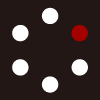
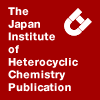
HETEROCYCLES
An International Journal for Reviews and Communications in Heterocyclic ChemistryWeb Edition ISSN: 1881-0942
Published online by The Japan Institute of Heterocyclic Chemistry
e-Journal
Full Text HTML
Received, 10th July, 2013, Accepted, 31st July, 2013, Published online, 31st July, 2013.
DOI: 10.3987/COM-13-12771
■ ONE-POT SYNTHESIS OF 11,23-BIS(IMIDAZOL-1-YL)CALIX[4]ARENE DERIVATIVES BASED ON THE UPPER RIM OF BIS-AMINOCALIX[4]ARENE
Bing Zhao, Yao-Yu Ruan, Ming-jie Ma, Qi-Gang Deng,* Li-Yan Wang, Ya-Qing Feng, and Yan Gao*
College of Chemistry and Chemical Engineering, Qiqihar University, 42 Wenhuadajie, Qiqihar, Heilongjian Shang, 161006, China
Abstract
A simple one-pot synthesis for a new 11,23-bis(imidazol-1-yl)calix[4]arene derivatives in their cone conformation was reported by the four component condensation reaction of 11,23-bis-aminocalix[4]arene, aldehyde, benzil and ammonium acetate. This work provides a simple, efficient method for the construction of 11,23-bis(imidazol-1-yl)calix[4]arene derivatives on which the substituted imidazole rings are directly linked to their upper rim.Since Gutsche and Muthukrishnan1 reported the one-pot synthesis of calixarene by the condensation reaction between para-substituted phenol and formaldehyde in alkaline medium, the fundamental calixarene frameworks have been widely modified at the phenolic hydroxy groups (lower rim) and at the para position (upper rim). In particular, the remarkable growth of the field of the calixarene supramolecular chemistry as receptors has been greatly motivated by the search for derivatives capable of engaging selective complexation with netural molecule,2-5 cations,6-11 and anions.12-16 In fact, different species and numbers of functional groups and the different position in calixarene molecular scoffold have been integrated on calixarene’s complexation behaviour. Therefore, developing the simple, efficient method for the synthesis of calixarenes and improving their supramolecular properties are still indispensable.
Recently, the green chemical methods have been extensively used in organic synthesis, combinatorial and medical chemistry due to their abilities to improve reaction efficiency, yield, selectivity and experiment conditions, and minimize the number of reaction steps, energy consumption, waste production, and generate large libraries of compounds.17 Currently, microwave irradiation technology (MWI),18 click synthesis19 and multi-component reaction20 (MCRs) have been widely reported in the literatures. However, most of these efficient chemical processes are normally utilized in the construction of smaller pharmaceutical molecules. So far there are only several reports related to their applications in the construction of complex macromolecules, such as calixarene derivatives.21-23 Consequently, mild and expedient protocols for synthesis of calixarene derivatives are of significant importance.
Recently, we have developed a highly efficient procedure for the preparation of 11,23-bis(imidiazol-1-yl)calix[4]arene derivatives on which the substituted imidazole groups are directly linked to their upper rims via one-pot, multi-component reactions based on the bisaminocalix[4]arene compounds. Herein, we report a facile, and convenient one-pot four component route to the synthesis of some novel 11,23-bis(imidiazol-1-yl)calix[4]arene derivatives by the reaction of benzaldehydes, benzil, bis-aminocalix[4]arene and ammonium acetate in the presence of inexpensive acetic acid as the solvent without any catalyst.
11,23-Bisaminocalix[4]arene 1 diprotected on the lower rim was prepared according to the literature.24 11,23-Bis(imidazol-1-yl)calix[4]arene derivatives 5 were synthesized by the reaction of bis-aminocalix[4]arene 1, benzaldehyde 2, benzil and ammonium acetate in the refluxing acetic acid for certain times. The compounds were separated by the recrystallization method with ethyl acetate. The conformational characteristics of 11,23-bis(imidazol-1-yl)calix[4]arene derivatives 5 were conveniently estimated by their 1H and 13C NMR spectroscopy. Take the compound 5a for example. In particular, in their 1H NMR spectra, a typical AB pattern is observed for methylene bridge ArCH2Ar protons and there is a Δδ separation of approximately 1 ppm between the exo and endo geminal protons. Furthermore, the coupling constant for the splitting of ArCH2Ar protons is approximately 13 Hz. The 1H NMR spectra of bis(2,4,5-triphenyl imidazol-1-yl)calix[4]arene 5a showed two signals for the aromatic protons attributed to the matrix rings of calix[4]arene at δ 6.91 and 6.55 and a pair of doublets for the bridging methylene groups at δ 4.18 (J = 13.2 Hz) and 3.17 (J = 13.2 Hz), respectively. The 13C NMR of compound 5a displayed signals at around δ 31 for the bridging methylene carbon atoms. The similar characterized results were observed for other compounds 5b-5d. The results obtained from the NMR suggest that these synthesized compounds adopt a single cone conformation.
