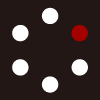
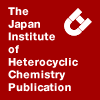
HETEROCYCLES
An International Journal for Reviews and Communications in Heterocyclic ChemistryWeb Edition ISSN: 1881-0942
Published online by The Japan Institute of Heterocyclic Chemistry
e-Journal
Full Text HTML
Received, 11th July, 2013, Accepted, 1st August, 2013, Published online, 7th August, 2013.
DOI: 10.3987/COM-13-S(S)90
■ Regioselective Bromination: An Approach to the D-Ring of the Gilvocarcins
Ehesan U. Sharif and George A. O'Doherty*
Department of Chemistry and Chemical Biology, Northeastern University, 360 Huntington Avenue, Boston, MA 02115-5096, U.S.A.
Abstract
A method for the regioselective ortho-bromination of unsymmetrically protected 3,5-dihydroxybenzoic acid esters has been developed. The route involves protecting group optimization studies to attain high regioselectivity for the ortho-bromination. Pd-catalyzed stannation and boration were performed to construct the D-ring coupling partners for the synthesis of gilvocarcin analogs.INTRODUCTION
The angucycline family of natural products has long been known for their impressive biological activities and unique structures.2 The gilvocarcins, a subset of angucycline family of natural products gained significance attention from synthetic and biological community because of its remarkably low in vivo cytotoxicity.2b,3 As a result, there have been numerous synthetic approaches to the gilvocarcins and defucogilvocarcins.4 The most potent member of this class of natural compounds is the gilvocarcin V, which also exhibits potent antiviral and antitumor activity. One of the mechanisms by which it exhibits antitumor activity is by inhibiting topoisomerase II.5 It has been reported that the vinyl side chain of gilvocarcin V undergoes a [2+2]-cycloadduct formation with DNA thymidine. Gilvocarcin V can also cause cross-linking between DNA and histone H3.6 In an effort to expand upon our synthetic endeavors toward the jadomycins,7 we embarked upon the synthesis and biological evaluation of novel gilvocarcin analogs with the aim of finding analogs with improved DNA binding and/or topoisomerase inhibition. In particular, we were interested in modifying the alkyl side chain at C-8 position, by building upon Snieckus’s late stage coupling strategy.4d
Retrosynthetically, we envisioned an alternative route to the gilvocarcins (Scheme 1) that combines aspects of the Snieckus’s and Echavarren’s syntheses.4d,4i The route will require the functionalization of triflate 2, which could be prepared from biaryl quinone 3. The quinone 3 could be obtained by coupling of bromojuglone 4 and metallated 5. Importantly, this revised route replaces an ester (3, R1 = OMe) for an amide (3, R1 = NMe2) used in the Echavarren’s reductive lactonization for the construction of the tetracyclic ring system. This change required an alternative approach for the synthesis of the desired metalated coupling partner 5, as the Snieckus’s lithiation strategy fails for the ester substrate 6a and the ortho-lithiation of amide (6b to 5) would be unselective. Thus we sought to generate 5 from bromide 6, which in turn could result from a regioselective bromination and orthogonal protection of 3,5-dihydroxybenzoic acid 7. Herein we report our successful synthesis of metallated 5, which required the development of a regioselective bromination of 3,5-dihydroxybenzoic acid derivatives. This effort involved the search for suitable protecting groups and conditions for this synthesis.
