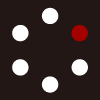
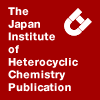
HETEROCYCLES
An International Journal for Reviews and Communications in Heterocyclic ChemistryWeb Edition ISSN: 1881-0942
Published online by The Japan Institute of Heterocyclic Chemistry
e-Journal
Full Text HTML
Received, 26th July, 2013, Accepted, 20th August, 2013, Published online, 10th September, 2013.
DOI: 10.3987/COM-13-12789
■ Facile Synthesis of Indolines by a Tandem Nitro-reduction Aza Michael Addition Reaction
Wellington Martins Ventura, Luiz Guilherme Souza de Assis, and Jason Guy Taylor*
Department of Chemistry, Federal University of Ouro Preto, Bauxita, ICEB, UFOP, Ouro Preto 35400-000, Brazil
Abstract
A diverse array of substrates are conveniently prepared by coupling diazonium salts to ethyl vinyl ether and subjecting the resultant aldehyde intermediate to a Wittig reaction to provide α,β-unsaturated esters with only one purification step. The cyclisation of 4-aryl-but-2-enoates is carried out in the presence of stoichiometric amounts of SnCl2∙2H2O and thus this one-pot strategy also permitted the expeditious synthesis of indolines in good yield.INTRODUCTION
Compounds with biological activity that have found applications as pharmaceuticals and agricultural chemicals are often derived from nitrogen-heterocyclic structures, which also appear frequently in natural products.1,2 Nitrogen-heterocycles pertinent to this work include indoline structures and a variety of synthetic methodologies for the synthesis of these structures have been summarized in many reviews.3,4
Despite the wide availability of synthetic methods, there still exists a need to develop more efficient procedures, particularly those that allow the synthesis of indoline intermediates with pendant functional groups which could later be exploited for the preparation of other complex azapolycyclic ring-systems. The intramolecular aza-Michael reaction offers a direct and atom-economical means of efficiently synthesizing nitrogen heterocycles. It is known that in the presence of triphenylphosphine, that azide functional group bearing acyclic α,β-unsaturated carbonyl and cyano compounds spontaneously cyclise upon reduction to afford pyrrolidines or piperidines in good yields.5 Furthermore, carbamates and sulfonamides can undergo intermolecular cyclisations with α,β-unsaturated carbonyl compounds in the presence of a simple base such as sodium tert-butoxide or sodium hydrogen carbonate6-10 (Scheme 1). In 2000 Bunce and co-workers demonstrated that the exploitation of a nitro group in a tandem reduction-intramolecular Michael addition protocol could efficiently provide nitrogen heterocycles such as 2-(tetrahydroquinolin-2-yl)-, 2-(3,4-dihydro-2H-benzo[b][1,4]oxazin-3-yl)- and 2-(tetrahydroquinoxalin-2-yl) acetates in good yield when simply employing iron powder in glacial acetic (Scheme 1).11
Thus, proof of concept had been established for the synthesis of aryl fused 6 membered ring nitrogen heterocycles and our curiosity was aroused as to whether analogous 5 membered compounds could be conveniently accessed in a similar manner. The availability of a convenient approach for the synthesis of the requisite precursor for the domino reaction would render this approach synthetically useful for its application in a longer multistep synthesis. In this regard, herein, we report the application of a domino nitro reduction-intramolecular aza-Michael strategy which occurs in a one-pot fashion upon when 4-(2-nitrophenyl)but-2-enoate derivatives are reduced in the presence of zinc powder to afford substituted indoline derivatives.
RESULTS AND DISCUSSION
The synthesis of the target precursor begins with diazotization of commercially available substituted 2-nitroanilines that are subsequently coupled to ethyl vinyl ether in the presence of Cp2Fe following the protocol described by Buchwald and Chernyak.12 The coupling reaction can be considered as the synthetic equivalent of an α-arylation with acetaldehyde enolate to provide substituted 2-(2-nitrophenyl)acetaldehyde derivatives.
Next, the crude reaction mixture containing 2-(2-nitrophenyl)acetaldehyde was employed directly in the synthesis of the α,β-unsaturated esters via a Wittig reaction with phosphonium ylide 2 under neutral conditions (Scheme 2).
