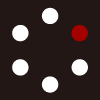
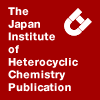
HETEROCYCLES
An International Journal for Reviews and Communications in Heterocyclic ChemistryWeb Edition ISSN: 1881-0942
Published online by The Japan Institute of Heterocyclic Chemistry
e-Journal
Full Text HTML
Received, 8th July, 2013, Accepted, 25th July, 2013, Published online, 5th August, 2013.
DOI: 10.3987/COM-13-12769
■ Bifunctionalized Allenes. Part X. An Electrophilic Cyclization Protocol for Convenient Highly Regioselective Synthesis of 3-Sulfonylfuran-2(5H)-ones from 2-Sulfonylallenoates
Valerij Ch. Christov,* Ivaylo K. Ivanov, and Ismail E. Ismailov
Department of Organic Chemistry & Technology, Konstantin Preslavsky University of Shumen, 115, Universitetska str. BG-9712 Shumen, Bulgaria
Abstract
A simple and convenient protocol for the highly regioselective synthesis of 3-sulfonylfuran-2(5H)-ones by electrophilic cyclization reaction of 2-sulfonylallenecarboxylates with neighbouring ester group participation is described. However, the reaction of 2-sulfonylalka-2,3-dien-1-ones with electrophilic reagents proceeded with formation of 2-sulfonylalka-2,4-dien-1-ones by addition-elimination reaction. A possible mechanism involving the cyclization and addition-elimination reaction of 2-sulfonyl-substituted allenic esters and ketones was proposed.Unsaturated γ-lactones, i. e. butenolides or furan-2(5H)-ones are an important class of compounds because they often occur in natural products and exhibit a broad range of biological activities.1,2 These compounds with different substitution patterns are considered as potential insectides, fungicides, bactericides, antibiotics, anticancer agents, allergy inhibitors, antiinflammatories, antipsoriasis agents, stimulatory agents, cyclooxygenase inhibitors, antimutagen agents, phospholipase A2 inhibitors, etc.2 Furan-2(5H)-ones are also important intermediates in organic synthesis due to the presence of the conjugated C=C bond as well as the five-membered lactone ring. Much attention has been paid to the development of efficient and diverse synthetic methods for construction of this five-membered ring system.3 Among these, cyclization involving allenecarboxylic acids and their derivatives, so called lactonization reaction, is one of the most efficient pathways.4 α-Allenecarboxylic acids and their esters, disubstituted on the γ-carbon atom, underwent electrophilic attack on the central atom and ring closure to 2(5H)-furanones (γ-lactones) when treated with electrophile.4
On the other hand, the reactions of allenyl and diallenyl sulfones with electrophilic reagents have been investigated in the 70s years of the last century. It has been shown5 that depending on the structure of the starting allenic compounds the reactions proceed with ring closure to give 5H-1,2-oxathiole 2-oxides (γ-sultines). Recently, different products of the reactions of the allenyl sulfones with bromine have been reported.4o,6 The reaction of allenyl sulfones with Br2 afforded E-bromohydroxylation- or E-bromination-elimination products highly regio- and stereoselectively depending on the substitution pattern of the allene functionality as the five-membered ionic intermediate with Br3- as the counter ion was isolated.6a Highly selective thiiranation of allenyl sulfones with Br2 in the presence of Na2S2O3 leads to asymmetric synthesis of alkylidenethiiranes.6b In contrast, if the 1-bromo-1-sulfonylallenes are treated with bromine, initially carbenium bromides are generated by attack on the central allenic carbon atom, followed by hydrogen bromide elimination to yield stereospecifically trans-2,3-dibromo-1-sulfonyl-1,3-dienes.6c
As a part of our long-standing studies directed toward the development of efficient electrophilic cyclization reactions of bifunctionalized allenes,7 we become interested in 1,1-bifunctionalized allenes comprising an ester and a sulfonyl group such as A (Scheme 1). Of particular interest are the applications of these groups as temporary transformers of chemical reactivity of the allenic system in the synthesis of eventually heterocyclic compounds. This molecule can be considered as a combination of an allenecarboxylate and an allenyl sulfone and might have different reactivity profiles in electrophilic reactions. Recently, we presented a convenient, efficient and varied method for synthesis of 2-sulfonylated allenecarboxylates, derived by intermediate formation of allenecarboxylates, allenyl sulfones and propargyl sulfinates applying the relatively high acidity of the hydrogen atom at the allenic C-1 atom and the [2,3]-sigmatropic rearrangement.8
It should be pointed out that conceptually there exist two distinct modes of cyclization of the 2-sulfonyl-substituted alka-2,3-dienoates if the electrophilic atom forms a new bond with the central carbon of the allenic system, which seems likely.4-6 It is evident that these pathways are closely connected with the intramolecular neighbouring group participation of the ester and/or the sulfonyl groups as internal nucleophile(s) in the final step of the cyclization. Besides the 5-endo-trig cyclizations11 to the furan-2(5H)-one (butenolide, γ-lactone) B or to the 5H-1,2-oxathiole 2-oxide (γ-sultine) C, electrophilic addition might afford the 3,4-adduct D and the 3,2-adduct E (Scheme 1). This is a part of our general synthetic strategy for investigation of the scope and limitations of the electrophilic cyclization reactions of bifunctionalized allenes. Herein, we wish to report our recent results of these investigations.