RESULTS AND DISCUSSION
The synthesis of 5 with either boron or tin metallation began with the preparation of the required bromination substrates 8-12 (with symmetrical or unsymmetrical substitutions) from readily available dihydroxybenzocic acid 7 (Scheme 2). The 3,5-dihydroxybenzoic acid 7 was converted to the corresponding methyl ester 8 upon treatment with catalytic p-TsOH in MeOH.8 The methyl 3,5-dihydroxybenzoate 8 was then mono-benzylated (K2CO3/BnBr) to form 11 or silylated (TBSCl/Imid.) to give 12.9,10 Dimethylation of 8 using K2CO3 and MeI produced 9,11 which was then treated with LiAlH4 to obtain the corresponding benzyl ether 10.12
After synthesizing the symmetrical substrates (8-10) and the unsymmetrical substrates (11 and 12), we investigated their selective bromination. We first looked at the selective mono-bromination of the symmetrical substrates 8-10 (Scheme 3), which was undertaken with 1 eq. brominating reagent in CCl4. In the case of methyl 3,5-dihydroxybenzoate 8, we found that only bis-brominated product 1413 was observed along with recovered starting material. In contrast, if the phenols were protected as methyl ethers (9 and 10), only mono-bromination occurred to give 15 and 16, respectively.14,15 Although mono-bromination could be achieved selectively in case of symmetrical substrates (9 and 10), the regioselective deprotection of the methyl ethers (15 and 16) proved to be problematic. For instance, we were unable to find conditions for which only one methyl group was removed using reagents like BBr3 and AlCl3.
Fortunately turning to the unsymmetrical substrates allowed for the identification of a practical alternative. The solution derived from the high reactivity and ortho-directing ability of the phenol. Thus, bromination of 11 yielded only the desired ortho-bromide 19 in 75% yield. This result was particularly appealing as under these conditions neither the bis-brominated product 21 nor the regioisomeric ortho-bromide 20 were observed. In an effort to maximize the utility of this approach, we investigated the bromination of silyl ether 12. Unfortunately, the silyl protecting group proved not to be compatible with the bromination conditions. While it appeared that regioselective bromination occurred (12 to 22), it was accompanied with loss of the silyl group (12 to 8) along with subsequent bis-bromination (8 to 14), (Scheme 4).
Having found a suitable protecting group and substitution pattern for the regioselective bromination, we turned to the reaction optimization (11 to 19). First the reaction was performed using different brominating reagents in CCl4 in order to find the optimum reagent. NBS was found to perform better than Br2, while additives like HOAc did not improve the yield. Although acceptable yields of the desired brominated product were obtained, longer reaction times (~8 h) were required for reaction completion. Next we investigated the effect of solvents on the bromination reaction. Of all the solvents screened, CHCl3 was the most effective. Thus under the optimum condition (1.05 eq. NBS in CHCl3), the desired ortho-brominated product 19 was obtained in 97% yield and in ~7 min (Scheme 5).
After developing a successful procedure for regioselective bromination, the phenol 19 was substituted as methyl ether to form 23. The aromatic bromide 23 was then converted to borate 24 or stannane 25 through Pd-catalyzed metallation (Scheme 6).16 The low yield of the stannation reaction led us to optimize the reaction conditions. The best yield (65%) of the stannation reaction was observed by using Pd2(dba)3•CHCl3/PCy3, in the presence of LiCl in dioxane. The moderate yield in stannation was attributed to the in situ proto-destannylation of product 25.
With the required coupling partners in hand, we next pursued their use in Pd-catalyzed cross-coupling reactions to produce substituted juglone 27 (Scheme 7). Both the Stille (25 + 26 to 27) and the Suzuki (24 + 26 to 27) type cross coupling reactions gave coupling product 27, where the Stille reaction giving significantly higher yields (78% vs 30%). The moderate yield in Suzuki cross-coupling is probably due to less tendency of the arylboronic acid pinacol ester 24 to undergo hydrolysis to form the reactive boric acid. The more than satisfactory yields from the Stille reactions discouraged us from pursuing any further optimization of the Suzuki reaction conditions.
In conclusion a highly efficient and regioselective bromination of 3,5-dihydroxy benzoic acid derivatives has been developed. The strategy was successfully employed in the synthesis of the D-ring coupling partners 24 and 25. A Suzuki and Stille cross coupling of the metallated compound 24 and 25 with 2-bromojuglone 26 is also demonstrated to obtain 27, which is envisioned as being useful for the synthesis of gilvocarcin. The orthogonal protecting groups on the D-ring would allow ready installation of the desired functionality at C-8 position for rapid synthesis of analogs. Future works along these lines are ongoing and will be reported in due course.
