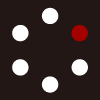
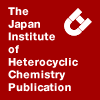
HETEROCYCLES
An International Journal for Reviews and Communications in Heterocyclic ChemistryWeb Edition ISSN: 1881-0942
Published online by The Japan Institute of Heterocyclic Chemistry
e-Journal
Full Text HTML
Received, 31st July, 2013, Accepted, 23rd October, 2013, Published online, 1st November, 2013.
DOI: 10.3987/COM-13-12795
■ DIOSPENTENOLS A AND B, TWO NEW CYCLOPENTAPYRANS ISOLATED FROM ABANDONED MINE BACTERIUM STREPTOMYCES SP. KMA-001
Jin-Soo Park, Jin Wook Cha, Seong-Hwan Kim, Dong-Chan Oh, and Hak Cheol Kwon*
Natural Medicine Center, Korea Institute of Science and Technology, 679 Saimdang-ro, 210-340, Korea
Abstract
Two new cyclopentapyrans, diospentenols A and B were isolated from the Streptomyces sp. KMA-001 which was isolated from a heat-treated soil sample collected at the abandoned zinc mine. Spectroscopic analyses including extensive 2D NMR spectra, mass spectrometry, and chemical modification revealed structures of diospentenols consisted of 7,7a-dihydro-2H- cyclepenta[b]pyran-6-one core with a hydroxyl group and allyl side chain. This carbon skeleton is rare in natural products reported in the literature. Diosphenol, previously isolated from marine sponge and ascidian, is one of a few known natural products that possess this structural core. Each diospentenol was verified as a racemate using the modified Mosher method and chiral HPLC analysis.INTRODUCTION
Abandoned mines are often extremely contaminated by heavy metals and acid, creating a unique bioregion. Microorganisms have adapted to life in the harsh conditions of an abandoned mine, leading hem to develop unique chemical arsenals.1, 2 The investigation of microbial diversity in the soil and drainage of abandoned mines led us to the isolation of several actinomycete strains including Streptomyces sp. KMA-001. The strain KMA-001 was isolated from soil collected from abandoned Yeonhwa zinc mine in Gangwon-do, Korea.
The initial chemical studies of secondary metabolites produced by the strain KMA-001 indicated that it predominantly produces aldgamycin derivatives.3 Further chemical analysis of this strain indicated the presence of two notable secondary metabolites that differ from the aldgamycin derivatives. These peaks were isolated and purified by flash column chromatography and prep-HPLC to yield two new cyclopentapyrans, diospentenols A (1) and B (2) (Figure 1).
This study describes the purification and analysis of these new compounds from the strain KMA-001 and discusses chemical modifications and circular dichroism spectral data for the assignment of their relative or absolute configuration.
RESULTS AND DISCUSSION
Diospentenol A (1) was obtained as a pale yellow amorphous powder. The molecular formula of 1 was established by HREIMS as C11H12O3 (obsd [M]+ at m/z 192.0785), indicating that 1 contains six degrees of unsaturation. The 13C NMR spectrum of 1 contains 11 carbon signals including one carbonyl carbon (δC 201.5), six olefinic carbons (δC 146.7, 136.4, 134.8, 134.2, 119.3 and 117.4), two oxygenated carbons (δC 76.4 and 67.7), one methine carbon (δC 51.8) and one aliphatic methylene carbon (δC 33.5). The molecular formula and 13C NMR data indicate the presence of two rings within 1. The 1H NMR spectrum contains 11 proton resonances including five olefinic protons (δH 6.77, 6.15, 5.82, 5.15 and 5.04), two hydroxylated methylene protons (δH 4.43 and 4.35), a carbinol proton (δH 4.21), two aliphatic methylene protons (δH 2.59 and 2.30) and a methine proton (δH 2.37). All protons were assigned with respect to their attached carbon atom via 1H-13C HSQC correlations. (Table 1) The 1H-1H COSY spectrum provided two partial structures, from H2-1 to H-3 and from H2-10 to H-11 (Figure 2).
Dihydro-2H-pyran as a partial structure of 1 was constructed by interpreting HMBC correlations from the carbinol proton H-11 (δH 4.21) to the oxygenated methylene carbon C-1 (δC 67.7) and the olefinic quaternary carbon C-4 (δC 134.8). The olefinic proton H-3 (δH 6.77) and the oxygenated methine proton H-11 (δH 4.21) were correlated to the olefinic quaternary carbon C-5 (δC 146.7) in the HMBC spectrum.