We initiated this study with the electrophilic cyclization reaction of the 2-benzenesulfonyl-4-methylpenta-2,3-dienoate 1c with bromine (Table 1). We established that the reaction occurs with cyclization by neighbouring group participation of the only carboxylic group with formation of the 3-benzenesulfonyl-4-bromo-5,5-dimethyl-furan-2(5H)-one 6. We tried to optimize the reaction conditions to get a useful selectivity and the best yield for the furan-2(5H)-one 6 by studying the electrophile equiv., reaction temperature, time and solvent effect. Note that when the reaction was conducted in CH2Cl2 at room temperature, thin-layer chromatography showed that the two reactants still interacted and the reaction was completed within 4 hours with the formation of the desired product 6. It is necessary to carry out this reaction under argon atmosphere since the electrophilic reagents are sensitive to the moisture in air. The desired product 6 was obtained in 42% yield (see Table 1, entry 1). When the reaction was carried out at reflux, it was complete within 3 hours and the yield was considerably lower (34%, entry 2). With 1.5 equiv. of bromine in CH2Cl2 and CHCl3, the yield is lower (entries 3, 4 and 5). Polar solvents such as ethanol, acetonitrile, and nitromethane gave low yields, even with longer reaction times (6-8 hours) and mainly recovered starting materials (entries 6, 7, and 8, respectively). Similar yields were obtained in hydrocarbons as solvents (entries 9 and 10). Fortunately, when 1,2-dichloroethane was used as solvent at rt and minus temperatures for 5-8 hours, the yield improved to 77% (entries 11-14). It should be noted that temperature of -20 °C is the best and most convenient: when the temperature is higher or lower, the yield is worse (compare entries 1-3, 6 and 9-11 with entries 4, 5, 7, 8, 12-14, Table 1). When 1.2 equiv. of electrophilic reagent were used, the reaction was higher yielding (compare entries 11-14, Table 1). We therefore, conducted the remainder of the reactions in 1,2-dichloroethane at -20 °C using 1.0 equiv. of the 2-sulfonyl-allenecarboxylates 1a-h and 1.2 equiv. of the electrophilic reagent for 5 hours. The cyclic product 6 was fully characterized by means of NMR (1H, and 13C), and IR spectroscopy.
Having determined the optimized reaction conditions in hand, we explored the score of the lactonization reaction of the 2-sulfonylallenoates 1a-h and the results that we obtained are summarized in Table 2. It should be noted that the reaction under this set of standard reaction conditions in the favored 5-endo-trig mode affords the 3-sulfonylfuran-2(5H)-ones 3-12 in good to excellent yields irrespective of the nature of the substituents on the allenic system and sulfone group. The reaction scope is wide: R can be methyl, CCl3, or phenyl, R1 can be methyl, R2 can be H or methyl, also R1+R2 can be -(CH2)5-, R3 can be H, or methyl, and E can be Cl, Br, PhS, and PhSe.
To establish the generality of this methodology, the reaction of the 2-sulfonylalka-2,3-dien-1-ones 2a,c-e,g,h with different electrophilic reagents such as sulfuryl chloride, bromine, benzenesulfenyl chloride and benzeneselenyl chloride was examined. To our surprise, when we applied the current standard conditions to 1,1-bifunctionalized allenes comprising a carbonyl and a sulfonyl group such as 2 (Table 3), instead of the 3-sulfonylfuran-2(5H)-ones 3-12, the acyclic compounds 13-18 were isolated in 72-76% yield after stirring for 5 hours at -20 °C and for one hour to rt. The results were summarized in Table 3.
Interestingly, this protocol can also be successfully applied to the electrophilic reaction of the 2-sulfonyl-substituted allenyl ketones 2a,c-e,g,h which afforded the (2Z)-2-sulfonylalka-2,4-dien-1-ones 13-18 highly regio- and stereoselectively, indicating a further
elimination reaction following the addition reaction of electrophiles yielding the second carbon-carbon double bond highly chemoselectively.
