied on the fact that stabilised ylides bearing an α-tert-butoxycarbonyl group undergo ready loss of isobutene and CO2 upon treatment with p-toluenesulfonic acid.11 We thus started by reacting two equivalents of tert-butyl triphenylphosphoranylideneacetate 10 with the phthalimidoacyl chlorides 11 and 12, prepared, respectively, by successive treatment of (S)-alanine and (S)-phenylalanine with phthalic anhydride followed by thionyl chloride (Scheme 3). This gave the expected ylide products 13 and 14 as stable crystalline solids in low to moderate yield after chromatographic purification. As for all ylides described in this paper, their structure was readily confirmed from the highly informative 13C NMR spectra with phosphorus coupling to almost all signals. The removal of the tert-butoxycarbonyl groups was now carried out using the reported method,11 but replacing benzene with the less harmful toluene, to give the ylides 15 and 16 in moderate to good yield. In our previous work,5 conversion of the C=O into C=S was achieved directly by treatment with Lawesson's reagent,12 however for these more sensitive compounds this was not suitable. Instead we reverted to the two-step method of Bestmann,13 involving reaction with trifluoromethanesulfonic anhydride to form the O-sulfonylphosphonium triflate, then evaporation and treatment of the residue in situ with sodium sulfide in aqueous ethanol. This afforded the target thioxo-stabilised ylides 17 and 18 in acceptable yield.
These were now subjected to FVP using a temperature of 650 °C and a pressure of 10–2 Torr leading to complete reaction and collection of solid products in the cold trap, which proved in each case to be a mixture of Ph3PO and a new product readily separated from it by chromatography. These were identified as the pyrrolo[2,1-a]isoindol-5-one-2-thiones 19 and 20 formed in moderate yield, which appear to be a previously unknown compound type.
This is consistent with the behaviour of the ester-containing oxo ylides 7 as shown in Scheme 2,8 and also the simpler oxo ylides such as 15 and 16 which were generated by Bestmann, and found upon heating in solution to cyclise to give pyrroloisoindolediones 9 directly.14 It therefore appears that the presence of the Ph3P=CH–C=S function, which in compounds 3 with R2 = Me or But led to the rearrangement to 4, is not sufficient to overcome the tendency of phthalimido-containing ylides to undergo intramolecular Wittig reaction. Attempts to overcome this problem by using thioxo ylides with different N-protecting groups derived from the α-tert-butoxycarbonyl analogues of 5 have so far not succeeded. We therefore turned our attention to the second approach.
Formation of a thioxo-stabilised ylide from a tertiary phosphine with three different groups, followed by selective migration of one of these from P to S would give a product with a stereogenic centre at phosphorus. For this purpose we selected the arylmethylphenylphosphines 21–23 since these have markedly different groups, leading to a good prospect of selectivity in the migration, and are also accessible in enantiomerically pure form thanks to the asymmetric Appel reaction developed by Gilheaney and co-workers,15,16 thus potentially opening the way to non-racemic 1-sulfanyl-2-phosphanylalkenes.
Reaction of phosphines 21–2315 with bromopinacolone 24 followed by treatment of the resulting phosphonium salts with sodium hydroxide gave the ylides 25–27 as stable crystalline solids in low to moderate yield (Scheme 5). For conversion of these more hydrolytically sensitive ylides into their thioxo analogues we found it essential to conduct the reaction of the O-sulfonylphosphonium triflate intermediates with anhydrous sodium sulfide in dry DMF giving the desired products 28–30 in acceptable yield as yellow oils.
When these ylides were subjected to FVP at 10–2 Torr and 550 or 600 °C, the products collected in the cold trap were rather complex mixtures, which did however show some indication of the desired process having occurred (Scheme 6). Thus for 28 a component with a 31P NMR signal at –55.5 was present consistent with product 31 or 32, while for 30 two separate products with 31P NMR signals at –39.9 and –49.3 in a 2:1 ratio also seemed likely to be the rearrangement products. However in each case these were accompanied by the phosphine sulfide 33 and various other products, meaning that the reactions were unlikely to be of much preparative value. When the residue in the inlet tube was examined however a more interesting result was obtained. The NMR data for the residue in the inlet after FVP of 28 at 550 °C was consistent with the 1,4-benzoxaphosphininium salt structure 34 where the nature of the counterion is unclear. A very similar product was obtained as the involatile residue left when the 2-chlorophenyl oxo-stabilised ylide 26 was subjected to FVP.
The 31P NMR shift around –10 ppm is in reasonable agreement with values previously determined for similar 1,4-oxaphosphininium salts,17,18 and both the chemical shift (6.2–6.3 ppm) and coupling constant (J = 8 Hz) for H-3 are in excellent agreement with literature values.18 Perhaps the most convincing confirmation of the structure comes from the 13C NMR spectra where signals for the four different carbons directly attached to phosphorus are especially prominent [123.7 (d, J = 93 Hz, Ph C-1), 100.9 (d, J = 89 Hz, C-4a), 74.9 (d, J = 89 Hz, CH, C-3), 12.9 (d, J = 61 Hz, PMe)].