Synthesis of the α,β-unsaturated carbonyl compound in which the neighboring methylene carbon is a also a benzylic position is often troublesome due to the possibility for double bond migration.13 A small amount of undesired isomer was isolated by column chromatography, but in general, the synthesis of 3a-g proceeded smoothly to afford the α,β-unsaturated esters in modest yields and with predominantly E-selectivity (>96%) as determined by 1H NMR spectroscopy.
Reduction of 3a under a hydrogen atmosphere in the presence of a catalytic amount of Pd/C gave substituted indole 4 in good yield.
The spectral data for 4 were identical with those reported for the compound that was obtained by using a different synthetic route.14 Evidently, the nitro group is reduced faster than the alkene function resulting in formation of a substituted aniline, which then undergoes an intramolecular Michael addition reaction to give a cyclic precursor to 4. The formation of indole 4 from the intermediate indoline requires a Pd-catalyzed dehydrogenation reaction to take place. The highly efficient Pd-catalyzed aromatization of indolines are known.15
Substrates 3a-g were subjected to the tandem reduction-aza-Michael reaction using SnCl2∙2H2O as the reducing agent (Table 1). Perhaps unsurprisingly, substituted indolines 5a-g were obtained in good yield. In the case of 3a, a small amount of a white solid was also isolated from the reaction mixture, which was found to be 2-methylindole. This was confirmed by the 1H NMR spectra displaying two clear singlet resonance at 2.48 ppm (CH3) and 6.26 ppm (CH), integrating in a 3 : 1 ratio, corresponding to the methyl and methine protons.16
We were pleased to find that in general, yields were modest to good irrespectively of the electronic or steric nature of the aryl substituent (entries 1-6). It appears that the intramolecular aza-Michael reaction occurs spontaneously post nitro reduction and therefore allowing the obtainment of the indoline product in a one-pot fashion. Cyclisation is easily confirmed by absence of the olefinic hydrogen signals and the appearance of a methine double doublet at ~3.30 ppm in the 1H NMR spectra. The purity of the compounds was confirmed by 1H, 13C NMR spectra and high resolution mass spectrometry.
In summary, we have developed a convenient methodology for the synthesis of a variety of indolines in 3 synthetic steps that require only two purifications by column chromatography. The key cyclisation step is realized in the form of a domino type reaction in which nitro reduction is immediately followed by intramolecular aza-Michael reaction to α,β-unsaturated esters. Work is ongoing in our group to expand this approach towards the development of new strategies for the synthesis of medium sized benzo-fused nitrogen heterocycles.
EXPERIMENTAL
General Method for the Synthesis of Indoline Derivatives
To a solution of the substrate 5a-g (2 mmol) in THF (10 mL) and distilled water (4.5 mL) was added SnCl2∙2H2O (8 mmol). The reaction mixture was heated under reflux for 6 h. The reaction was monitored by TLC analysis. After cooling to room temperature, the solution was poured onto ice and treated with a solution of 5% NaHCO3 in water (20 mL) until the pH was between 7-8. EtOAc (20 mL) was then added and the white precipitate was filtered off. The product was then extracted with EtOAc (3 x 20 mL). The combined organic layers were dried over Na2SO4, filtered and the solvent was removed under reduced pressure to leave the crude product, which was further purified by column chromatography.
Ethyl 2-(indolin-2-yl)acetate (5a): The product was obtained as a pale yellow oil; Rf = 0.5 (Hexanes/ EtOAc, 7:3); υmax (thin film, cm-1): 3351, 2942, 1623, 1456, 1310, 1254, 1262, 1157, 1044, 925, 865, 761; δH (400 MHz, CDCl3): 7.12 (1H, d, J = 7.6 Hz), 7.09 (1H, t, J = 7.6 Hz), 6.79 (1H, t, J = 7.6 Hz), 6.73 (1H, d, J = 7.6 Hz), 5.67 (1H, br s), 4.28-4.31 (1H, m), 4.22 (2H, q, J = 7.2 Hz), 3.26 (1H, dd, J = 8.4, 15.2 Hz), 2.77-2.68 (3H, m), 1.32 (3H, t, J = 7.2 Hz); δC (100 MHz, CDCl3): 172.1, 148.3, 128.8, 127.7, 124.8, 120.3, 110.9, 60.8, 56.0, 40.2, 35.7, 14.2; MS m/z (EI): calcd. for C12H15NO2 205.1103, found 205.1109.