Thus, on the basis of the literature data10 and our previous results,7 a rationale for this reaction is depicted in Scheme 2. The initial act is the attack of the electrophile (Cl+, Br+, S+ or Se+) on the most nucleophilic atom of the allenic system of π-bonds (C3) with the formation of the cyclic onium (chloronium, bromonium, thiiranium or seleniranium) ions A after attack on the relatively electron-rich C3-C4-double bond. Subsequently, the ions A are easily transformed into the more stable five-membered cyclic ions B via neighbouring group participation of the oxygen atom of the carbonyl functionality (path a). When R4 is OEt, the intermediates B undergo a nucleophilic attack to the EtO group and elimination of ethyl halide affording the final cyclic products 3-12 (path c). On the other hand, in the case of the sulfonyl-substituted allenyl ketones 2 (R is Me, CCl3 or Ph) as starting materials, the formation of the final sulfonyl-2,4-dienyl ketones 13-18 can be considered in terms of the assumptions for the nucleophilic attack on the cyclic three-membered onium ions A (path b) or on the cyclic five-membered carbenium ions B (path d) and following elimination of hydrogen halide. The stereoselectivity observed may be explained by the favorable trans arrangement of the electrophile and the carbonyl group in the (2Z)-2-sulfonylalka-2,4-dien-1-ones 13-18.
In conclusion, we have developed a simple and convenient protocol for the electrophilic interaction of 2-sulfonylallenoates and allenyl ketones affording 3-sulfonylfuran-2(5H)-ones by cyclization with the neighbouring ester group participation of the 2-sulfonyl-allenecarboxylates and 2-sulfonylalka-2,4-dien-1-ones by addition-elimination reaction of the 2-sulfonyl-substituted allenyl ketones with excellent regio- and stereoselectivity. Due to the easy availability of starting materials, the convenient operation and the usefulness of the butenolide and dienic products, the reaction may show potentials and will be useful in organic synthesis. Further studies on the synthetic applications of this reaction and the physiological activity of selected cyclic and acyclic products are now under investigation in our laboratory. Furthermore, a continuation of these studies towards the synthesis and electrophilic cyclization reactions of other bifunctionalized allenes is currently in progress in our laboratory.
EXPERIMENTAL
All new synthesized compounds were purified by column chromatography and characterized on the basis of NMR, IR and microanalytical data. NMR spectra were recorded on DRX Brucker Avance-250 (Bruker BioSpin GmbH, Karlsruhe, Germany) (1H at 250.1 MHz, 13C at 62.9 MHz) and Brucker Avance II+600 (Bruker BioSpin GmbH, Karlsruhe, Germany) (1H at 600.1 MHz, 13C at 150.9 MHz) spectrometers for solutions in CDCl3. Chemical shifts are in parts per million downfield from internal TMS. J values are given in Hertz. IR spectra were recorded with an FT-IRAfinity-1 Shimadzu spectrophotometer (Shimadzu Corp., Japan). Elemental analyses were carried out by the Microanalytical Service Laboratory of Faculty of Chemistry and Pharmacy, University of Sofia using Vario EL3 CHNS(O) (Elementar Analysensysteme GmbH, Hanau, Germany). Column chromatography was performed on Kieselgel F25460 (70-230 mesh ASTM, 0.063-0.200 nm, Merck). The melting points were measured in open capillary tubes and are uncorrected. The solvents were purified by standard methods. Reactions were carried out in oven-dried glassware under an argon atmosphere and exclusion of moisture. All compounds were checked for purity on TLC plates Kieselgel F25460, Merck. Benzenesulfenyl chloride was prepared from diphenyl disulfide and sulfuryl chloride in dichloromethane and distilled in vacuo (bp 80-81 °C/20 mm Hg) before used.9 Diphenyl disulfide, sulfuryl chloride, and benzeneselenyl chloride were commercially available and used without purification.
General procedure for the reactions of the 1 and 2 with electrophilic reagents.
To a solution of the allene 1 or 2 (3 mmol) in dry 1,2-dichloroethane (5 mL) at -20 °C was added dropwise with stirring a solution of electrophilic reagent (sulfuryl chloride, bromine, benzenesulfenyl chloride, or benzeneselenyl chloride) (3.6 mmol) in the same solvent (5 mL). The reaction mixture was stirred for 5 h at the same temperature and for 1 h to rt. The solvent was removed using a rotatory evaporator and the residue was purified by column chromatography on silica gel (Kieselgel Merck 60 F254) with EtOAc/hexane.
4-Chloro-3-methanesulfonyl-5,5-dimethylfuran-2(5H)-one (3)
This compound was obtained as yellow oil, yield 74%. Eluent for TLC: EtOAc : hexane = 4:1, Rf 0.51; IR (neat): 1751 (C=O), 1596 (C=C), 1325, 1146 (SO2), 1076 (C-O-C). 1H NMR (250.1 MHz): δH 1.69 (s, 6H, 2Me), 3.51 (s, 3H, MeSO2). 13C NMR (62.9 MHz): δC 25.5 (Me), 42.7 (Me), 94.8 (C), 135.0 (C), 171.4 (C), 175.3 (C). Anal. Calcd for C7H9SO4Cl requires: C 37.42, H 4.04. Found: C 37.53, H 4.17.