In terms of the mechanism for these processes, we postulate initial loss of a methyl radical from 28, a reaction commonly observed under FVP conditions for a wide range of 2-methoxyphenylphosphonium ylides,19 followed by 6-exo-trig cyclisation onto the thiocarbonyl function (Scheme 7). The form in which sulfur is lost and the nature of the resulting counterion is unclear.
In the case of 26, the phosphonium enolate resonance form which is a major contributor to the ylide structure simply undergoes an intramolecular SNAr reaction to afford the benzoxaphosphininium chloride.
EXPERIMENTAL
Melting points were recorded on a Reichert hot-stage microscope and are uncorrected. IR spectra were determined on a Perkin-Elmer 1710 spectrometer as Nujol mulls or thin films. All NMR spectra were obtained using a Varian Gemini 2000 spectrometer, at 300 MHz for 1H, 121 MHz for 31P, and 75 MHz for 13C, and are reported in ppm to high frequency of TMS as internal standard for H and C and relative to external 85% H3PO4 for P. Spectra were recorded in CDCl3 unless otherwise stated. Mass spectra were recorded using an AEI Kratos MS-50 spectrometer using EI (70 eV) or, where noted, CI (isobutane). Elemental analysis was performed using a Carlo-Erba 1106 analyser.
For flash vacuum pyrolysis (FVP), the sample was volatilised from a tube in a Büchi Kugelrohr oven through a 30 × 2.5 cm horizontal fused quartz tube. This was heated externally by a Carbolite Eurotherm tube furnace MTF-12/38A, the temperature being monitored by a Pt/Pt-13%Rh thermocouple situated at the centre of the furnace. The products were collected in a U-shaped trap cooled in liquid nitrogen. The whole system was maintained at a pressure of 10–2 Torr by an Edwards Model E2M5 high capacity rotary oil pump, the pressure being measured by a Pirani gauge situated between the cold trap and the pump. Under these conditions the contact time in the hot zone was estimated to be 10 ms.
Starting Materials
tert-Butyl triphenylphosphoranylideneacetate 1011 was prepared by reacting tert-butyl chloroacetate with triphenylphosphine in toluene to give the phosphonium salt (80%) and then treating this with sodium hydroxide (89%). (2S)-2-Phthalimidopropionyl chloride 118 and (2S)-3-phenyl-2-phthalimidopropionyl chloride 128 were prepared, respectively, from (S)-alanine and (S)-phenylalanine by first melting a mixture of the amino acids with phthalic anhydride, heating for 4 h and recrystallising the product from water, then treatment with thionyl chloride followed by evaporation. The unsymmetrical tertiary phosphines (2-methoxyphenyl)methylphenylphosphine 21, (2-chlorophenyl)methylphenylphosphine 22 and (2-methylphenyl)methylphenylphosphine 23 were prepared by the multi-step method of Gilheaney and co-workers15 as shown in Scheme 5.
Preparation of tert-butyl (4S)-3-oxo-4-phthalimido-2-triphenylphosphoranylidenepentanoate 13
A solution of tert-butyl triphenylphosphoranylideneacetate 10 (5.57 g, 16 mmol) in dry THF (50 mL) was stirred while a solution of (2S)-2-phthalimidopropionyl chloride 11 (2.25 g, 8 mmol) in dry THF (25 mL) was added and the mixture was heated under reflux for 4 h. The mixture was cooled and filtered. Evaporation of the filtrate followed by column chromatography of the residue (SiO2, EtOAc) and recrystallisation from petroleum/EtOAc gave the product 13 (0.90 g, 20%) as colourless crystals; mp 208–210 ˚C; [αD] +3.75 (c 1.0, CH2Cl2]; IR 1709, 1668, 1592, 1387, 1307, 1163, 1078, 925, 722 cm–1; 1H NMR δ 7.7–7.5 (m, 10H), 7.5–7.3 (m, 9H), 6.02 (q, J = 7 Hz, 1H), 1.95 (d, J = 7 Hz, 3H), 1.05 (s, 9H); 13C NMR δ 192.2 (d, J = 4 Hz, CO), 168.7 (N-CO), 166.7 (d, J = 14 Hz, COO), 133.2 (2CH), 133.0 (d, J = 10 Hz, PPh C-2), 132.5 (2C), 131.5 (d, J = 3 Hz, PPh C-4), 128.5 (d, J = 13 Hz, PPh C-3), 126.6 (d, J = 93 Hz, PPh C-1), 122.8 (2CH), 78.8 (CMe3), 54.2 (d, J = 9 Hz, CHMe), 28.1 (CMe3), 16.0 (CHMe) [ylide C not apparent]; 31P NMR δ +18.9; MS (CI) m/z 578 (M+H+, 5), 364 (19), 319 (8), 301 (5), 279 (33), 263 (100). Anal. Calcd for C35H32NO5P: C, 72.78; H, 5.58; N, 2.42. Found: 73.10; H, 5.72; N, 2.66.