In addition, a methine proton H-7 (δH 2.37) was correlated to a ketone carbon C-6 (δC 201.5) in the HMBC spectrum. As a result, the linkage of an olefinic quaternary carbon C-5 (δC 146.7) and the ketone carbon C-6 (δC 201.5) was established to complete the second ring, thus describing the entire carbon framework. A hydroxyl group at C-5 was deduced based upon the chemical shift of C-5 and the molecular formula. The presence of this vinyl alcohol at C-5 was confirmed by acetylation using Ac2O in pyridine to produce 5-O-acetyldiospentenol A (3). The double bond geometry of C-2 and C-3 was assigned as a Z-configuration based on the 1H-1H coupling constant between H-2 and H-3 (10.0 Hz).4
The relative configurations at C-7 and C-11 were established through 1H-1H coupling constant analysis and 1D NOE experiments. As demonstrated in previous studies, vicinal coupling constants for two anti protons and those of two syn protons in cyclopentenone systems are approximately 2.5 and 6.5 Hz, respectively.5-7 The vicinal coupling constant (2.5 Hz) between H-11 and H-7 and no NOE enhancement of H-7 by irradiation of H-11 thus indicates an anti-relationship between H-7 and H-11 in compound 1 (Figure 3).
To determine the absolute configuration at C-7 and C-11 of 1, it was necessary to modify the ketone at C-6 of 1 to a hydroxyl group for the modified Mosher’s method. Before reduction of the ketone, the hydroxyl group at C-5 was acetylated, and the acetylated derivative of 1 (3) was reduced using sodium borohydride in methanol as shown Scheme 1. Contrary to our expectations, deacetylation and reduction of the double bond between C-4 and C-5 occurred to yield 4,5-dihydro-6-hydroxydiospentenol A (4). The direct treatment of 1 with sodium borohydride in methanol also resulted in the formation of compound 4. As an alternative, we protected the C-5 hydroxyl group by methylation to yield 5-O-methyldiospentenol A (5).8 The treatment of 5 with sodium borohydride in methanol afforded 5-O-methyl-6-hydroxydiospentenol A (6).
The relative configurations of compounds 4 and 6 were assigned by the interpretation of NOESY NMR spectra (Figure 4). In the 2D NOESY spectrum of 4, proton H-4 shows NOE correlations with H-5 and H-6, whereas H-5 shows NOE correlations with H-4 and H-11. These NOE data indicate syn-relationships between H-4, H-5, H-6 and H-11. NOE correlations were observed between H-6, H2-8 and H-11 in the 2D NOESY spectrum of 6 (Figure 4). These NOE data, coupled with the relative configuration in compound 4, confirmed the relative configurations at C-6, C-7 and C-11 of 6. On the basis of these data, the relative configuration at C-7 and C-11 of diospentenol A (1) was assigned as anti-configuration.
To determine the absolute configurations at C-6, C-7 and C-11 of 6, compound 6 was treated with dimethylaminopyridine and (R)-(-)-α-methoxy-α-(trifluoromethyl)phenylacetyl chloride ((R)-MTPA-Cl) in pyridine-d5 in an NMR sample tube. Unexpectedly, even though the CD spectra of compounds 1 and 2 showed distinguishable Cotton effects at 310-360 nm (Figure S25), the 1H NMR spectrum of the (S)-Mosher ester of 6 contains two sets of proton signals, attributable to the presence of a diastereomer mixture. This result reveals that 6 is a racemate, suggesting that diospentenol A (1) is also a racemate. However, it does not seem to be a 1:1 racemic mixture due to the strong Cotton effects in the CD spectrum. We examined the chiral purity of 1 using an HPLC-CD detector with a lux cellulose-1 chiral column (Phenomenex). The chiral HPLC-CD chromatogram clearly showed two peaks with opposite sign in an approximately 1:0.9 ratio. Diospentenol A (1) is thus racemate, and has an anti-configuration at C-7 and C-8.