EXPERIMENTAL
General Methods and Materials: 1H and 13C NMR spectra were recorded on 400 MHz, and 500 MHz spectrometers. Chemical shifts were reported relative to internal tetramethylsilane (δ 0.00) or CDCl3 (δ 7.26) for 1H NMR and CDCl3 (δ 77.0) for 13C NMR. Infrared (IR) spectra were obtained on FT-IR spectrometer. Optical rotations were measured with a digital polarimeter in the solvent specified. Flash column chromatography was performed on 60-200 mesh silica gel. Analytical thin-layer chromatography was performed with precoated glass-backed plates (60 Å, F254) and visualized by quenching of fluorescence and by charring after treatment with p-anisaldehyde or phosphomolybdic acid or potassium permanganate stain. Rf values are obtained by elution in the stated solvent ratios (v/v). Ether (Et2O), THF, methylene chloride (CH2Cl2) and triethylamine (Et3N) were dried by passing through activated alumina columns with argon gas pressure. Commercial reagents were used without purification unless otherwise noted. Air- and/or moisture-sensitive reactions were carried out under an atmosphere of argon/nitrogen using oven-dried glassware and standard syringe/septa techniques.
Methyl 5-(benzyloxy)-2-bromo-3-hydroxybenzoate 19
Phenol 11 (1.0 g, 3.87 mmol) was dissolved in 40 mL dry CHCl3 and cooled to 0 ºC. To this cold solution was added N-bromosuccinimide (723.5 mg, 4.07 mmol) in one portion and the solution was stirred for 7 min. After exactly 7 min, saturated aqueous NaHCO3 (50 mL) was added and the aqueous layer was extracted with CH2Cl2. Combined organic layer was washed with saturated brine, and dried over Na2SO4. After removal of the solvent, the residue was subjected to silica gel column chromatography, elution with 6-8% hexane/EtOAc afforded product 19 (1.26 g, 97%). Colorless solid; mp 72 ºC; Rf (10% hexanes/EtOAc) = 0.65; IR (thin film, cm-1) ν 3368, 3310, 2842, 1724, 1680, 1412, 1264, 1033, 866; 1H NMR (CDCl3, 400 MHz): δ 7.33-7.42 (m, 5H)δ 7.10 (d, J = 2.8 Hz, 1H), δ 6.82 (d, J = 2.8 Hz, 1H), δ 6.04 (brs, 1H), δ 5.05 (s, 2H), δ 3.92 (s, 3H); 13C NMR (CDCl3, 100 MHz): δ 166.3, 158.9, 154.1, 136.2, 132.4, 128.9,128.5, 127.7, 111.0, 105.8, 101.3, 70.6, 52.8; HRMS (ESI): calcd for [C15H13BrO4 + H]+ 337.0075, found 337.0075.
Methyl 2-bromo-5-((tert-butyldimethylsilyl)oxy)-3-hydroxybenzoate 22
Phenol 12 (110.3 mg, 0.39 mmol) was dissolved in 3.9 mL dry CCl4 and cooled to 0 ºC. To this cold solution was added N-bromosuccinimide (73 mg, 0.41 mmol) and stirred for 3 h, gradually rising to ambient temperature. Diluted with Et2O (10 mL), and quenched by adding saturated aqueous NaHCO3 (10 mL). The aqueous layer was extracted with Et2O twice and the combined organic layer was washed with saturated brine, and dried over Na2SO4. After removal of the solvent, the residue was subjected to silica gel column chromatography, eluting with 5% hexane/EtOAc afforded product 22 (63.5 mg, 45%); viscous oil; Rf (15% hexanes/EtOAc) = 0.55; IR (thin film, cm-1) ν 3410, 3300, 2842, 1724, 1680, 1412, 1264, 1033, 866; 1H NMR (CDCl3, 400 MHz): δ 6.89 (d, J = 3.2 Hz, 1H), δ 6.68 (d, J = 3.2 Hz, 1H), δ 3.90 (s, 3H), δ 0.96 (s, 9H), δ 0.19 (s, 6H); 13C NMR (CDCl3, 100 MHz): δ 166.4, 156.0, 154.0, 132.5, 115.8, 110.9, 101.7, 52.7, 25.7, 18.3, −4.3; HRMS (ESI): calcd for [C14H21BrO4Si + H]+ 361.0471, found 361.0474.