Preparation of tert-butyl (4S)-3-oxo-5-phenyl-4-phthalimido-2-triphenylphosphoranylidenepentanoate 14
The same method as for 13 using tert-butyl triphenylphosphoranylideneacetate 10 and (2S)-3-phenyl-2-phthalimidopropionyl chloride 12 (2.47 g, 8 mmol) gave the product 14 (1.81 g, 39%) as colourless crystals; mp 200–202 ˚C; [αD] –1.30 (c 1.0, CH2Cl2]; IR 1711, 1646, 1624, 1571, 1440, 1326, 1176, 1108, 1090, 729, 693 cm–1; 1H NMR δ 7.8–7.6 (m, 8H), 7.5–7.3 (m, 12H), 7.2–7.0 (m, 4H), 6.35 (dd, J = 12, 4 Hz, 1H), 3.90 (half AB pattern of d, J = 14, 12 Hz, 1H), 3.85 (half AB pattern of d, J = 14, 4 Hz, 1H), 1.05 (s, 9H); 13C NMR δ 190.0 (d, J = 4 Hz, CO), 168.8 (N-CO), 166.8 (d, J = 14 Hz, COO), 139.4 (C), 133.1 (2CH), 133.1 (d, J = 10 Hz, PPh C-2), 132.2 (2C), 131.6 (d, J = 3 Hz, PPh C-4), 129.1 (2CH), 128.5 (d, J = 13 Hz, PPh C-3), 128.1 (2CH), 126.6 (d, J = 93 Hz, PPh C-1), 126.0 (CH), 122.8 (2CH), 78.8 (CMe3), 60.5 (d, J = 9 Hz, CHMe), 34.8 (CH2Ph) 28.1 (CMe3) [ylide C not apparent]; 31P NMR δ +18.9; MS (CI) m/z 653 (M+, 2), 386 (10), 364 (29), 338 (27), 320 (21), 294 (25), 279 (80), 263 (64), 196 (6), 89 (100). Anal. Calcd for C41H36NO5P: C, 75.33; H, 5.55; N, 2.14. Found: 75.64; H, 5.29; N, 2.10.
Preparation of (3S)-2-oxo-3-phthalimidobutylidenetriphenylphosphorane 15
A solution of ylide 13 (0.72 g, 1.23 mmol) and p-toluenesulfonic acid (0.36 g, 1.85 mmol) in toluene (50 mL) was heated under reflux for 3 h. The solution was cooled and added to a mixture of aqueous NaOH (2 M) and EtOAc. Separation and drying of the organic layer followed by evaporation gave the product 15 (0.34 g, 56%) as a colourless solid; mp 214–216 ˚C; [αD] –1.60 (c 1.0, CH2Cl2]; IR 1771, 1706, 1603, 1378, 1225, 1121, 881, 723, 680 cm–1; 1H NMR δ 7.80 (m, 2H) 7.7–7.6 (m, 6H), 7.6–7.4 (m, 9H), 7.3–7.2 (m, 2H), 5.00 (q, J = 6 Hz, 1H), 3.75 (br s, 1H, P=CH), 1.80 (d, J = 6 Hz, 3H); 13C NMR δ 187.6 (CO), 168.3 (N-CO), 133.4 (2CH), 133.1 (d, J = 10 Hz, PPh C-2), 132.5 (2C), 131.9 (PPh C-4), 128.7 (d, J = 13 Hz, PPh C-3), 126.8 (d, J = 91 Hz, PPh C-1), 122.9 (2CH), 53.0 (d, J = 15 Hz, CH), 48.9 (d, J 112 Hz, P=C), 16.2 (Me); 31P NMR δ +17.6; MS (CI) m/z 477 (M+, 5), 317 (6), 279 (100), 263 (25), 200 (46). Anal. Calcd for C30H24NO3P: C, 75.46; H, 5.07; N, 2.93. Found: 75.73; H, 5.14; N, 2.55.
Preparation of (3S)-2-oxo-4-phenyl-3-phthalimidobutylidenetriphenylphosphorane 16
The same method as for 15 using ylide 14 (0.26 g, 0.409 mmol) and p-toluenesulfonic acid (0.12 g, 0.614 mmol) gave the product 16 (0.20 g, 83%) as a colourless solid; mp 183–185 ˚C; [αD] +4.2 (c 1.0, CH2Cl2]; IR 1770, 1711, 1378, 1107, 718 cm–1; 1H NMR δ 7.75–7.40 (m, 16H), 7.30–7.05 (m, 8H), 5.20 (t, J = 8 Hz, 1H), 3.80 (br s, 1H, P=CH), 3.70 (d, J = 8 Hz, 2H); 13C NMR δ 186.5 (CO), 168.4 (N-CO), 139.2 (C), 133.4 (2CH), 133.1 (d, J = 10 Hz, PPh C-2), 132.0 (PPh C-4), 131.6 (2CH), 128.9 (d, J = 13 Hz, PPh C-3), 128.5 (CH), 128.4 (2CH), 126.9 (C), 126.7 (d, J = 85 Hz, PPh C-1), 123.0 (2CH), 58.9 (d, J = 15 Hz, CH), 49.6 (d, J 111 Hz, P=C), 35.1 (CH2); 31P NMR δ +17.6; MS (CI) m/z 554 (M+H+, 1), 294 (15), 279 (100), 263 (29), 203 (11). Anal. Calcd for C36H28NO3P: C, 78.10; H, 5.10; N, 2.53. Found: 77.64; H, 4.78; N, 2.35.