Diospentenol B (2) was obtained as a pale yellow amorphous powder. The molecular formula of 2, established as C11H12O3 by HREIMS on the basis of a molecular ion peak at m/z 192.0783, is identical to that of 1. Its similar 1H and 13C NMR spectra and molecular formula suggested that 2 is a stereoisomer of 1. The vicinal coupling constant between H-7 and H-11 (6.5 Hz for 2 and 2.5 Hz for 1) as seen in the 1H NMR spectrum of 2 indicates a syn-relationship between H-7 and H-11 in 2.9 In addition, a significant NOE correlation between H-7 and H-11 in the 1D NOESY spectrum of 2 supports the relative configuration at C-7 and C-11 as a syn-configuration (Figure 2). The difference of 3JH-7,H-11 values of 1 and 2 seems to result from an eclipsed conformation between C-7 and C-11 in 1 and 2, not a staggered conformation as depicted Figure 3.10 The treatment of 2 in CD3OD with sodium borohydride in an NMR sample tube resulted in the formation of 4-deutero-5-hydro-6-hydroxydiospentenol B (7). In the 2D NOESY spectrum of 7, proton H-7 showed strong NOE correlations with H-5, H-6 and H-11. These NOE data demonstrate that H-5, H-6, H-7, and H-11 all have a syn relationship in compound 7. These results further confirmed the syn-configuration at C-7 and C-11 of diospentenol B (2). As in compound 1, chiral HPLC analysis revealed that diospentenol B (2) is also a racemate with 1:0.9 ratio. However, the CD spectrum of 2 contains a strong negative band at 310-360 nm (Figure S25). Absolute configuration for diospentenols A (1) and B (2) was difficult to determine due to difficulties in crystallization and the absence of hydroxyl functional groups at chiral centers or adjacent carbons at chiral centers. We tried to overcome these obstacles by reducing the ketone at C-6 to a hydroxyl group. However, due to the racemic mixtures of 1 and 2, the absolute configurations could not be determined. We did, however, succeed in forming a desirable stereoselective reduction product and its MTPA ester. At first, 1 and 2 were thought to be chirally pure based on CD spectra and optical rotations. The CD spectrum of 1 exhibited a negative Cotton effect at near 290 nm and a broad positive band between 310-380 nm, whereas 2 showed negative signs at near 290 nm and positive signs at 330-380 nm. Furthermore, the optical rotations of 1 and 2 were +22.5 and -12.5, respectively. Consequently, based on chiral HPLC-CD using a lux cellulose-1 chiral column (150 x 4.6 mm, Phenomenex), we knew that these CD and optical rotation data were caused by an approximately 1:0.9 racemic mixtures of both 1 and 2.
We attempted to resolve an absolute configuration of a stereo-isomer corresponding to each peak on the chiral HPLC-CD chromatogram of 1 using electronic circular dichroism (ECD) calculations by time-dependent density functional theory profile of 1.11 The pattern of Cotton effects in the CD spectrum of 1 were similar to the predictive spectrum of the (7S,11S) isomer by ECD calculation (Figure 5), thus allowing us to assign higher ratio stereo-isomer as (7S,11S)-configuration. The Cotton effects in the CD spectrum of 2 were also matched well with the ECD predictive spectrum for a (7R,11S) isomer (Figure 6). These results suggest that the compound 1 is a racemic mixture of (7S,11S) and (7R,11R) isomers with 1:0.9 ratio, whereas 2 is a 1:0.9 racemic mixture of (7R,11S) and (7S,11R) isomers.
Cyclopentenone derivatives have been isolated from various biological sources including bacteria,12-14 fungi7,15-17 and liverwort.18,19 These compounds show a wide range of biological activities, such as tyrosinase inhibition,14 antibacterial activities,19 leishmanicidal,20 glucose-6-phosphated translocase T1 inhibition17 and melanogenesis inhibitory activities.15 However, a cyclopenta[b]pyran is not explored thoroughly in the literature.21 Especially, a carbon skeleton of diospentenol incorporating a 2,3-dihydropyran and a cyclopent-2-enone is rare in the natural products reported in the literature. One of a few cyclopent[b]pyrans with a 2,3-dihydropyran and a cyclopent-2-enone derived from natural resources is diosphenol, isolated from marine invertebrates such as sponge and ascidians, which has a C7-C8 double bond, unlike 1 and 2.22-24 The diosphenol was also detected from the liquid culture medium of the strain KMA-001. In many cases, microorganisms are suspected to be the biosynthetic origins of marine invertebrate natural products.25 These assumptions are more plausible due to the detection of diosphenol in long-period cultures of strain KMA-001 (Figure S27).