Methyl 5-(benzyloxy)-2-bromo-3-methoxybenzoate 23
Bromophenol 19 (4.14 g, 12.28 mmol) was dissolved in 25 mL acetone. After forming a homogeneous solution, K2CO3 (3.4 g, 24.56 mmol) and MeI (3.8 mL, 61.4 mmol) was added. The reaction mixture was refluxed under argon for 1 h, then cooled to room temperature and acetone was removed under reduced pressure. The residue was redissolved in 100 mL Et2O and H2O. The aqueous layer was extracted with Et2O thrice. Combined organic layer was washed with saturated brine, and dried over Na2SO4. After removal of the solvent, the residue was subjected to silica gel column chromatography, eluting with 8-10% hexane/EtOAc afforded product 23 (4.1 g, 95%). Colorless solid: mp 60-61 ºC; Rf (10% hexanes/EtOAc) = 0.85; IR (thin film, cm-1) ν 2842, 1736, 1680, 1434, 1254, 1029, 872; 1H NMR (CDCl3, 400 MHz): δ 7.33-7.43 (m, 5H) δ 6.91 (d, J = 2.8 Hz, 1H), δ 6.65 (d, J = 2.8 Hz, 1H), δ 5.05 (s, 2H), δ 3.92 (s, 3H), δ 3.84 (s, 3H); 13C NMR (CDCl3, 100 MHz): δ 167.2, 158.9, 157.3, 136.1, 134.8, 128.8, 128.4, 127.7, 107.2, 103.1, 102.4, 70.6, 56.6, 52.7; HRMS (ESI): calcd for [C16H15BrO4 + H]+ 351.0232, found 351.0225.
Methyl 5-(benzyloxy)-3-methoxy-2-(4,4,5,5-tetramethyl-1,3,2-dioxaborolan-2-yl)benzoate 24
Aromatic bromide 23 (20 mg, 0.06 mmol) was dissolved in 1.0 mL THF and cooled to –78 ºC and vacuum degased and back filled with argon. To this degassed reaction mixture was added bis(pinacolato)diborane (28.95 mg, 0.11 mmol), KOAc (17.67 mg, 0.18 mmol) and (dppf)2PdCl2•CH2Cl2 (2.45 mg, 0.003 mmol). The reaction mixture was refluxed overnight under argon. The reaction mixture was cooled to 0 ºC and was diluted with Et2O and quenched with saturated aqueous NaHCO3. The organic layer was extracted with Et2O (20 mL X 2). The pooled organic layer was subsequently washed with saturated brine and dried over Na2SO4. After removal of the solvent under reduced pressure, the residue was subjected to flash column chromatography using silica gel. Elution with 8-10% hexane-EtOAc gave borate 24 (17 mg, 75%) as a colorless solid: Rf (10% hexanes/EtOAc) = 0.30; mp 134 ºC; IR (thin film, cm-1) ν 2910, 1741, 1680, 1429, 1320, 1154, 864; 1H NMR (CDCl3, 400 MHz): δ 7.31-7.44 (m, 5H) δ 7.19 (d, J = 2.0 Hz, 1H), δ 6.64 (d, J = 1.2 Hz, 1H), δ 5.08 (s, 2H), δ 3.89 (s, 3H), δ 3.76 (s, 3H), δ 1.42 (s, 12H); 13C NMR (CDCl3, 100 MHz): δ 167.9, 163.9, 160.8, 136.6, 135.1, 128.8, 128.3, 127.9, 105.9, 103.4, 84.0, 70.4, 55.9, 52.6, 29.9, 25.1; HRMS (ESI): calcd for [C22H27BO6 + H]+ 399.1979, found 399.1982.