4-Benzenesulfenyl-3-methanesulfonyl-5,5-dimethylfuran-2(5H)-one (4)
This compound was obtained as yellow oil, yield 73%. Eluent for TLC: EtOAc : hexane = 4:1, Rf 0.68. IR (neat) (νmax, cm-1): 1749 (C=O), 1599 (C=C), 1494, 1441 (Ph), 1329, 1153 (SO2), 1079 (C-O-C). 1H NMR (600.1 MHz): δH 1.80 (s, 6H, 2Me), 3.41 (s, 3H, MeSO2), 7.10-7.71 (m, 5H, Ph). 13C NMR (150.9 MHz): δC 28.6 (Me), 41.9 (Me), 89.1 (C), 125.4, 125.8, 130.1, 135.4 (Ph), 127.4 (C), 169.3 (C), 180.4 (C). Anal. Calcd for C13H14S2O4 requires: C 52.33, H 4.73. Found: C 52.27, H 4.83.
4-Bromo-5,5-dimethyl-3-trichloromethanesulfonylfuran-2(5H)-one (5)
This compound was obtained as light yellow oil, yield 76%. Eluent for TLC: EtOAc : hexane = 4:1, Rf 0.60. IR (neat) (νmax, cm-1): 1748 (C=O), 1603 (C=C), 1330, 1157 (SO2), 1075 (C-O-C). 1H NMR (250.1 MHz): δH 1.75 (s, 6H, 2Me). 13C NMR (62.9 MHz): δC 27.4 (Me), 90.2 (C), 109.1 (C), 136.0 (C), 165.7 (C), 166.7 (C). Anal. Calcd for C7H6SO4Cl3Br requires: C 22.57, H 1.62. Found: C 22.72, H 1.71.
3-Benzenesulfonyl-4-bromo-5,5-dimethylfuran-2(5H)-one (6)
This compound was obtained as pale orange crystals, mp 141-142 °C, yield 77%. Eluent for TLC: EtOAc : hexane = 4:1, Rf 0.56. IR (KBr) (νmax, cm-1): 1753 (C=O), 1595 (C=C), 1490, 1437 (Ph), 1328, 1147 (SO2), 1080 (C-O-C). 1H NMR (600.1 MHz): δH 1.77 (s, 6H, 2Me), 7.51-8.30 (m, 5H, Ph). 13C NMR (150.9 MHz): δC 27.5 (Me), 90.6 (C), 127.4, 130.7, 134.5, 136.8 (Ph), 137.4 (C), 165.5 (C), 172.4 (C). Anal. Calcd for C12H11SO4Br requires: C 43.52, H 3.35. Found: C 43.66, H 3.29.
4-Chloro-5-isopropyl-3-methanesulfonyl-5-methylfuran-2(5H)-one (7)
This compound was obtained as yellow oil, yield 74%. Eluent for TLC: EtOAc : hexane = 4:1, Rf 0.74. IR (neat) (νmax, cm-1): 1754 (C=O), 1597 (C=C), 1330, 1140 (SO2), 1076 (C-O-C). 1H NMR (600.1 MHz): δH 0.93 (d, J 1.0 Hz, 3H, CHMe2), 1.16 (d, J 1.0 Hz, 3H, CHMe2), 1.71 (m, 1H, CHMe2), 1.76 (s, 3H, Me), 3.53 (s, 3H, MeSO2). 13C NMR (150.9 MHz): δC 17.1 (Me), 17.8 (Me), 22.5 (Me), 38.1 (CH), 44.1 (Me), 96.9 (C), 135.2 (C), 170.8 (C), 171.4 (C). Anal. Calcd for C9H13SO4Cl requires: C 42.77, H 5.18. Found: C 42.83, H 5.17.
4-Bromo-5-isopropyl-5-methyl-3-trichloromethanesulfonylfuran-2(5H)-one (8)
This compound was obtained as yellow oil, yield 73%. Eluent for TLC: EtOAc : hexane = 4:1, Rf 0.72. IR (neat) (νmax, cm-1): 1752 (C=O), 1598 (C=C), 1336, 1142 (SO2), 1076 (C-O-C). 1H NMR (600.1 MHz): δH 0.94 (d, J 1.0 Hz, 3H, CHMe2), 1.15 (d, J 1.0 Hz, 3H, CHMe2), 1.60 (m, 1H, CHMe2), 1.84 (s, 3H, Me). 13C NMR (150.9 MHz): δC 16.8 (Me), 17.7 (Me), 21.9 (Me), 37.0 (CH), 97.2 (C), 108.4 (C), 136.5 (C), 163.7 (C), 166.8 (C). Anal. Calcd for C9H10SO4Cl3Br requires: C 26.99, H 2.52. Found: C 27.05, H 2.67.