Preparation of (3S)-2-thioxo-3-phthalimidobutylidenetriphenylphosphorane 17
A solution of ylide 15 (2.0 g, 4.2 mmol) in dry CH2Cl2 (100 mL) was stirred while trifluoromethanesulfonic anhydride (1.18 g, 4.2 mmol) was added dropwise. The mixture was stirred under N2 for 15 h and the evaporated. The residue was dissolved in EtOH (50 mL) and a solution of sodium sulfide nonahydrate (1.51 g, 6.3 mmol) in water (50 mL) was added. After stirring for 12 h, the mixture was extracted with CH2Cl2 (3 × 30 mL). Drying and evaporation of the extract gave the product 17 as a yellow powder (1.22 g, 59%); mp 204–205 ˚C; [αD] –0.9 (c 1.0, CH2Cl2]; IR 1703, 1103, 720 cm–1; 1H NMR δ 7.9–7.3 (m, 19H), 5.32 (d, J = 40 Hz, 1H, P=CH), 5.28 (q, J = 7 Hz, 1H), 1.92 (d, J = 7 Hz, 3H); 13C NMR δ 200.0 (CS), 168.3 (N-CO), 133.4 (2CH), 133.3 (d, J = 9 Hz, PPh C-2), 133.0 (2C), 132.2 (PPh C-4), 128.8 (d, J = 13 Hz, PPh C-3), 124.4 (d, J = 93 Hz, PPh C-1), 123.0 (2CH), 82.3 (d, J 118 Hz, P=C), 57.8 (d, J = 17 Hz, CH), 18.5 (Me); 31P NMR δ +8.6; MS (CI) m/z [M+ not apparent], 291 (6), 279 (100), 263 (15), 220 (7). Anal. Calcd for C30H24NO2PS: C, 73.00; H, 4.90; N, 2.84. Found: 71.23; H, 4.80; N, 2.67.
Preparation of (3S)-2-thioxo-4-phenyl-3-phthalimidobutylidenetriphenylphosphorane 18
The same method as for 17 using ylide 16 (1.3 g, 2.4 mmol), trifluoromethanesulfonic anhydride (0.67 g, 2.4 mmol) and sodium sulfide nonahydrate (0.86 g, 3.6 mmol) gave the product 18 as a yellow powder (0.69 g, 51%); mp 217–218 ˚C; [αD] –2.3 (c 1.0, CH2Cl2]; IR 1707, 1323, 1097, 722 cm–1; 1H NMR δ 7.7–7.4 (m, 20H), 7.3–7.0 (m, 4H), 5.50 (m, 1H), 5.30 (d, J = 40 Hz, 1H, P=CH), 3.98 (dd, J = 14, 5 Hz, 1H, CH2), 3.75 (dd, J = 14, 8 Hz, 1H, CH2); 13C NMR δ 198.2 (CS), 168.3 (N-CO), 139.1 (C), 133.4 (2CH), 133.3 (d, J = 10 Hz, PPh C-2), 133.0 (2C), 132.3 (PPh C-4), 132.0 (2CH), 128.9 (d, J = 13 Hz, PPh C-3), 128.2 (2CH), 126.1 (CH), 124.4 (d, J = 93 Hz, PPh C-1), 123.0 (2CH), 83.0 (d, J 116 Hz, P=C), 64.0 (d, J = 17 Hz, CH), 37.6 (CH2); 31P NMR δ +8.6. Anal. Calcd for C36H28NO2PS: C, 75.90; H, 4.95; N, 2.46. Found: 76.22; H, 4.85; N, 2.63.
Preparation of (3S)-3-methyl-2-thioxo-2,3-dihydro-5H-pyrrolo[2,1-a]isoindol-5-one 19
The ylide 17 (0.45 g) was subjected to FVP at 650 ˚C and 10–2 Torr. The material in the cold trap was dissolved out with CH2Cl2 and shown by 31P and 1H NMR to consist of Ph3PO and a new compound. These were separated by column chromatography (SiO2, EtOAc) to give the product 19 (0.11 g, 55%) as light brown crystals; mp 114–116 ˚C; IR 1704, 1618, 1314, 1168, 892, 767, 696 cm–1; 1H NMR δ 7.85–7.80 (m, 1H), 7.75–7.70 (m, 1H), 7.70–7.60 (m, 2H), 5.95 (s, 1H), 4.27 (q, J = 7 Hz, 1H), 1.59 (d, J = 7 Hz, 3H); 13C NMR δ 202.9 (CS), 165.1 (CO), 162.1 (C), 133.3 (CH), 133.0 (C), 132.6 (CH), 130.4 (C), 124.3 (CH), 124.2 (CH), 102.0 (CH), 57.3 (CH), 15.6 (Me); MS (CI) m/z 200 (M+–Me, 100), 148 (10), 75 (6). HRMS Calcd for C11H6NOS (M+–Me): 200.0170. Found: 200.0176.