Ethyl 2-(6-chloroindolin-2-yl)acetate (5b): The product was obtained as a pale yellow oil; Rf = 0.4 (Hexanes/EtOAc, 7:3); υmax (thin film, cm-1): 3354, 2942, 1624, 1456, 1316, 1268, 1152, 1043, 934; δH (400 MHz, CDCl3): 7.01 (1H, d, J = 7.6 Hz), 6.72 (1H, d, J = 7.6 Hz), 6.56 (1H, s), 5.82 (1H, br s, NH), 4.23-4.30 (1H, m), 4.21 (2H, q, J = 7.2 Hz), 3.27 (1H, dd, J = 8.5, 15.0 Hz), 2.76-2.68 (3H, m), 1.32 (3H, t, J = 7.2 Hz); δC (100 MHz, CDCl3): 172.4, 143.3, 133.5, 124.7, 122.8, 120.5, 110.3, 60.5, 56.2, 40.5, 35.9, 14.4; MS m/z (EI): calcd. for C12H14ClNO2 239.0713, found 239.0709.
Ethyl 2-(4-methylindolin-2-yl)acetate (5c): The product was obtained as a pale yellow oil; Rf = 0.47 (Hexanes/EtOAc, 7:3); υmax (KBr disc, cm-1): 3359, 2951, 1635, 1447, 1376, 1312, 1269, 1174, 1159, 1061, 1040; δH (400 MHz, CDCl3): 7.06-7.02 (2H, m), 6.58 (1H, s), 5.71 (1H, br s, NH), 4.23-4.30 (1H, m), 4.22 (2H, q, J = 7.2 Hz), 3.29 (1H, dd, J = 8.5, 15.0 Hz), 2.79-2.68 (3H, m), 2.25 (3H, s), 1.35 (3H, t, J = 7.2 Hz); δC (100 MHz, CDCl3): 170.9, 151.3, 135.2, 127.9, 122.6, 118.5, 112.0, 60.0, 55.5, 40.0, 34.5, 18.6, 14.5; MS m/z (EI): calcd. for C13H17NO2 219.1259, found 219.1252.
Ethyl 2-(6-methoxyindolin-2-yl)acetate (5d): The product was obtained as a pale yellow oil; Rf = 0.35 (Hexanes/EtOAc, 7:3); υmax (thin film, cm-1): 3335, 2942, 1628, 1476, 1311, 1275, 1172, 1169, 1108, 972; δH (400 MHz, CDCl3): 7.11 (1H, d, J = 7.6 Hz), 6.14 (1H, d, J = 7.6 Hz), 6.02 (1H, s), 5.51 (1H, br s, NH), 4.23-4.30 (1H, m), 4.22 (2H, q, J = 7.2 Hz), 3.72 (3H, s), 3.27 (1H, dd, J = 8.5, 15.0 Hz), 2.74-2.67 (3H, m), 1.33 (3H, t, J = 7.2 Hz); δC (100 MHz, CDCl3): 173.3, 153.7, 145.3, 124.1, 123.8, 124.5, 115.0, 61.5, 56.2, 55.2, 40.2, 35.8, 14.4; MS m/z (EI): calcd. for C13H17NO3 235.1208, found 235.1212.
Ethyl 2-(4,6-dichloroindolin-2-yl)acetate (5e): The product was obtained as a pale yellow oil; Rf = 0.4 (Hexanes/EtOAc, 7:3); υmax (thin film, cm-1): 3364, 2947, 1624, 1510, 1456, 1312, 1268, 1158, 1063, 967, 940; δH (400 MHz, CDCl3): 6.64 (1H, s), 6.32 (1H, s), 5.74 (1H, br s, NH), 4.21-4.30 (1H, m), 4.21 (2H, q, J = 7.2 Hz), 3.25 (1H, dd, J = 8.5, 15.0 Hz), 2.76-2.70 (3H, m), 1.31 (3H, t, J = 7.2 Hz); δC (100 MHz, CDCl3): 172.4, 150.1, 136.3, 132.5, 124.7, 122.8, 111.3, 60.1, 56.6, 40.2, 36.1, 14.5; MS m/z (EI): calcd. for C12H13Cl2NO2 273.0323, found 273.0317.
