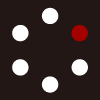
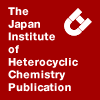
HETEROCYCLES
An International Journal for Reviews and Communications in Heterocyclic ChemistryWeb Edition ISSN: 1881-0942
Published online by The Japan Institute of Heterocyclic Chemistry
e-Journal
Full Text HTML
Received, 13th July, 2013, Accepted, 31st July, 2013, Published online, 7th August, 2013.
DOI: 10.3987/COM-13-12778
■ A CONVENIENT SYNTHESIS OF 2-MERCAPTO-OXAZOLES VIA β-KETOAZIDE AND ITS APPLICATION TO A KEY INTERMEDIATE OF PI3Kγ INHIBITORS
Yusuke Oka,* Tetsuya Yabuuchi, and Yoshinori Sekiguchi
Medicinal Chemistry Laboratories, Taisho Pharmaceutical Co., Ltd., 1-403 Yoshino-Cho, Kita-ku, Saitama-city, Saitama 331-9530, Japan
Abstract
A convenient synthesis of 2-mercapto-oxazoles via the reaction of β-ketoazide with triphenylphosphine (TPP) and carbon disulfide and its application to the synthesis of a key intermediate of phosphoinositide 3-kinase γ (PI3Kγ) inhibitors are described.INTRODUCTION
Oxazoles are an important chemical class of heterocycles in the pharmaceutical industry and in natural products chemistry.1 The biological activities of synthetic oxazoles and complex naturally occurring derivatives continue to drive organic chemists to develop new synthetic methods.2,3 Of the available synthetic approaches, iminophosphorane-mediated synthesis is pre-eminent because this method can provide several kinds of alkyl, aryl, and secondary amine groups at the 2-position of 1,3-oxazoles.4,5
For an extensive structure-activity relationship (SAR) study, however, the introduction of a greater variety of functional groups, such as tertiary amine, alkoxyl, and sulfonyl groups, into the 2-position of 1,3-oxazoles is needed, as we previously reported in a description of our phosphoinositide 3-kinase γ (PI3Kγ) program (Figure 1).6 2-Mercapto-oxazole is an attractive intermediate for the development of SAR studies because it can easily be converted into not only the corresponding thioether and alkyl sulfonyl groups, but also 2-chloro-oxazole, which can be subjected to an SNAr displacement reaction. In our previous paper, we used the reported method7 to prepare the desired compound 3 using α-aminoketone 2 with carbon disulfide (Figure 1). However, the instability of 2 under acidic conditions led to a low yield of intermediate 3, which made it difficult to apply this process to the multi-gram synthesis of 3. In addition, the generation of toxic H2S gas was of concern during the cyclization step using carbon disulfide.
Herein, we report an easy and modified synthesis method for 2-mercapto-oxazole derivatives through an iminophosphorane-mediated reaction and its application to the multi-gram scale preparation of a key intermediate for PI3Kγ inhibitors.
RESULTS AND DISCUSSION
The synthesis of 2-substituted oxazoles via iminophosphoranes derived from β-ketoazide has been reported.4 This methodology was applied to the synthesis of 2-mercapto-oxazole 3 via an iminophosphorane-mediated reaction with carbon disulfide (Table 1). The reaction of β-ketoazide 1 with carbon disulfide and triphenylphosphine (TPP) under the reported conditions (CHCl3, instead of CH2Cl2 or toluene)4b produced the desired 2-mercapto-oxazole 3 at low-isolated yields (yields of 31 % and 43 %, respectively). The use of MeCN in place of the solvents described above improved the conversion of 1 into 3. In addition, ether series solvents, such as THF and dioxane, were found to enhance this reaction (entries 4 and 5). When the reaction was carried out at 100 °C in dioxane, the yield was further improved up to 78 % (entry 6). Under this condition, the multi-gram synthesis (up to a scale of 10 g) of 2-mercapto-oxazole 3 was successfully achieved (entry 7, 63 % yield). These results prompted us to further investigate the substrate scope and limitations of this reaction under our optimized conditions (Table 2).
The iminophosphorane-mediated reaction of β-ketoazides 10 with carbon disulfide generally produced the corresponding oxazoles 11 at good yields, as shown in Table 2. Phenyl groups with an electron-donating substituent, such as a methoxy group or p-bromine atom, produced oxazoles at high yields (entries 2-5). Changing the position of the electron-donating substituents (o-, m-, and p-methoxy) gave products in moderate to good yields (entries 2-4). By contrast, an attempt to introduce an electron-withdrawing substituent (methyl ester group) into the p-position of the phenyl group resulted in a low yield. This feature was just as valid for π-electron-deficient heteroaromatic rings, such as a 2-pyridine analogue 10g (entries 6 and 7). When α-methyl β-ketoazide 10h and t-butyl β-ketoazide 10i were applied, the corresponding oxazoles were obtained at yields of 94 % and 86 %, respectively. These results suggest that this synthetic methodology tends to be susceptible to an electronic effect, rather than to a steric hindrance.