Preparation of (3S)-3-benzyl-2-thioxo-2,3-dihydro-5H-pyrrolo[2,1-a]isoindol-5-one 20
The ylide 18 (0.57 g) was subjected to FVP at 650 ˚C and 10–2 Torr as described above to give, after chromatographic separation, the product 20 (0.15 g, 52%) as light brown crystals; mp 84–85 ˚C; IR 1718, 1620, 1076, 721 cm–1; 1H NMR δ 7.90–7.85 (m, 1H), 7.75–7.65 (m, 1H), 7.65–7.60 (m, 2H), 7.15–7.05 (m, 5H), 5.80 (s, 1H), 4.60 (dd, J = 5, 3 Hz, 1H), 3.73 (half AB pattern of d, J = 14, 5 Hz, CH2), 3.56 (half AB pattern of d, J = 14, 3 Hz, CH2); 13C NMR δ 201.8 (CS), 167.3 (CO), 162.5 (C), 134.3 (C), 133.2 (CH), 132.5 (CH), 131.6 (C), 130.1 (C), 129.7 (2CH), 128.1 (2CH), 126.8 (CH), 124.3 (CH), 124.2 (CH), 103.2 (CH), 62.1 (CH), 35.1 (CH2); MS (CI) m/z 200 (M+–Me, 100), 148 (10), 75 (6). Anal. Calcd for C18H13NOS: C, 74.20; H, 4.50; N, 4.81. Found: 73.92; H, 4.83; N, 4.94.
Preparation of 1-((2-methoxyphenyl)methyl(phenyl)phosphoranylidene)-3,3-dimethylbutan-2-one 25
A solution of (2-methoxyphenyl)methylphenylphosphine 21 (0.94 g, 4 mmol) in dry toluene (25 mL) was stirred at rt while 1-bromo-3,3-dimethylbutan-2-one 24 (0.72 g, 4 mmol) was added dropwise. After the addition, the mixture was stirred for 12 h and the resulting oily solid was filtered off and washed with toluene. It was then dissolved in hot water (15 mL) and the resulting solution treated with NaOH (0.33 g, 8 mmol) dissolved in water (10 mL). The milky suspension was the extracted with CH2Cl2 (5 × 30 mL) and the combined extracts were dried and evaporated to give the product 25 (1.03 g, 77%) as a pale brown solid; mp 120–122 ˚C; IR 1590, 1522, 1275, 1107, 924, 752, 697 cm–1; 1H NMR δ 7.74 (ddd, J = 15, 7.5, 1.5 Hz, 1H), 7.62–7.40 (m, 6H), 7.13 (tdd, J = 7.5, 2, 1 Hz, 1H), 6.93 (dd, J = 7.5, 5 Hz, 1H), 3.68 (s, 3H, OMe), 3.43 (br s, 1H, P=CH), 2.44 (d, J = 15 Hz, 3H, PMe), 1.23 (s, 9H); 13C NMR δ 200.3 (CO), 160.9 (d, J = 2 Hz, C-OMe), 135.4 (d, J = 9 Hz, Ar CH), 133.9 (d, J = 2 Hz, Ar CH), 131.0 (d, J = 3 Hz, Ph C-4), 130.8 (d, J = 10 Hz, 2CH, Ph C-2), 129.4 (d, J = 89 Hz, C, Ph C-1), 128.4 (d, J = 12 Hz, 2CH, Ph C-3), 121.5 (d, J = 12 Hz, Ar CH), 115.2 (d, J = 86 Hz, C, Ar C-1), 111.5 (d, J = 6 Hz, Ar CH), 55.3 (OMe), 47.7 (d, J = 109 Hz, P=C), 40.5 (d, J = 12 Hz, CMe3), 28.8 (CMe3), 11.3 (d, J = 65 Hz, PMe); 31P NMR δ +9.4; MS (ES) m/z 329 (M++H, 100); HRMS: Calcd for C20H26O2P (M+H): 329.1670. Found: 329.1672 ; Anal. Calcd for C20H25O2P: C, 73.15; H, 7.67. Found: 72.87; H, 7.23.