Initially, 11,23-bis-aminocalix[4]arene, benzaldehyde, benzil and ammonium acetate as model substrates to optimize the reaction conditions. In the acetic acid as the solvent, the above four component were stirred at room temperature for 48 h to afford the expected 11,23-bis(imidiazol-1-yl)calix[4]arene derivatives in a satisfactory yield (Table 1, entry 1). In order to fine-tune the reaction conditions, the effect of the temperature was first investigated. After researching the reaction in acetic acid at 50 °C, 80 °C and the refluxing temperature, the results were showed that higher yields were obtained from the reaction in refluxing acetic acid (Table 1, entries 2–4). At same time, we found that the reaction time was reduced to 6 h and the yield was increased to 89% when the same reaction was carried out at refluxing temperature (Table 1, entry 6), which means that the increasing of reaction temperature could accelerate the reaction rate.
Subsequently, we focused on the reaction solvent selection. Generally, solvent was critical and had a significant impact on the product yield. In the model reaction, the reaction could be going on smoothly in the presence of acetic acid as the solvent. When the acetic acid was replaced by ethanol or acetonitrile, there was an obvious decrease in the yield even though the reaction time was increased (Table 1, entries 7-10), which means that ethanol and acetonitrile are not effective solvents in this protocol because they could not afford a acid media for this reaction. In light of the acidity and solubility of acetic acid, we chose the ion liquid, 1-butyl-3-methylimidazolium ([Bmin]Br and [Bmin]HSO4), to replace the acetic acid and only 30% and 45% yields of product were obtained from the model reaction, respectively (Table 1, entries 11 and 12). Then pure water and HCl solution (15%) as solvents were tested as the reaction medium of model reaction (Table 1, entries 13 and 14). However, almost none product was detected. Therefore, acetic acid was used as the optimized solvent for further research.
Under the optimized conditions of this one-pot four-component, the role of benzaldehyde 3 with various substituent groups on the benzene ring was examined by the reaction with bisaminocalix[4]arene 1, benzil 2 and ammonium acetate 4 and it was found, except the o-salicylaldehyde, that the other benzaldehydes with electron-donation or electron-withdrawing gave the corresponding products 5 in reasonablely good to excellent yields within 6 h. Moreover, nitrobenzaldehyde expressed more efficiently in comparison to those bearing electron-donating groups and gave better yield and shorter reaction time (Table 2, entry 4). Especially, no product was observed for the reaction of o-salicylaldehyde in this one-pot four-component (Table 2, entry 5) due to the effect of the steric hindrance from hydroxyl group.
After completion of the reaction, the water was added into the reaction mixture with proper pH adjustment (about pH 7.0). Then the large quantities of solid were precipitated. After drying, the pure products were obtained by the recrystallization method with ethyl acetate avoiding tedious work-up and column purification. The products were characterized by 1H NMR, 13C NMR, IR, and elementary analysis.
In conclusion, we have described a one-pot four-component condensation reaction of 11,23-bisaminocalix[4]arenen, benzaldehyde, benzil and ammonium acetate in refluxing acetic acid to synthesize the 11,23-bis(imidazol-1-yl)calix[4]arene derivatives, which are all in a single cone conformation. The advantages of this protocol are catalyst-free reaction conditions, easy work-up, easy of product isolation and high yield. We believe that this method is a useful condensation reaction for synthesis of 11,23-bis(imidazol-1-yl)calix[4]arene derivatives on which the substituted imidazole rings are directly linked to the their upper rim.
EXPERIMENTAL
All melting points were estimated using a X4 melting apparatus apparatus in open capillaries and are uncorrected. IR spectra were determined as KBr disks with a Perkin-Elmer Spectrum one FT-IR. The 1H NMR spectra were recorded using a Bruker AV400MHz spectrometer using CDCl3 as solvent and TMS as an internal standard. The 13C NMR spectra were determined using TMS as an internal reference with a Bruker AV400MHz spectrometer operating at 100 MHz. Elemental analyses were performed on a Perkin-Elmer 240 microanalyser. Purity of compounds was checked by TLC. TLC was carried out on a Merck Kieselgel GF254. Column chromatography was performed using Merck Kieselgel 60 (0.075–0.15 mm). All of the organic solvents used in this study were dried over appropriate drying agents and distilled prior to use.