Ethyl 2-(6-bromoindolin-2-yl)acetate (5f): The product was obtained as a pale yellow oil; Rf = 0.4 (Hexanes/EtOAc, 7:3); υmax (thin film, cm-1): 1721, 1625, 1473, 1441, 1394, 1380, 1379, 1328, 1299, 1255, 1252, 1175, 1055, 1027, 904; δH (400 MHz, CDCl3): 7.03 (1H, d, J = 7.6 Hz), 6.70 (1H, d, J = 7.6 Hz), 6.59 (1H, s), 5.70 (1H, br s, NH), 4.21 (2H, q, J = 7.2 Hz), 3.27 (1H, dd, J = 8.5, 15.0 Hz), 2.76-2.68 (3H, m), 1.32 (3H, t, J = 7.2 Hz); δC (100 MHz, CDCl3): 172.1, 143.5, 133.2, 124.7, 122.5, 121.5, 113.5, 60.5, 56.1, 40.5, 35.8, 14.4; MS m/z (EI): calcd. for C12H14BrNO2 283.0208, found 283.0211.
Ethyl 2-(5,6-dimethylindolin-2-yl)acetate (5g): The product was obtained as a pale yellow oil; Rf = 0.37 (Hexanes/EtOAc, 7:3); υmax (thin film, cm-1): 3080, 2979, 2939, 2885, 2861, 1653, 1619, 1150, 1045; δH (400 MHz, CDCl3): 7.24 (1H, s), 6.90 (1H, s), 4.96 (1H, br s, NH), 4.25-4.29 (1H, m), 4.20 (2H, q, J = 7.2 Hz), 3.32 (1H, dd, J = 8.5, 15.0 Hz), 2.77-2.65 (3H, m), 2,22 (3H, s), 2.17 (3H, s), 1.32 (3H, t, J = 7.2 Hz); δC (100 MHz, CDCl3): 171.0, 137.3, 135.2, 131.9, 127.6, 126.5, 115.4, 60.3, 56.1, 40.0, 34.2, 20.2, 19.4, 14.4; MS m/z (EI): calcd. for C14H19NO2 233.1416, found 233.1420.
ACKNOWLEDGEMENTS
This work was supported by the Brazilian funding agency Fundação de Amparo à Pesquisa do Estado de Minas Gerais (FAPEMIG) under research grant project code APQ-00307-12. Authors gratefully acknowledge the generous financial support from, the Universidade Federal de Ouro Preto and the Conselho Nacional de Desenvolvimento Científico e Tecnológico (CNPq) for graduate research studentships and bursaries.
References
1. T. Naito, Chem. Pharm. Bull., 2008, 56, 1367. CrossRef
2. J. P. Michael, J. Nat. Prod. Rep., 1996, 13, 73. CrossRef
3. D. Liu, G. Zhao, and L. Xiang, Eur. J. Org. Chem., 2010, 3975. CrossRef
4. N. G. Kozlov, K. N. Gusak, and A. P. Kadutskii, Chem. Heterocycl. Compd., 2010, 46, 505. CrossRef
5. N. Knouzi, M. Vaultier, L. Toupet, and R. Carrie, Tetrahedron Lett., 1987, 28, 1757. CrossRef
6. S. Fustero, J. Moscardo, M. Sanchez-Rosello, E. Rodriguez, and P. Barrio, Org. Lett., 2010, 12, 5494. CrossRef
7. M. Gärtner, R. Weihofen, and G. Helmchen, Chem. Eur. J., 2011, 17, 7605. CrossRef
8. C. Enkisch and C. Schneider, Eur. J. Org. Chem., 2009, 5549. CrossRef
9. P. Wipf and Y. Kim, Tetrahedron Lett., 1992, 33, 5477. CrossRef
10. D. Bland, G. Chambournier, V. Dragan, and D. J. Hart, Tetrahedron, 1999, 55, 8953. CrossRef
11. R. A. Bunce, D. M. Herron, and M. L. Ackerman, J. Org. Chem., 2000, 65, 2847. CrossRef
12. N. Chernyak and S. L. Buchwald, J. Am. Chem. Soc., 2012, 134, 12466. CrossRef
13. N. D. F. Moreira, T. T. de Oliveira, T. J. Nagem, and J. G. Taylor, Heterocycl. Commun., 2011, 17 (5-6), 203. CrossRef
14. M. A. Guerrero and L. D. Miranda, Tetrahedron Lett., 2006, 47, 2517. CrossRef
15. R. Bader, R. J. Bridgwater, and P. R. Freeman, J. Am. Chem. Soc., 1961, 83, 3319. CrossRef
16. S. Cho, J. H. Kim, T.-J. Kim, and S. C. Shim, Tetrahedron, 2001, 57, 3321. CrossRef