Preparation of 1-((2-chlorophenyl)methyl(phenyl)phosphoranylidene)-3,3-dimethylbutan-2-one 26
The same method as for 25 using (2-chlorophenyl)methylphenylphosphine 22 (1.33 g, 5.7 mmol) and 1-bromo-3,3-dimethylbutan-2-one 24 (1.02 g, 5.7 mmol) gave the product 26 (0.32 g, 17%) as pale yellow crystals; mp 104–106 ˚C ; 1H NMR δ 7.90–7.84 (m, 1H), 7.56–7.51 (m, 3H), 7.48–7.40 (m, 5H), 3.44 (d, J = 27 Hz, P=CH), 2.52 (d, J = 14 Hz, PMe), 1.19 (s, 9H); 13C NMR δ 200.7 (CO), 136.6 (d, J = 4 Hz, C-Cl), 136.4 (d, J = 11 Hz, Ar CH), 133.3 (d, J = 3 Hz, Ph C-4), 131.6 (d, J = 3 Hz, Ar CH), 131.02 (d, J = 10 Hz, 2CH, Ph C-2), 130.95 (d, J = 5 Hz, Ar CH), 128.8 (d, J = 12 Hz, 2CH, Ph C-3), 127.9 (d, J = 90 Hz, C, Ph C-1), 127.2 (d, J = 12 Hz, Ar CH), 126.0 (d, J = 85 Hz, C, Ar C-1), 48.8 (d, J = 110 Hz, P=CH), 40.5 (d, J = 12 Hz, CMe3), 28.7 (CMe3), 11.2 (d, J = 65 Hz, PMe); 31P NMR δ +12.0; MS (CI) m/z 335 (37Cl-M+H, 20), 333 (35Cl-M+H, 100), 277 (14), 275 (56), 253 (5), 251 (18); HRMS (CI): Calcd for C19H23ClOP (M+H): 333.1175. Found: 333.1180.
Preparation of 1-((2-methylphenyl)methyl(phenyl)phosphoranylidene)-3,3-dimethylbutan-2-one 27
The same method as for 25 using (2-methylphenyl)methylphenylphosphine 23 (1.88 g, 8.9 mmol) and 1-bromo-3,3-dimethylbutan-2-one 24 (1.58 g, 8.9 mmol), with recrystallisation from EtOAc gave the product 27 (0.71 g, 26%) as colourless crystals; mp 116–117 ˚C ; 1H NMR δ 7.61–7.49 (m, 4H), 7.47–7.41 (m, 3H), 7.31–7.27 (m, 1H), 7.25–7.22 (m, 1H), 3.50 (d, J = 27 Hz, 1H, P=CH), 2.40 (d, J = 16 Hz, 3H, PMe), 2.29 (s, 3H, Ar-Me), 1.19 (s, 9H); 13C NMR δ 200.2 (CO), 141.4 (d, J = 8 Hz, Ar CMe), 133.1 (d, J = 11 Hz, Ar CH), 132.1 (d, J = 10 Hz, Ar CH), 132.0 (d, J = 3 Hz, Ph C-4), 131.6 (d, J = 3 Hz, Ar CH), 131.3 (d, J = 11 Hz, 2CH, Ph C-2), 128.9 (d, J = 12 Hz, 2CH, Ph C-3), 126.1 (d, J = 13 Hz, Ar CH), 28.6 (CMe3), 21.7 (d, J = 5 Hz, Ar-Me), 12.4 (d, J = 68 Hz, PMe) [signals for Ph C-1, Ar C-1, P=CH and CMe3 not apparent]; 31P NMR δ +9.3; MS (ES) m/z 313 (M++H, 100); MS (EI) m/z 312 (M+, 3), 255 (M–But, 100); HRMS (EI): Calcd for C20H25OP (M+): 312.1643. Found: 312.1645.
Preparation of 1-((2-methoxyphenyl)methyl(phenyl)phosphoranylidene)-3,3-dimethylbutane-2-thione 28
A solution of ylide 25 (1.0 g, 3 mmol) in dry CH2Cl2 (40 mL) was stirred at rt under nitrogen while trifluoromethanesulfonic anhydride (0.86 g, 0.50 mL, 3 mmol) was added dropwise. After the addition the mixture was stirred for 1 h and then evaporated under reduced pressure and the residue was taken up in dry DMF (30 mL). Anhydrous sodium sulfide (0.35 g, 4.5 mmol) was added and the mixture was stirred at rt for 72 h. The mixture was then added to water (100 mL) and the product was extracted with diethyl ether (3 × 20 mL). The extracts were washed thoroughly with water, dried and evaporated to give the product 28 (0.22 g, 21%) as a brown oil; 1H NMR δ 7.62–7.59 (m, 1H), 7.57–7.42 (m, 6H), 7.07–7.01 (m, 1H), 7.00–6.94 (m, 1H), 4.89 (d, J = 33 Hz, P=CH), 3.70 (s, 3H, OMe), 2.78 (d, J = 15 Hz, 3H, PMe), 1.37 (s, 9H); 13C NMR δ 211.2 (d, J = 3 Hz, CS), 160.9 (d, J = 2 Hz, C-OMe), 135.5 (d, J = 8 Hz, Ar CH), 134.2 (d, J = 2 Hz, Ar CH), 131.4 (d, J = 3 Hz, Ph C-4), 130.8 (d, J = 9 Hz, 2CH, Ph C-2), 128.6 (d, J = 12 Hz, 2CH, Ph C-3), 127.2 (d, J = 89 Hz, C, Ph C-1), 121.0 (d, J = 12 Hz, Ar CH), 112.0 (d, J = 90 Hz, C, Ar C-1), 111.5 (d, J = 6 Hz, Ar CH), 83.8 (d, J = 115 Hz, P=C), 55.3 (OMe), 43.1 (d, J = 14 Hz, CMe3), 28.0 (CMe3), 10.7 (d, J = 67 Hz, PMe); 31P NMR δ +1.2.