Starting Materials. Bi-aminocalix[4]arene (1),25 and benzil (2)26 were prepared by previously reported procedures. All other chemicals used in this study were commercially available.
Typical Procedure for the Preparation of Products 5a-5d. A mixture of bi-aminocalix[4]arene (1 mmol), appropriate aldehyde (2 mmol), benzil (2 mmol) and ammonium acetate (2 mmol) were magnetically stirred in 5 mL acetic acid at refluxing until the reactions were completed (monitored by TLC). After adding ice water, the solution was adjusted with the aqueous of NaOH until the pH value is about 7.0. The result precipitate was collected and recrystallized with CH2Cl2 and MeOH to give pure product for analysis.
5,17-Di-tert-butyl-11,23-bis(2,4,5-triphenylimidazol-1-yl)-25,27-dibutoxycalix[4]arene (5a): Gray white powder (yield 88%): mp >300 °C. IR (KBr) 3333, 3058, 2957, 2871, 1602, 1480, 1254, 1196, 1067 cm-1. 1H MNR (400 MHz, CDCl3), δ: 8.14 (s, 2H, OH), 7.58 (d, J = 8.4 Hz, 4H, ArH), 7.47 (d, J = 8.4 Hz, 4H), 7.19–7.28 (m, 22H, ArH), 6.91 (s, 4H, ArH), 6.55 (s, 4H, ArH), 4.18 (d, 4H, J = 13.2 Hz, ArH1H2Ar), 3.88 (t, J = 6.8Hz, OCH2), 3.17 (d, 4H, J = 13.2 Hz, ArH1H2Ar), 1.94–1.98 (m, 4H, CH2), 1.63–1.66 (m, 4H, CH2), 1.05 (t, 6H, J = 7.2 Hz, CH3), 0.87 (s, 18H, C(CH3)3); 13C MNR (100 MHz, CDCl3), δ: 153.8, 150.0, 147.6, 138.1, 134.7, 131.4, 131.1, 130.9, 128.9, 128.5, 128.3, 128.1, 128.0, 127.7, 126,5, 125.5, 76.2, 33.9, 32.2, 31.6, 31.4, 19.3, 14.1; Anal. Calcd for C86H84N4O4: C, 83.46; H, 6.84; N, 4.53. Found: C, 83.59; H, 6.71; N, 4.33.
5,17-Di-tert-butyl-11,23-bis[2-(4-chlorophenyl)-4,5-diphenylimidazol-1-yl]-25,27-dibutoxycalix[4]arene (5b): Gray white powder (yield 85%): mp >300 °C. IR (KBr) 3317, 3053, 2960, 2868, 1598, 1481, 1250, 1197, 1095, 1014 cm-1. 1H MNR (400 MHz, CDCl3), δ: 8.21 (s, 2H, OH), 7.55 (d, J = 7.2 Hz, 4H), 7.43 (d, J = 7.2 Hz, 4H), 7.19–7.28 (m, 20H, ArH), 6.93 (s, 4H, ArH), 6.57 (s, 4H, ArH), 4.16 (d, 4H, J = 13.2 Hz, ArH1H2Ar), 3.90 (t, 4H, J = 6.8 Hz, OCH2), 3.19 (d, 4H, J = 13.2 Hz, ArH1H2Ar), 1.93–2.01 (m, 4H, CH2), 1.63–1.68 (m, 4H, CH2), 1.06 (t, 6H, J = 7.2 Hz, CH3), 0.89 (s, 18H, C(CH3)3), 13C MNR (100 MHz, CDCl3), δ: 154.1, 150.2, 147.5, 138.1, 134.2, 131.3, 131.0, 129.1, 128.5, 128.3, 128.1, 127.5, 126.7, 125.5, 76.3, 33.9, 32.2, 31.6, 31.2, 19.4, 14.3; Anal. Calcd for C86H82Cl2N4O4: C, 79.06; H, 6.33; N, 4.29. Found: C, 79.11; H, 6.46; N, 4.18.