Methyl 5-(benzyloxy)-3-methoxy-2-(tributylstannyl)benzoate 25
Aromatic bromide 23 (1.5 g, 4.27 mmol) was dissolved in 85 mL dry dioxane followed by addition of LiCl (362.1 mg, 8.54 mmol). The reaction mixture was then cooled to –78 ºC, vacuum degassed and back filled with argon. To this degassed reaction mixture, added hexabutylditin (4.3 mL, 8.54 mmol), a premixed solution of Pd2(dba)3•CHCl3 (155 mg, 0.11 mmol) and PCy3 (180.8 mg, 0.43 mmol) in 5 mL dioxane. The reaction mixture was refluxed for 10 h under argon. The reaction was cooled to 0 ºC and diluted with Et2O and quenched with saturated aqueous NaHCO3. The organic layer was extracted with Et2O (100 mL X 2). The pooled organic layer was subsequently washed with saturated brine and dried over Na2SO4. After removal of the solvent under reduced pressure, the residue was subjected to flash column chromatography using silica gel neutralized with Et3N. Elution with 10% hexane-Et2O gave stannane 25 (1.56 g, 65%) as a colorless oil: Rf (10% hexanes/EtOAc) = 0.80; IR (thin film, cm-1) ν 2950, 2920, 2854, 1740, 1684, 1456, 1378, 1280, 1237, 1169, 1146, 1138, 1048, 1023, 862; 1H NMR (CDCl3, 400 MHz): δ 7.34-7.48 (m, 5H) δ 7.26 (d, J = 2.0 Hz, JSn-H = 6.4 Hz, 1H), δ 6.65 (d, J = 2.4 Hz, JSn-H = 6.0 Hz, 1H), δ 5.11 (s, 2H), δ 3.89 (s, 3H), d 3.75 (s, 3H), δ 1.46-1.54 (m, 6H), δ 1.29-1.38 (m, 6H), δ 1.02-1.06 (m, 6H), δ 0.91 (t, J = 6.4 Hz, 9H); 13C NMR (CDCl3, 100 MHz): δ 169.1, 166.0, 160.0, 139.3, 136.9, 128.8, 128.3, 127.9, 125.8, 107.2 (3JSn-13C = 11.8 Hz), 102.1 (3JSn-13C = 12.0 Hz), 70.4, 55.4, 52.5, 29.4 (3JSn-13C = 10.8 Hz), 27.6 (2JSn-13C = 32.4 Hz), 13.9, 12.9 (1JSn-13C = 182.6 Hz); HRMS (ESI): calcd for [C28H42O4Sn + H]+ 563.2183, found 563.2182.
Methyl 5-(benzyloxy)-2-(5-hydroxy-1,4-dioxo-1,4-dihydronaphthalen-2-yl)-3-methoxybenzoate 27
A suspension of juglone 26 (398.73 mg, 1.58 mmol), stannane 25 (1.15 g, 2.05 mmol), and CuI (60.04 mg, 0.32 mmol) in 18 mL dry THF at rt was vacuum degased and backfilled with argon. To this mixture, added a solution of Pd2(dba)3•CHCl3 (81.8 mg, 0.08 mmol) and PPh3 (83.1 mg, 0.32 mmol) in THF (2 mL) under argon. The mixture was stirred at 80 ºC for 4 h. It was then cooled down to 0 ºC, diluted with EtOAc (50 mL), and quenched by adding saturated aqueous NaHCO3 (20 mL). The mixture was stirred at 0 ºC for 30 min. After separation of the two phases, the aqueous layer was extracted with EtOAc twice and the combined organic layer was washed with saturated brine, and dried over Na2SO4. After removal of the solvent, the residue was subjected to silica gel column chromatography, eluting with 8-10% hexane/EtOAc afforded coupled product 27 (548 mg, 78%).