4-Benzeneselenyl-3-benzenesulfonyl-5-isopropyl-5-methylfuran-2(5H)-one (9)
This compound was obtained as yellow crystals, mp 148-149 °C, yield 70%. Eluent for TLC: EtOAc : hexane = 4:1, Rf 0.79. IR (KBr) (νmax, cm-1): 1751 (C=O), 1600 (C=C), 1493, 1441 (Ph), 1320, 1139 (SO2), 1076 (C-O-C). 1H NMR (600.1 MHz): δH 0.86 (d, J 1.0 Hz, 3H, CHMe2), 1.09 (d, J 1.0 Hz, 3H, CHMe2), 1.49 (m, 1H, CHMe2), 1.71 (s, 3H, Me), 7.40, 7.58, 7.80, 7.93, 8.13 (m, 10H, 2Ph). 13C NMR (150.9 MHz): δC 15.3 (Me), 16.0 (Me), 20.4 (Me), 33.4 (CH), 100.1 (C), 126.5, 128.7, 129.1, 129.5, 131.1, 133.9, 137.4, 138.4 (2Ph), 129.4 (C), 173.6 (C), 183.4 (C). Anal. Calcd for C20H20SO2Se requires: C 55.17, H 4.63. Found: C 55.22, H 4.76.
4-Benzenesulfenyl-3-methanesulfonyl-1-oxaspiro[4,5]dec-3-en-2-one (10)
This compound was obtained as orange crystals, mp 161-162 °C, yield 72%. Eluent for TLC: EtOAc : hexane = 4:1, Rf 0.82. IR (KBr) (νmax, cm-1): 1754 (C=O), 1597 (C=C), 1318, 1138 (SO2), 1487, 1440 (Ph), 1077 (C-O-C). 1H NMR (600.1 MHz): δH 1.50-2.08 (m, 10H, -(CH2)5-), 3.42 (s, 3H, MeSO2), 7.18, 7.70, 7.91 (m, 5H, Ph). 13C NMR (150.9 MHz): δC 20.9 (CH2), 23.4 (CH2), 38.2 (CH2), 43.2 (Me), 85.4 (C), 125.4, 125.9, 131.7, 137.3 (Ph), 127.4 (C), 170.6 (C), 182.8 (C). Anal. Calcd for C16H18S2O4 requires: C 56.78, H 5.36. Found: C 56.87, H 5.49.
3-Benzenesulfonyl-4-chloro-1-oxaspiro[4,5]dec-3-en-2-one (11)
This compound was obtained as yellow oil, yield 77%. Eluent for TLC: EtOAc : hexane = 4:1, Rf 0.75. IR (neat) (νmax, cm-1): 1751 (C=O), 1594 (C=C), 1489, 1429 (Ph), 1320, 1146 (SO2), 1079 (C-O-C). 1H NMR (600.1 MHz): δH 1.48-2.10 (m, 10H, -(CH2)5-), 7.40, 7.58, 8.24 (m, 5H, Ph). 13C NMR (150.9 MHz): δC 22.9 (CH2), 23.7 (CH2), 37.5 (CH2), 90.6 (C), 129.1, 131.0, 134.7, 138.1 (Ph), 127.4 (C), 170.5 (C), 178.5 (C). Anal. Calcd for C15H15SO4Cl requires: C 55.13, H 4.63. Found: C 53.22, H 4.71.
4-Benzeneselenyl-3-benzenesulfonyl-1-oxaspiro[4,5]dec-3-en-2-one (12)
This compound was obtained as pale orange crystals, mp 172-173 °C, yield 75%. Eluent for TLC: EtOAc : hexane = 4:1, Rf 0.78. IR (KBr) (νmax, cm-1): 1748 (C=O), 1604 (C=C), 1487, 1429 (Ph), 1322, 1139 (SO2), 1077 (C-O-C). 1H NMR (600.1 MHz): δH 1.49-2.06 (m, 10H, -(CH2)5-), 7.20, 7.51, 7.77, 7.82, 7.92, 8.50 (m, 10H, 2Ph). 13C NMR (150.9 MHz,): δC 21.7 (CH2), 24.2 (CH2), 38.4 (CH2), 85.5 (C), 125.0, 125.7, 128.1, 131.4, 131.8, 134.7, 137.5, 137.8 (Ph), 124.7 (C), 172.1 (C), 188.2 (C). Anal. Calcd for C21H20SO4Se requires: C 56.37, H 4.51. Found: C 56.48, H 4.66.
(3Z)-4-Bromo-3-methanesulfonyl-5-methylhexa-3,5-dien-2-one (13)
This compound was obtained as yellow oil, yield 73%. Eluent for TLC: EtOAc : hexane = 3:1, Rf 0.66. IR (neat) (νmax, cm-1): 1673 (C=O), 1625, 1606 (C=C), 1308, 1137 (SO2). 1H NMR (600.1 MHz): δH 2.20 (dd, Jcis 1.4 Hz, Jtrans 0.9 Hz, 3H, Me-C=C), 2.64 (s, 3H, Me-C=O), 3.27 (s, 3H, MeSO2), 5.42 (m, 1H, =CH), 5.51 (m, 1H, =CH). 13C NMR (150.9 MHz): δC 21.4 (Me), 28.2 (Me), 41.4 (Me), 129.1 (C), 137.4 (C), 143.0 (C), 148.5 (C), 187.4 (C). Anal. Calcd for C8H11SO3Br requires: C 35.97, H 4.15. Found: Found: C 36.12, H 4.27.