The diosphenol showed cytotoxicity against HCT116 cells and antimicrobial effects on S. aureus, B. subtilis and C. albicans.22, 24 However, diospentenols A (1) and B (2) were inactive in a nitric oxide inhibitory assay using lipopolysaccharide (LPS)-induced murine macrophage RAW 267.7 cells and showed no cytotoxicity against AGS, A549, HCT116, HepG2, or MCF-7 cancer cells (IC50, over 40 µg g/mL). In addition, these compounds of 100 g/mL showed no antibacterial activity against Bacillus subtilis ATCC 6633 and Salmonella typhimurium ATCC 14028.
EXPERIMENTAL
IR spectra were recorded using a Thermo Scientific Nicolet iS10 spectrometer. 1H, 13C and 2D NMR spectral data were obtained in methanol-d4 or pyridine-d5 on a Varian Unity 500 MHz NMR spectrometer. Low resolution ESIMS were measured on a Waters Alliance 2695/Quattro micro API system. High-resolution mass spectral data were acquired on a JEOL/JMS-AX505WA instrument. A Lichroprep RP-18 column (Merck, 40-63 μm) was used for column chromatography. Semi-preparative HPLC separations were performed using a Gilson 321 HPLC system with a Phenomenex Luna C18 (2) 10 μm column (10 × 250 mm) at a flow rate 4 mL/min. A Waters 1525 HPLC-PDA system with a Phenomenex Luna C18 (2) 5 μm column (4.6 × 100 mm) was used for the analysis of extracts and fractions.
Biological Material. Strain KMA-001 was isolated from a heat-treated soil sample (55 °C, 5 min) collected at the Yeonhwa abandoned zinc mine in Korea. Yeast-malt extract (YM) agar containing nystatin (50 g/mL) was used for isolation of this strain. Close to full length 16S rDNA sequence analysis of strain KMA-001 indicated that it is Streptomyces sp. The 16S rDNA sequence of KMA-001 showed 99.3% homology with Streptomyces goshikiensis NBRC 12868, S. citricolor NBRC 13005 and S. sproverrucosus NBRC 15458.3 The GenBank accession number for the 16S rDNA sequence of strain KMA-001 is HM805015. This strain was deposited at the Korean Culture Center of Microorganisms (KCCM) under accession No. KCCM 42921.
Fermentation and Extraction. A stock culture of strain KMA-001 was maintained on yeast-malt extract (YM) agar containing nystatin (50 g/mL) to minimize fungal contamination. The YM agar medium consisted of yeast extract (Difco, 0.5%), malt extract (Difco, 3%) and agar (1.5%). The strain KMA-001 was grown on the YM agar medium for seven days and was then cultured in 25 mL YM liquid medium (yeast extract 4 g and malt extract 10 g in one liter of deionized water) in a 100 mL Erlenmeyer flask for three days at 28 °C with shaking at 200 rpm. This seed culture was inoculated into the production medium consisting of starch (10 g), yeast extract (4 g), peptone (2 g), FeSO4·7H2O (0.08 g), and KBr (0.1 g) in one liter of deionized water. The production culture (12 L) was incubated at 28 °C for 10 days on a rotary shaker (200 rpm). After 10 days of fermentation, 20 g/L Amberlite XAD-7 adsorbent resin was added to the culture flasks, and the mixture was shaken for an additional hour. The resin was then collected by filtration through cheesecloth, washed with deionized water, and eluted twice with acetone.
Isolation and Purification of 1 and 2. The acetone extract was fractionated by C18 flash column chromatography using mixtures of MeOH - water (20, 40, 60, 80 and 100% MeOH in water, each 200 mL, 20 mL/min) as elution solvents. The 40% MeOH fraction (200 mg) was separated by RP HPLC (Luna C-8(2) 21.2 × 250 mm, 15 μm) using a gradient elution from 20% MeCN in water up to 40% MeCN in water (flow rate 10 mL/min) for 60 min to afford two subfractions containing compounds 1 and 2. Each subfraction was subjected to prep-HPLC using a Luna C18(2) column (21.2 × 250 mm) and eluting with 29% MeCN in water (4 mL/min) to yield diospentenols A (1, 5 mg) and B (2, 3 mg).
Diospentenol A (1): pale yellowish amorphous powder; UV (MeOH) λmax (log ε) 296 (4.40) nm; IR (KBr) νmax 3315, 1702, 1420, 1080, 617 cm-1; 1H NMR (CD3OD, 500 MHz) and 13C NMR (CD3OD, 125 MHz) data, see Table 1; ESIMS m/z 193 [M+H]+; HREIMS m/z 192.0785 (calcd for C11H12O3, 192.0786).