Alternative method: Bromojuglone 26 (16.8 mg, 0.07 mmol) and borate 24 (32 mg, 0.08 mmol) was dissolved in 0.8 mL THF/H2O (9:1). To this added Cs2CO3 (65 mg, 0.2 mmol), Pd(dppf)2•Cl2•CH2Cl2 (3.0 mg, 0.003 mmol). The reaction mixture was vacuum degassed and refluxed under argon overnight. Diluted with EtOAc (5.0 mL), and quenched by adding saturated aqueous NH4Cl (10 mL). The mixture was stirred at 0 ºC for 30 min. The aqueous layer was extracted with EtOAc twice and the combined organic layer was washed with saturated brine, and dried over Na2SO4. After removal of the solvent, the residue was subjected to silica gel column chromatography, eluting with 8-10% hexane/EtOAc afforded product 27 (9.3 mg, 30%). Reddish-orange solid; mp 172 ºC; (Rf 15% hexanes/EtOAc)= 0.45; IR (thin film, cm-1) ν 3325, 3060, 2975, 2919, 2903, 2866, 2842, 1654, 1578, 1446, 1379, 1342, 1290, 1258, 1241, 1148, 1050, 916; 1H NMR (CDCl3, 400 MHz): δ 12.15 (s, 1H), δ 7.60-7.67 (m, 3H), δ 7.37-7.48 (m, 5H), δ 7.29 (d, J = 2.4 Hz, 1H), δ 6.82 (s, 1H), δ 6.80 (d, J = 2.4 Hz, 1H), δ 5.14 (s, 2H), δ 3.74 (s, 3H), δ 3.72 (s, 3H); 13C NMR (CDCl3, 100 MHz): δ 190.7, 183.8, 166.7, 161.4, 160.5, 158.3, 148.8, 136.3, 135.9, 132.7, 132.1, 128.9, 128.6, 127.9, 124.1, 119.7, 117.1, 115.6, 107.2, 103.8, 70.7, 65.3, 52.6; HRMS (ESI): calcd for [C26H20O7 + H]+ 445.1287, found 445.1277.
ACKNOWLEDGEMENTS
We are grateful to NIH (GM090259) and the NSF (CHE-1213596) for their financial support of our research program.
References
1. This paper is dedicated to Professor Victor Snieckus on the occasion of his 77th birthday.
2. a) S. W. Ayer, A. G. McInnes, P. Thibault, J. A. Walter, J. L. Doull, T. Parnell, and L. C. Vining, Tetrahedron Lett., 1991, 32, 6301; CrossRef b) N. Hirayama, K. Takahashi, K. Shirahata, Y. Ohashi, and Y. Sasada, Bull. Chem. Soc. Jpn., 1981, 54, 1338; CrossRef c) J. Rohr and R. Thiericke, Nat. Prod. Rep., 1992, 9, 103. CrossRef
3. a) F. Tomita, K. Takahashi, and T. Tamaoki, J. Antibiot., 1982, 35, 1038; CrossRef b) Y. Li, X. Huang, K. Ishida, A. Maier, G. Kelter, Y. Jiang, G. Peschel, K. Menzel, M. Li, M. Wen, L. Xu, S. Grabley, H. Fiebig, C. Jiang, C. Hertweck, and I. Sattler, Org. Biomol. Chem., 2008, 6, 3601; CrossRef c) M. Shepherd, T. Liu, C. Mendez, J. Salas, and J. Rohr, Appl. Environ. Microbiol., 2011, 77, 435; CrossRef d) N. Tibrewal, T. E. Downey, S. G. Van Lanen, E. U. Sharif, G. A. O’Doherty, and J. Rohr, J. Am. Chem. Soc., 2012, 134, 12402; CrossRef e) P. Pahari, M. K. Kharel, M. D. Shepherd, S. G. van Lanen, and J. Rohr, Angew. Chem. Int. Ed., 2012, 51, 1216; CrossRef f) M. K. Kharel, P. Pahari, M. D. Shepherd, N. Tibrewal, S. E. Nybo, K. A. Shaaban, and J. Rohr, Nat. Prod. Rep., 2012, 29, 264. CrossRef