(3Z)-3-Benzenesulfonyl-4-chloro-5-methylhexa-3,5-dien-2-one (14)
This compound was obtained as yellow oil, yield 76%. Eluent for TLC: EtOAc : hexane = 3:1, Rf 0.72. IR (neat) (νmax, cm-1): 1677 (C=O), 1624, 1608 (C=C), 1479, 1437 (Ph), 1313, 1138 (SO2). 1H NMR (600.1 MHz,): δH 1.98 (dd, Jcis 1.5 Hz, Jtrans 0.9 Hz, 3H, Me-C=C), 2.63 (s, 3H, Me-C=O), 5.22 (m, 1H, =CH), 5.60 (m, 1H, =CH), 7.60-8.10 (m, 5H, Ph). 13C NMR (150.9 MHz): δC 20.2 (Me), 28.6 (Me), 128.5 (C), 130.5, 131.1, 134.5, 137.6 (Ph), 140.5 (C), 142.5 (C), 146.5 (C), 189.3 (C). Anal. Calcd for C13H13SO3Cl requires: C 54.83, H 4.60. Found: C 54.95, H 4.75.
(3Z)-4-Chloro-3-methanesulfonyl-5,6-dimethylhepta-3,5-dien-2-one (15)
This compound was obtained as yellow oil, yield 75%. Eluent for TLC: EtOAc : hexane = 3:1, Rf 0.71. IR (neat) (νmax, cm-1): 1679 (C=O), 1628, 1610 (C=C), 1314, 1142 (SO2). 1H NMR (600.1 MHz): δH 1.77 (m, 3H, Me), 1.81 (m, 3H, Me), 1.94 (m, 3H, Me), 2.63 (s, 3H, Me-C=O), 3.31 (s, 3H, MeSO2). 13C NMR (150.9 MHz): δC 15.5 (Me), 20.8 (Me), 21.9 (Me), 28.3 (Me), 42.5 (Me), 135.6 (C), 138.5 (C), 140.3 (C), 144.7 (C), 187.4 (C). Anal. Calcd for C10H15ClO3S requires: C 47.90, H 6.03. Found: C 48.03, H 6.12.
(2Z)-3-Bromo-4,5-dimethyl-1-phenyl-2-trichloromethanesulfonylhexa-2,4-dien-1-one (16)
This compound was obtained as yellow oil, yield 74%. Eluent for TLC: EtOAc : hexane = 3:1, Rf 0.67. IR (neat) (νmax, cm-1): 1679 (C=O), 1625, 1611 (C=C), 1308, 1143 (SO2). 1H NMR (600.1 MHz): δH 1.81 (m, 3H, Me), 1.88 (m, 3H, Me), 2.01 (m, 3H, Me), 7.81, 7.84, 8.15 (m, 5H, Ph). 13C NMR (150.9 MHz): δC 15.3 (Me), 20.4 (Me), 21.8 (Me), 108.2 (C), 130.5, 131.4, 134.7, 143.3 (Ph), 132.5 (C), 138.0 (C), 139.1 (C), 141.4 (C), 177.4 (C). Anal. Calcd for C15H14SO3Cl3Br requires: C 39.11, H 3.06. Found: C 39.22, H 3.17.
(3Z)-4-Benzenesulfenyl-4-cyclohex-1-enyl-3-methanesulfonylbut-3-en-2-one (17)
This compound was obtained as yellow oil, yield 73%. Eluent for TLC: EtOAc : hexane = 3:1, Rf 0.61. IR (neat) (νmax, cm-1): 1678 (C=O), 1627, 1609 (C=C), 1475, 1434 (Ph), 1309, 1140 (SO2). 1H NMR (600.1 MHz): δH 1.56, 1.87, 2.22, 2.39 (m, 8H, -(CH2)4-), 2.44 (s, 3H, Me-C=O), 3.22 (s, 3H, MeSO2), 6.87 (m, 1H, H-C=), 7.14, 7.63, 7.77 (m, 5H, Ph). 13C NMR (150.9 MHz): δC 22.3 (CH2), 23.8 (CH2), 26.5 (CH2), 28.5 (CH3), 29.6 (CH2), 43.3 (Me), 126.3, 128.0, 133.6, 136.4 (Ph), 135.1 (C), 136.2 (C), 140.4 (C), 142.3 (C), 184.7 (C). Anal. Calcd for C17H20S2O3 requires: C 60.68, H 5.99. Found: C 60.80, H 6.12.