5,17-Di-tert-butyl-11,23-bis[2-(4-methoxyphenyl)-4,5-diphenylimidazol-1-yl]-25,27-dibutoxycalix[4]arene (5c): Gray white powder (yield 82%): mp >300 °C. IR (KBr) 3317, 3053, 2955, 2868, 1611, 1482, 1254, 1176, 1071, 1030 cm-1. 1H MNR (400 MHz, CDCl3), δ: 7.98 (s, 2H, OH), 7.56 (d, J = 7.2 Hz, 4H), 7.42 (d, J = 7.2 Hz, 4H), 7.19–7.34 (m, 16H, ArH), 6.92 (s, 4H, ArH), 6.70 (m, 4H, ArH), 6.55 (s, 4H, ArH), 4.18 (d, 4H, J = 13.2 Hz,ArH1H2Ar), 3.88 (t, 4H, J = 6.8 Hz, OCH2), 3.83 (s, 6H, CH3), 3.21 (d, 4H, J = 13.2 Hz, ArH1H2Ar), 1.92–1.99 (m, 4H, CH2), 1.58–1.67 (m, 4H, CH2), 1.05 (t, 6H, J = 7.2 Hz, CH3), 0.88 (s, 18H, C(CH3)3); 13C MNR (100 MHz, CDCl3), δ: 159.5, 153.8, 150.0, 147.5, 131.4, 131.0, 129.9, 128.9, 128.3, 128.1, 127.6, 126.5, 125.5, 113.7, 76.3, 55.1, 33.9, 32.2, 31.7, 31.3, 19.3, 14.1; Anal. Calcd for C88H88N4O6: C, 81.45; H, 6.84; N, 4.32. Found: C, 81.30; H, 6.79; N, 4.47.
5,17-Di-tert-butyl-11,23-bis[2-(4-nitrophenyl)-4,5-diphenylimidazol-1-yl]-25,27-dibutoxycalix[4]arene (5d): Gray white powder (yield 88%): mp >300 °C. IR (KBr) 3341, 3053, 2958, 2871, 1592, 1521, 1480, 1340, 1256, 1196, 1068, 1020 cm-1. 1H MNR (400 MHz, CDCl3), δ: 8.27 (d, J = 7.2 Hz, 4H), 7.91 (s, 2H, OH), 7.56 (d, J = 7.2 Hz, 4H), 7.19–7.36 (m, 20H, ArH), 6.96 (s, 4H, ArH), 6.57 (s, 4H, ArH), 4.16 (d, 4H, J = 13.2 Hz, ArH1H2Ar), 3.90 (t, 4H, J = 6.8 Hz, OCH2), 3.19 (d, 4H, J = 13.2 Hz, ArH1H2Ar), 1.93–2.01 (m, 4H,CH2), 1.63–1.68 (m, 4H, CH2), 1.06 (t, 6H, J = 7.2 Hz, CH3), 0.89 (s, 18H, C(CH3)3), 13C MNR (100 MHz, CDCl3), δ: 171.1, 154.5, 146.9, 136.8, 134.1, 131.3, 128.5, 128.3, 128.0, 127.5, 127.0, 123.5, 76.6, 33.8, 32.3, 31.7, 31.2, 19.4, 14.2; Anal. Calcd for C82H86N6O8: C, 77.80; H, 6.23; N, 6.33. Found: C, 77.67; H, 6.41; N, 6.52.
ACKNOWLEDGEMENTS
This work was supported by the Science Fund for Young Scholars of Heilongjiang Province of China (no. QC2009C61), the Program for Young Teachers’ Scientific Research at Qiqihar University (no. 2012K–Z09).
References
1. C. D. Gutche and R. J. Muthukrishnan, J. Org. Chem., 1978, 43, 4905. CrossRef
2. O Sénèque, M. N. Rager, G. Michel, and O. Reinaud, J. Am. Chem. Soc., 2000, 122, 6183. CrossRef
3. A. Arduinia, M. Cantonia, E. Graviania, A. Pochinia, A. Secchia, A. R. Sicuria, R. Ungaroa, and M. Vincentib, Tetrahedron, 1995, 51, 599. CrossRef
4. R. Ungaro, A. Arduini, A. Casnati, A. Pochini, and F. Ugozzoli, Pure Appl. Chem., 1996, 68, 1213. CrossRef
5. C. Capici, G. Gattuso, A. Notti, M. F. Parisi, S. Pappalardo, G. Brancatelli, and S. Geremia, J. Org. Chem., 2012, 77, 9668. CrossRef
6. H. M. Chawla, R. Shukla, and S. Pandey, Tetrahedron Lett., 2012, 53, 2996. CrossRef
7. K. Araki, H. Shimizu, and S. Shinkai, Chem. Lett., 1993, 22, 205. CrossRef
8. A. Ohki, S. Maeda, J. P. Lu, and R. A. Bartsch, Anal. Chem., 1994, 66, 1743. CrossRef
9. A. D. Martin, C. L. Raston, A. N. Sobolev, and M. A. Spackman, Cryst. Growth Des., 2009, 9, 3759. CrossRef