Preparation of 1-((2-chlorophenyl)methyl(phenyl)phosphoranylidene)-3,3-dimethylbutane-2-thione 29
The same method as for 28 using ylide 26 (0.15 g, 0.45 mmol), trifluoromethanesulfonic anhydride (0.17 g, 0.62 mmol) and sodium sulfide (0.35 g, 4.5 mmol) gave the product 29 (0.09 g, 60%) as an orange oil;
1H NMR δ 7.90–7.82 (m, 1H), 7.57–7.34 (m, 8H), 4.90 (d, J = 35 Hz, P=CH), 2.51 (d, J = 14 Hz, 3H, PMe), 1.36 (s, 9H); 31P NMR δ +0.9.
Preparation of 1-((2-methylphenyl)methyl(phenyl)phosphoranylidene)-3,3-dimethylbutane-2-thione 30
The same method as for 28 using ylide 27 (0.22 g, 0.78 mmol), trifluoromethanesulfonic anhydride (0.24 g, 0.78 mmol) and sodium sulfide (90 mg, 1.15 mmol) gave the product 30 (0.12 g, 48%) as a yellow oil; 1H NMR δ 7.62–7.38 (m, 7H), 7.31–7.17 (m, 2H), 4.99 (d, J = 34 Hz, P=CH), 2.76 (d, J = 14 Hz, 3H, PMe), 2.35 (s, 3H. Ar-Me), 1.37 (s, 9H); 13C NMR δ 211.7 (d, J = 4 Hz, CS), 140.8 (d, J = 8 Hz, Ar CMe), 133.8 (d, J = 10 Hz, Ar CH), 132.8 (d, J = 11 Hz, Ar CH), 132.0 (d, J = Hz, Ph C-4), 131.8 (d, J = 11 Hz, Ar CH), 131.2 (d, J = 9 Hz, 2CH, Ph C-2), 131.1 (d, J = 10 Hz, Ar CH), 128.7 (d, J = 12 Hz, 2CH, Ph C-3), 126.7 (d, J = 86 Hz, Ph C-1), 125.8 (d, J = 7 Hz, Ar CH), 123.9 (d, J = 88 Hz, Ar C-1), 83.5 (d, J = 114 Hz, P=CH), 43.1 (d, J = 14 Hz, CMe3), 28.5 (CMe3), 21.5 (d, J = 5 Hz, Ar-Me), 12.5 (d, J = 67 Hz, PMe); 31P NMR δ +1.2; HRMS (CI): Calcd for C20H26PS (M+H): 329.1493. Found: 329.1498.
Pyrolysis of ylide 28 leading to formation an involatile residue of 2-tert-butyl-4-methyl-4-phenyl-1,4-benzoxaphosphininium salt 34
A sample of ylide 28 (170 mg) was subjected to FVP at 550 C and 10–2 Torr. Analysis of the residual solid left in the inlet tube showed it to consist mainly of the salt 34 (30 mg); 1H NMR δ 7.98–7.87 (m, 3H), 7.79–7.74 (m, 1H), 7.68–7.56 (m, 3H), 7.51–7.46 (m, 1H), 7.42–7.37 (m, 1H), 6.16 (d, J = 8 Hz, =CH–), 2.87 (d, J = 14 Hz, PMe), 1.38 (s, 9H); 13C NMR δ 178.4 (d, J = 4.5, C-2), 155.0 (d, J = 2 Hz, C-8a), 136.1 (d, J = 2 Hz, CH, Ph C-4), 134.3 (d, J = 3 Hz, CH, C-5), 132.1 (d, J = 12 Hz, 2CH, Ph C-2), 131.6 (d, J = 7 Hz, CH, C-7), 130.0 (d, J = 14 Hz, 2CH, Ph C-3), 127.1 (d, J = 12 Hz, CH, C-6), 123.7 (d, J = 93 Hz, Ph C-1), 119.1 (d, J = 5 Hz, CH, C-8), 100.9 (d, J = 89 Hz, C-4a), 74.9 (d, J = 89 Hz, CH, C-3), 39.1 (d, J = 9 Hz, CMe3), 27.7 (CMe3), 12.9 (d, J = 61 Hz, PMe); 31P NMR δ –10.2; HRMS (ES) Calcd for C19H22OP (M+): 297.1408. Found: 297.1401.