(2Z)-3-Benzeneselenyl-2-benzenesulfonyl-3-cyclohex-1-enyl-1-phenylpropenone (18)
This compound was obtained as yellow crystals, Mp 164-165 °C, yield 72%. Eluent for TLC: EtOAc : hexane = 3:1, Rf 0.60. IR (KBr) (νmax, cm-1): 1681 (C=O), 1623, 1609 (C=C), 1475, 1434 (Ph), 1311, 1144 (SO2). 1H NMR (600.1 MHz): δH 1.47, 1.56, 1.95, 2.26 (m, 8H, -(CH2)4-), 6.67 (m, 1H, H-C=), 7.46, 7.60, 7.69, 7.75, 7.81, 7.84, 7.87, 7.97, 8.01 (m, 15H, 3Ph). 13C NMR (150.9 MHz): δC 21.5 (CH2), 23.2 (CH2), 24.1 (CH2), 29.5 (CH2), 112.2 (C), 131.4 (C), 139.6 (C), 129.3, 129.8, 130.5, 131.4, 131.5, 131.6, 132.0, 133.6, 135.7, 138.1, 139.4, 140.1 (3Ph), 149.3 (C), 191.4 (C). Anal. Calcd for C27H24SO3Se requires: C 63.90, H 4.77. Found: C 63.82, H 4.88.
ACKNOWLEDGMENTS
We gratefully acknowledge the financial support by Research Fund of the Konstantin Preslavsky University of Shumen (Project No. RD-08-243/2013), National Research Fund of Bulgaria (Project No. DRNF-02-13/2009), and Human Resources Development Operational Programme of the European Union (Project No. BG051PO001-3.3.06-0003/2012). Special thanks to MSc. Boris Prodanov and MSc. Padost Nikolova for the technical help in the chromatographical separations.
References
1. F. M. Dean, ‘Naturally Occurring Oxygen Ring Compounds’, Butterworths, Washington, DC, 1963, pp. 53-81.
2. (a) M. Uchida, Y. Koike, G. Kusano, Y. Kondo, S. Nozoe, C. Kabuto, and T. Takemoto, Chem. Pharm. Bull., 1980, 28, 92; CrossRef (b) K. Kakinuma, J. Koike, K. Ishibashi, W. Takahashi, and H. Takei, Agric. Biol. Chem., 1986, 50, 625; CrossRef (c) L. J. Reynolds, B. P. Morgan, G. A. Hite, E. D. Mihelich, and E. A. Dennis, J. Am. Chem. Soc., 1988, 110, 5172; CrossRef (d) A. Tanabe, Jpn. Kokai Tokkyo. Koho JP 63,211,276 [88,211,276], 1988 (Chem. Abstr., 1989, 110, 94978q); (e) G. C. M. Lee, Eur. Pat. Appl. EP 372,940, 1990 (Chem. Abstr., 1990, 113, 191137j); (f) T. S. Brima, US Pat. 4,968,817, 1990 (Chem. Abstr., 1991, 114, 185246y); (g) G. C. M. Lee and M. E. Garst, PCT Int Appl WO 91 16,055, 1991 (Chem. Abstr., 1992, 116, 59197m); (h) J. N. Gnabre, J. L. Pinnas, D. G. Martin, S. A. Mizsak, D. A.Kloosterman, L. Baczynskyj, J. W. Nielsen, R. B. Bates, J. J. Hoffmann, and V. V. Kane, Tetrahedron, 1991, 47, 3545; CrossRef (i) G. M. Konig, A. D. Wright, O. Sticher, C. K. Angerhofer, and J. M. Pezzuto, Planta Med., 1994, 60, 532; CrossRef (j) Y. Ducharme, J. Y. Gauthier, P. Prasit, Y. Leblanc, Z. Wang, S. Leger, and M. Therien, PCT Int Appl WO 95 00,501, 1995 (Chem. Abstr., 1996, 124, 55954y); (k) W. C. Patt, J. J. Edmunds, J. T. Repine, K. A. Berryman, B. R. Reisdorph, C. Lee, M. S. Plummer, A. Shahripour, S. J. Haleen, J. A. Keiser, M. A. Flynn, K. M. Welch, E. E. Reynolds, R. Rubin, B. Tobias, H. Hallak, and A. M. Doherty, J. Med. Chem., 1997, 40, 1063; CrossRef (l) Z. Jiang and D.-Q. Yu, J. Nat. Prod., 1997, 60, 122; CrossRef (m) M. Resch, A. Steigel, Z.-l. Chen, and R. Bauer, J. Nat. Prod., 1998, 61, 347; CrossRef (n) K. Yoneyama, Y. Takeuchi, M. Ogasawara, M. Konnai, Y. Sugimoto, and T. Sassa, J. Agric. Food. Chem., 1998, 46, 1583; (o) G. M. Konig, A. D. Wright, and S. G. Franzblau, Planta Med., 2000, 66, 337; CrossRef (p) D. Tasdemir, G. P. Concepcion, G. C. Mangalindan, M. K. Harper, E. Hajdu, and C. M. Ireland, Tetrahedron, 2000, 56, 9025. CrossRef