A plausible mechanism for the sequence of the reaction is shown in Scheme 1. First, the β-ketoazide reacted with TPP to yield an iminophosphorane (Staudinger reaction), which then underwent an aza-Wittig type reaction with the carbon disulfide to yield an isothiocyanate intermediate (III). The subsequent intramolecular cyclization of the carbonyl group (or the enol form) to the isothiocyanate moiety produced the 2-mercapto-oxazoles. The low yields, in the case of a phenyl ring with an electron-withdrawing group or an π-electron-deficient pyridine ring, may be due to the low electron density across the carbonyl group of intermediate III.8
In conclusion, we have developed an iminophosphorane-mediated one-pot synthesis method for producing 2-mercapto-oxazoles from β-ketoazide, carbon disulfide, and TPP. This method has been applied to prepare 2-mercapto-oxazole 3, which is a key intermediate of PI3Kγ inhibitors, and appears to be broadly applicable. The method described above enabled us not only to avoid the reduction step of the azide group and the generation of H2S gas, but also to synthesize multi-gram quantities of 2-mercapto-oxazoles easily and reproducibly. Because of the availability of the starting materials and the simplicity of this procedure, this synthetic approach may complement the existing methods for the synthesis of 2-mercapto-oxazoles.
EXPERIMENTAL
The 1H NMR spectra were determined using TMS as an internal reference with a JEOL ECA600 NMR spectrometer operating at 600 MHz. The 13C NMR spectra were determined using TMS as an internal reference with a JEOL ECA500 NMR spectrometer operating at 126 MHz or a JEOL ECA600 NMR spectrometer operating at 151 MHz. Low-resolution mass spectra (MS) were recorded using a Micromass Platform LC mass spectrometer with an electrospray ionization (ESI)/atmospheric pressure chemical ionization (APCI) dual source. High-resolution mass spectra (HRMS) were recorded using a Shimadzu LCMS-IT-TOF mass spectrometer with an ESI/APCI dual source. Elemental analyses were performed using a Perkin-Elmer 2400, a Yanaco MT-6, or an Elementar vario MICRO cube elemental analyzer, and the results were within 0.4 % of the calculated values.
Typical Procedure for the Preparation of 2-Mercapto-oxazoles
N-[4-Methyl-5-(2-sulfanyl-1,3-oxazol-5-yl)-1,3-thiazol-2-yl]acetamide (3): Carbon disulfide (5.7 mL, 94 mmol) was added to a solution of β-ketoazide 1 (15 g, 63 mmol) and PPh3 (25 g, 94 mmol) in dioxane (300 mL). After being stirred for 0.5 h at room temperature, the reaction mixture was stirred at 100 °C for 4 h. Then, 1 M NaOH (500 mL) was added to the mixture, and the mixture was washed with CHCl3. The water layer was acidified with AcOH and extracted using CHCl3-MeOH. The combined organic layer was washed with brine, dried over MgSO4, filtered and evaporated in vacuo, and washed with AcOEt to provide 3 (10 g, 63%): a pale yellow powder; 1H NMR (DMSO-d6, 600 MHz) δ 2.15 (s, 3H), 2.35 (s, 3H), 7.56 (s, 1H), 12.32 (s, 1H), 13.35 (br s, 1H); 13C NMR (DMSO-d6, 151 MHz) δ 16.40, 22.37, 109.87, 112.71, 141.20, 144.88, 156.26, 168.72, 176.84; MS m/z 256 (M+H)+, 278 (M+Na)+, 254 (M-H)-. Anal. Calcd for C9H9N3O2S2 ·0.1H2O: C, 42.04; H, 3.61; N, 16.34. Found: C, 41.76; H, 3.48; N, 16.11.
5-(2-Methoxyphenyl)-1,3-oxazole-2-thiol (11d): a pale yellow powder; 1H NMR (DMSO-d6, 600 MHz) δ 3.93 (s, 3 H) 7.07 (td, J = 7.64, 0.83 Hz, 1 H) 7.14 (d, J = 7.84 Hz, 1 H) 7.34-7.39 (m, 1 H) 7.55 (s, 1 H) 7.60 (dd, J = 7.84, 1.65 Hz, 1 H) 13.29 (br s, 1 H); 13C NMR (DMSO-d6, 151 MHz) δ 55.53, 111.38, 114.94, 115.08, 120.74, 124.32, 129.47, 143.88, 154.75, 176.53; MS m/z 208 (M+H)+, 230 (M+Na)+, 206 (M-H)-. Anal. Calcd for C10H9NO2S: C, 57.95; H, 4.38; N, 6.76. Found: C, 57.82; H, 4.34; N, 6.70.