10. X. S. Zeng, L. H. Weng, L. X. Chen, X. B. Leng, Z. Z. Zhang, and X. W. He, Tetrahedron Lett., 2000, 41, 4917. CrossRef
11. J. Hong, S. Cho, and S. Ham, Tetrahedron Lett., 2012, 53, 2009. CrossRef
12. J. L. Sessler, D. E. Gross, W. S. Cho, V. M. Lynch, F. P. Schmidtchen, G. W. Bates, M. E. Light, and P. A. Gale, J. Am. Chem. Soc., 2006, 128, 12281. CrossRef
13. K. Chellappan, N. Singh, I. C. Hwang, J. W. Lee, and K. S. Kim, Angew. Chem. Int. Ed., 2005, 117, 2959. CrossRef
14. H. Yang, H. Song, Y. Zhu, and S. Yang, Tetrahedron Lett., 2012, 53, 2026. CrossRef
15. H. Miyaji, P. Anzenbacher Jr, J. L. Sessler, E. R. Bleasdale, and P. Gale, Chem. Commun., 1999, 1723. CrossRef
16. Y. Iv, J. Xu, Y. Guo, and S. Shao, J. Incl. Phenom. Macro., 2012, 72, 95. CrossRef
17. R. S. Varma, Green Chem. 1999, 1, 43. CrossRef
18. (a) C. Nitsche and C. D. Klein, Tetrahedron Lett., 2012, 53, 5197; CrossRef (b) S. Sarkar, S. Dutta, and A. K. Sen, Synthesis, 2012, 44, 1079; CrossRef (c) C. O. Kappe and D. Dallinger, Nat. Rew. Drug Discov., 2006, 5, 51. CrossRef
19. V. G. Nenajdenko and N. V. Sokolova, Chem. Heterocycl. Commun., 2012, 48, 903. CrossRef
20. (a) C. S. Yao, B. Jiang, S. J. Tu, G. Li, T. J. Li, H. M. Yin, and X. S. Wang, Chem. Commun., 2012, 48, 11966; CrossRef (b) J. B. Gianino and B. L. Ashfeld, J. Am. Chem. Soc., 2012, 134, 18217; CrossRef (c) J. G. Yu, H. L. Zhang, Y. M. Zhang, L. J. Geng, and G. L. Feng, Synth. Commun., 2011, 41, 3448; CrossRef (d) A. Alizadeh, N. Zohreh, H. Sabahnoo, and Z. Noaparast, Tetrahedron, 2011, 67, 1709. CrossRef
21. (a) G. Gattuso, C. Liotta, A. Notti, S. Pappalardo, and M. F. Parisi, Tetrahedron Lett., 2008, 49, 7146; CrossRef (b) H. M. Chawla, S. P. Singh, and S. Upreti, Tetrahedron, 2007, 63, 5636; CrossRef (c) M. Mocerino, M. I. Ogden, J. K. Pettersen, W. S. Brian, and A. H. White, Supramol. Chem., 2006, 18, 91. CrossRef
22. (a) S. K. Nayak and M. K. Choudhary, Tetrahedron Lett., 2012, 53, 141; CrossRef (b) Y. K. Agrawal and R. N. Patadia, Synth. Commun., 2006, 36, 1083; CrossRef (c) V. K. Jain, H. C. Mandalia, and E. Suresh, J. Incl. Phenom. Macro., 2008, 62,167. CrossRef
23. (a) J. Zhan, D. Tian, and H. Li, New J. Chem., 2009, 33, 725; CrossRef (b) M. J. Hardman, A. M. Thomas, L. T. Carroll, L. C. Williams, S. Parkin, and J. L. Fantini, Tetrahedron, 2011, 67, 7027; CrossRef (c) W. Liu, M. A. Minier, A. H. Franz, M. Curtis, and L. Xue, Supramol. Chem., 2011, 23, 806. CrossRef
24. (a) B. S. Creaven, T. L. Gernon, J. McGinley, A. M. Moore, and H. Toftlund, Tetrahedron, 2006, 62, 9066; CrossRef (b) C. C. Zeng, Q. Y. Zheng, Y. L. Tang, and Z. T. Huang, Tetrahedron, 2003, 59, 2539. CrossRef
25. B. Zhao, Y. Y. Ruan, Q. G. Deng, L. Y. Wang, B. Song, and Y. Q. Feng, Heterocycl. Commun., 2013, 19, 19. CrossRef
26. J. M. Khurana and R. Arora, ARKIVOC, 2008, xiv, 211. CrossRef