Pyrolysis of ylide 26 leading to formation of an involatile residue of 2-tert-butyl-4-methyl-4-phenyl-1,4-benzoxaphosphininium chloride 34
A sample of ylide 26 (40 mg) was subjected to FVP at 550 C and 10–2 Torr. Analysis of the residual solid left in the inlet tube showed it to consist mainly of the chloride salt 34 (10 mg); 1H NMR δ 8.2–7.4 (m, 9H), 6.34 (d, J = 8 Hz, =CH–), 3.11 (d, J = 15 Hz, PMe), 1.39 (s, 9H); 13C NMR δ 136.1 (d, J = 2 Hz, CH, Ph C-4), 134.3 (d, J = 3 Hz, CH, C-5), 132.2 (d, J = 12 Hz, 2CH, Ph C-2), 131.7 (d, J = 7 Hz, CH, C-7), 130.0 (d, J = 14 Hz, 2CH, Ph C-3), 127.2 (d, J = 11 Hz, CH, C-6), 123.7 (d, J = 93 Hz, Ph C-1), 119.1 (d, J = 5 Hz, CH, C-8), 100.9 (d, J = 89 Hz, C-4a), 74.9 (d, J = 89 Hz, CH, C-3), 39.1 (CMe3), 27.7 (CMe3), 13.0 (d, J = 61 Hz, PMe) [C-2 and C-8a not apparent]; 31P NMR δ –10.4; HRMS (ES) Calcd for C19H22OP (M+): 297.1408. Found: 297.1413.
References
1. R. A. Aitken and A. W. Thomas, in Chemistry of the Functional Groups, Supplement A3, ed. by S. Patai, Wiley, New York, 1997, 473; F. Eymery, B. Iorga, and P. Savignac, Synthesis, 2000, 185. CrossRef
2. R. A. Aitken, N. Karodia, T. Massil, and R. J. Young, J. Chem. Soc., Perkin Trans. 1, 2002, 533. CrossRef
3. R. A. Aitken and L. Murray, J. Org. Chem., 2008, 73, 9781. CrossRef
4. R. A. Aitken and A. N. Garnett, New J. Chem., 2009, 33, 2402. CrossRef
5. R. A. Aitken, G. Dawson, N. S. Keddie, H. Kraus, A. M. Z. Slawin, J. Wheatley, and J. D. Woollins, Chem. Commun., 2009, 7381. CrossRef
6. R. A. Aitken and N. Karodia, Chem. Commun., 1996, 2079. CrossRef
7. R. A. Aitken, G. M. Buchanan, N. Karodia, T. Massil, and R. J. Young, Tetrahedron Lett., 2001, 42, 141. CrossRef
8. R. A. Aitken, H. R. Cooper, and A. P. Mehrotra, J. Chem. Soc., Perkin Trans. 1, 1996, 475. CrossRef
9. R. A. Aitken, M. E. Balkovich, H. J. Bestmann, O. Clem, S. E. Gibson, and T. Röder, Synlett, 1999, 1235. CrossRef
10. R. A. Aitken, N. A. Al-Awadi, M. E. Balkovich, H. J. Bestmann, O. Clem, S. Gibson, A. Groß, A. Kumar, and Th. Röder, Eur. J. Org. Chem., 2003, 840. CrossRef
11. M. P. Cooke, Jr. and D. L. Burman, J. Org. Chem., 1982, 47, 4955. CrossRef
12. L. Capuano, S. Drescher, and V. Huch, Liebigs Ann. Chem., 1993, 125. CrossRef
13. H. J. Bestmann, A. Pohlschmitt, and K. Kumar, Tetrahedron Lett., 1992, 5955. CrossRef
14. H. J. Bestmann, T. Moenius, and F. Soliman, Chem. Lett., 1986, 1527. CrossRef
15. E. Bergin, C. T. O'Connor, S. B. Robinson, E. M. McGarrigle, C. P. O'Mahony, and D. G. Gilheany, J. Am. Chem. Soc., 2007, 129, 9566. CrossRef
16. K. V. Rajendran, L. Kennedy, and D. G. Gilheany, Eur. J. Org. Chem., 2010, 5642. CrossRef
17. D. W. Allen and J. C. Tebby, J. Chem. Soc. (B), 1970, 1527. CrossRef
18. M.-L. Filleux-Blanchard, M. Simalty, M. Berry, H. Chahine, and M. H. Mebazaa, Bull. Soc. Chim. Fr., 1970, 3549.
19. R. A. Aitken and G. Burns, J. Chem. Soc., Perkin Trans. 1, 1994, 2455; CrossRef R. A. Aitken, ARKIVOC, 2000, v, 798; CrossRef R. A. Aitken, C. K. Bradbury, G. Burns, and J. J. Morrison, Synlett, 1995, 53; CrossRef R. A. Aitken, G. Burns, and J. J. Morrison, J. Chem. Soc., Perkin Trans. 1, 1998, 3937. CrossRef