Methyl 4-(2-sulfanyl-1,3-oxazol-5-yl)benzoate (11f): a pale yellow powder; 1H NMR (DMSO-d6, 600 MHz) δ 3.86 (s, 3H), 7.75 (d, J = 8.26 Hz, 2H), 8.03 (d, J = 8.26 Hz, 2H), 8.08 (s, 1H), 13.46 (br s, 1H); 13C NMR (DMSO-d6, 126 MHz) δ 52.20, 114.78, 123.18, 128.67, 128.77, 128.88, 129.95, 130.56, 146.15, 165.60, 177.67; MS m/z 236 (M+H)+, 258 (M+Na)+, 234 (M-H)-. HRMS m/z Calcd for C11H9NO3S (M+H)+, 236.0376. Found: 236.0371.
4-Methyl-5-phenyl-1,3-oxazole-2-thiol (11h): a pale yellow powder; 1H NMR (CDCl3, 600 MHz) δ 2.39 (s, 3H), 7.32-7.39 (m, 1H), 7.40-7.48 (m, 2H), 7.52-7.60 (m, 2H), 12.00 (br s, 1H); 13C NMR (CDCl3, 151 MHz) δ 9.56, 120.79, 125.11, 126.92, 128.59, 128.91, 144.34, 176.54; MS m/z 192 (M+H)+, 214 (M+Na)+, 190 (M-H)-. HRMS m/z Calcd for C10H9NOS: (M+H)+, 192.0478. Found: 192.0483.
References
1. (a) D. C. Palmer and E. C. Taylor, The Chemistry of Heterocyclic Compounds. Oxazoles: Synthesis, Reactions and Spectroscopy, Parts A&B; Wiley: Hoboken, NJ, 2004, Vol. 60; CrossRef (b) V. S. C. Yeh, Tetrahedron, 2004, 60, 11995. CrossRef
2. (a) C. Wan, J. Zhang, S. Wang, J. Fan, and Z. Wang, Org. Lett., 2010, 12, 2338; CrossRef (b) B. Shi, A. J. Blake, W. Lewis, I. B. Campbell, B. D. Judkins, and C. J. Moody, J. Org. Chem., 2010, 75, 152. CrossRef
3. (a) P. Wipf and J.-L. Methot, Org. Lett., 2001, 3, 1261; CrossRef (b) A. J. Philips, Y. Uto, P. Wipf, M. J. Reno, and D. R. Williams, Org. Lett., 2000, 2, 1165; CrossRef (c) P. Wipf and C. P. Miller, J. Org. Chem., 1993, 58, 3604. CrossRef
4. (a) P. Froyen, Phosphorous, Sulfur Silcon Relat. Elem., 1991, 60, 81; CrossRef (b) P. Molina, P. M. Fresneda, and P. Almendros, Synthesis, 1993, 54; CrossRef (c) P. Molina, P. M. Fresneda, and P. Almendros, Heterocycles, 1993, 36, 2255; CrossRef (d) H. Takeuchi, S. Yanagida, T. Ozaki, S. Hagiwara, and S. Eguchi, J. Org. Chem., 1989, 54, 431; CrossRef (e) A. Wolloch and E. Zbiral, Tetrahedron, 1976, 32, 1289; CrossRef (f) E. Zbiral, E. Bauer, and J. Stroh, Monatsh. Chem., 1971, 102, 168. CrossRef
5. (a) T. G. M. Dhar, Z. Shen, C. A. Fleener, K. A. Rouleau, J. C. Barrish, D. L. Hollenbaugh, and E. J. Iwanowicz, Bioorg. Med. Chem. Lett., 2002, 12, 3305; CrossRef (b) T. G. M. Dhar, Z. Shen, C. A. Fleener, K. A. Rouleau, J. C. Barrish, D. L. Hollenbaugh, and E. J. Iwanowicz, Org. Lett., 2002, 4, 2091; CrossRef (c) T. G. M. Dhar, Z. Shen, J. Guo, C. Liu, S. H. Watterson, H. H. Gu, W. J. Pitts, C. A. Fleener, K. A. Rouleau, N. Z. Sherbina, K. W. McIntyre, M. R. Witmer, J. A. Tredup, B.-C. Chen, R. Zhao, M. S. Bednarz, D. L. Cheney, J. F. MacMaster, L. M. Miller, K. K. Berry, T. W. Harper, J. C. Barrish, D. L. Hollenbaugh, and E. J. Iwanowicz, J. Med. Chem., 2002, 45, 2127. CrossRef
6. Y. Oka, T. Yabuuchi, Y. Fujii, H. Ohtake, S. Wakahara, K. Matsumoto, M. Endo, Y. Tamura, and Y. Sekiguchi, Bioorg. Med. Chem. Lett., 2012, 22, 7534. CrossRef
7. P. A. Harris, M. Cheung, R. N. Hunter III, M. L. Brown, J. M. Veal, R. T. Nolte, L. Wang, W. Liu, R. M. Crosby, J. H. Johnson, A. H. Epperly, R. Kumar, D. K. Luttrell, and J. A. Stafford, J. Med. Chem., 2005, 48, 1610. CrossRef
8. Similar explanation has been reported.5b