4. a) T. Matsumoto, T. Hosoya, and K. Suzuki, J. Am. Chem. Soc., 1992, 114, 3569; b) A. D. Patten, N. H. Nguyen, and S. J. Danishefsky, J. Org. Chem., 1988, 53, 1003; CrossRef c) K. A. Parker and C. A. Coburn, J. Org. Chem., 1991, 56, 1667; d) C. A. James and V. Snieckus, J. Org. Chem., 2009, 74, 4080; CrossRef e) I. Takemura, K. Imura, T. Matsumoto, and K. Suzuki, Org. Lett., 2004, 6, 2503; CrossRef f) P. R. Nandaluru and G. J. Bodwell, J. Org. Chem., 2012, 77, 8028; CrossRef g) A. Patra, P. Pahari, S. Ray, and D. Mal, J. Org. Chem., 2005, 70, 9017; CrossRef h) O. Cortezano-Arellano and A. Cordeo-Vargas, Tetrahedron Lett., 2010, 51, 602; CrossRef i) O. Frutos, C. Atienza, and A. M. Echavarren, Eur. J. Org. Chem., 2001, 163, 163. CrossRef
5. a) R. Oyola, R. Arce, A. E. Alegria, and C. Garcia, Photochem. Photobiol., 1997, 65, 802; CrossRef b) R. M. Knobler, F. B. Radlwimmer, and M. J. Lane, Nucleic Acids Res., 1993, 21, 3920. CrossRef
6. A. Matsumoto and P. C. Hanawalt, Cancer Res., 2000, 60, 3921.
7. a) M. Shan, E. U. Sharif, and G. A. O’Doherty, Angew. Chem. Int. Ed., 2010, 49, 9492; CrossRef b) E. U. Sharif and G. A. O’Doherty, Eur. J. Org. Chem., 2012, 2095. CrossRef
8. C. M. Suter and A. W. Weston, J. Am. Chem. Soc., 1939, 61, 531. CrossRef
9. L. Ying, H. Yang, X. Zhixiang, and C. Xuesong, Tetrahedron: Asymmetry, 2003, 14, 2355. CrossRef
10. M. J. Miller, D. G. Cleary, J. E. Ream, K. R. Snyder, and J. A. Sikorski, Bioorg. Med. Chem., 1995, 3, 1685. CrossRef
11. J. P. Brown, D. H. Johnson, A. Robertson, and W. B. Whalley, J. Chem. Soc., 1951, 2019. CrossRef
12. A. S. Bailey, D. H. Bates, H. R. Ing, and M. A. Warne, J. Chem. Soc., 1952, 4534.
13. F. Kaiser and H. G. Schmalz, Tetrahedron, 2003, 59, 7345. CrossRef
14. F. Sztaricskai, C. M. Harris, A. Neszmelyi, and T. M. Harris, J. Am. Chem. Soc., 1980, 102, 7093. CrossRef
15. A. K. Sinhababu and R. T. Borchardt, J. Org. Chem., 1983, 48, 2356. CrossRef
16. a) T. Ishiyama, M. Murata, and N. Miyaura, J. Org. Chem., 1995, 60, 7508; CrossRef b) M. Kosugi, T. Ohya, and T. Migita, Bull. Chem. Soc. Jpn., 1983, 56, 3855. CrossRef