3. For reviews, see: (a) Y. S Rao, Chem. Rev., 1964, 64, 353; CrossRef (b) Y. S. Rao, Chem. Rev., 1976, 76, 625; CrossRef (c) D. W. Knight, Contemp. Org. Synth., 1994, 287. CrossRef
4. (a) E. P. Kohler and W. J. Whitcher, J. Am. Chem. Soc., 1940, 62, 1489; CrossRef (b) G. Asknes and P. Froyen, Acta Chem. Stand., 1968, 22, 2347; CrossRef (c) G. Kresze, W. Runge, and E. Ruth, Liebigs Ann. Chem., 1972, 756, 112; CrossRef (d) S. Musierowicz, A. Wroblewski, and H. Krawczyk, Tetrahedron Lett., 1975, 16, 437; CrossRef (e) G. Kresze, L. Kloimstein, and W. Runge, Liebigs Ann. Chem., 1976, 979; CrossRef (f) G. B. Gill and M. S. H. Idris, Tetrahedron Lett., 1985, 26, 4811; CrossRef (g) K. Shingu, S. Hagishita, and M. Nakagawa, Tetrahedron Lett., 1967, 8, 4371; CrossRef (h) S. Ma and S. Wu, J. Org. Chem., 1999, 64, 9314; CrossRef (i) S. Ma, Z. Shi, and Z. Yu, Tetrahedron, 1999, 55, 12137; CrossRef (j) S. Ma and S. Wu, Tetrahedron Lett., 2001, 42, 4075; CrossRef (k) S. Ma, F. Pan, X. Hao, and X. Huang, Synlett, 2004, 85; CrossRef (l) C. Fu and S. Ma, Eur. J. Org. Chem., 2005, 3942; CrossRef (m) G. Chen, C. Fu, and S. Ma, Tetrahedron, 2006, 62, 4444; CrossRef (n) C. Zhou, Z. Ma, Z. Gu, C. Fu, and S. Ma, J. Org. Chem., 2008, 73, 772; CrossRef (o) S. Ma, Acc. Chem. Res., 2009, 42, 1679. CrossRef
5. (a) S. Braverman and D. Reisman, Tetrahedron Lett., 1977, 18, 1753; CrossRef (b) S. Braverman and D. Reisman, J. Am. Chem. Soc., 1977, 99, 605; CrossRef (c) S. Braverman and Y. Duar, J. Am. Chem. Soc., 1983, 105, 1061. CrossRef
6. (a) C. Zhou, C. Fu, and S. Ma, Tetrahedron, 2007, 63, 7612; CrossRef (b) C. Zhou, C. Fu, and S. Ma, Angew. Chem. Int. Ed., 2007, 46, 4379; CrossRef (c) R. W. Saalfrank, A. Welch, M. Haubner, and U. Bauer, Liebigs Ann. Chem., 1996, 171. CrossRef
7. (a) V. Ch. Christov, R.Nikolova, and B. Prodanov, Phosphorus, Sulfur Silicon Relat. Elem., 2000, 166, 275; CrossRef (b) V. Ch. Christov and B. Prodanov, Phosphorus, Sulfur Silicon Relat. Elem., 2000, 166, 265; CrossRef (c) V. Ch. Christov and B. Prodanov, Heterocycl. Сommun., 2002, 8, 349; (d) V. Ch. Christov and B. Prodanov, Phosphorus, Sulfur Silicon Relat. Elem., 2002, 177, 243;; CrossRef (e) V. Ch. Christov and B. Prodanov, Heterocycles, 2002, 57, 1777; CrossRef (f) V. Ch. Christov and B. Prodanov, Synth. Commun., 2004, 34, 1577; CrossRef (g) V. Ch. Christov and J. G. Ivanova, Synth. Commun., 2006, 36, 2231. CrossRef
8. I. K. Ivanov and V. Ch. Christov, Phosphorus, Sulfur Silicon Relat. Elem., 2013, 188, 913. CrossRef
9. H. Lecher and F. Holschneider, Ber. 1924, 57, 755.
10. (a) P. B. D. de la Mare and R. Bolton, ‘Electrophilic Addition to Unsaturated Systems’, Elsevier, Amsterdam, 1966, pp. 250-266; (b) M. C. Caserio, ‘Selectivity in Addition Reactions of Allenes’ in ‘Selective Organic Transformations’, ed. by B. S Thyagarajan, John Wiley & Sons, New York, 1970, pp. 239-299; (c) P. B. D. de la Mare and R. Bolton, ‘Electrophilic Addition to Unsaturated Systems’, Elsevier, Amsterdam, 1982, pp. 317-325; (d) T. L. Jacobs, ‘Electrophilic Addition to Allenes’ in ‘The Chemistry of the Allenes’, ed. by S. R. Landor, Academic Press, New York, 1982, Vol. 2, Ch. 5, pp. 417-510; (e) W. Smadja, Chem. Rev., 1983, 83, 263; CrossRef (f) S. Ma, ‘Ionic Addition to Allenes’ in ‘Modern Allene Chemistry’, ed. by N. Krause and A. S. K. Hashmi, Wiley-VCH: Weinheim, 2004, Vol. 2, pp. 595-699. CrossRef
11. J. E. Baldwin, Chem. Commun., 1976, 734; CrossRef 736; CrossRef 738. CrossRef