Diospentenol B (2): pale yellowish amorphous powder; UV (MeOH) λmax (log ε) 296 (4.33) nm; IR (KBr) νmax 3449, 1686, 1422, 1026, 615 cm-1; 1H NMR (CD3OD, 500 MHz) and 13C NMR (CD3OD, 125 MHz) data, see Table 1; ESIMS m/z 193 [M+H]+; HREIMS m/z 192.0783 (calcd for C11H12O3, 192.0786).
Acetylation of 1 to Yield 5-O-Acetxyldiospentenol A (3). Acetic anhydride (100 μL) was added to a solution of compound 1 (0.3 mg) in dry pyridine (0.3 mL) and the reaction mixture was stirred at room temperature for 2 h. After completion of the reaction, the solvent was removed in vacuo and the residue was fractionated with EtOAc and water. The organic layer was concentrated under reduced pressure and then purified by RP-HPLC (Phenomenex Luna C-8(2), 250 × 10 mm) using a gradient elution from 10% MeCN in water to 100% MeCN for 30 min to afford 5-O-acetyldiospentenol (3) (0.3 mg).
5-O-Acetyldiospentenol A (3): yellowish gum; 1H NMR (CD3CN, 500 MHz) δ 6.68 (1H, ddd, J = 10.0, 2.5, 2.0 Hz, H-3), 6.52 (1H, ddd, J = 10.0, 4.0, 2.5 Hz, H-2), 5.87 (1H, ddt, J = 17.0, 10.0, 7.0 Hz, H-9), 5.22 (1H, ddt, J = 17.0, 2.0, 1.5 Hz, H2-10trans), 5.18 (1H, ddt, J = 10.0, 2.0, 1.0 Hz, H2-10cis), 4.50 (1H, dt, J = 19.0, 2.5 Hz, H2-1a), 4.45 (1H, ddd, J = 19.0, 4.0, 2.0 Hz, H2-1b), 4.38 (1H, br d, J = 3.0 Hz, H-11), 2.61 (1H, m, H2-8a), 2.55 (1H, m, H-7), 2.34 (1H, m, H2-8b), 2.26 (3H, br s, OCOCH3); ESIMS m/z 257 [M+Na]+.
Reduction of 1 and 3 to Yield 4,5-Dihydro-6-hydroxydiospentenol A (4). Compound 1 (0.5 mg) was dissolved in 1 mL of CD3OD, and excess NaBH4 was added. The reaction mixture was stirred at room temperature for 1 h. After quenching the reaction and subsequent concentration, the reaction residue was purified by using same method as 3 to afford 4,5-dihydro-6-hydroxydiospentenol A (4) (0.2 mg). In the same fashion, a solution of 3 (0.3 mg) in 1 mL MeOH was treated with excess sodium borohydride to afford, after the purification as mentioned above, compound 4 (0.1 mg).
4,5-Dihydro-6-hydroxydiospentenol A (4): yellowish gum; 1H NMR (CD3OD, 500 MHz) δ 6.00 (1H, ddd, J = 10.0, 4.0, 2.0 Hz, H-2), 5.90 (1H, m, H-9), 5.82 (1H, dt, J = 10.0, 2.0 Hz, H-3), 5.13 (1H, dt, J = 17.0, 1.5 Hz, H2-10trans), 5.02 (1H, dt, J = 10.0, 1.5 Hz, H2-10cis), 4.05 (1H, ddd, J = 16.0, 4.0, 2.0 Hz, H2-1a). 3.99 (1H, dt, J = 16.0, 2.0 Hz, H2-1b), 3.91 (1H, td, J = 4.0, 1.0 Hz, H-5), 3.69 (1H, ddd, J = 6.0, 3.5, 1.0 Hz, H-11), 3.64 (1H, dd, J = 9.0, 4.0 Hz, H-6), 2.45 (1H, dddt, J = 14.0, 6.5, 5.0, 1.5 Hz, H2-8a), 2.42 (1H, m, H-4), 2.17 (1H, dddt, J = 14.0, 9.0, 7.5, 1.5 Hz, H2-8b), 2.07 (1H, ddd, J = 9.0, 5.0, 3.5 Hz, H-7); ESIMS m/z 219 [M+Na]+.
Methylation of 1 to Produce 5-O-Methyldiospentenol A (5). Methyl iodide (20 μL) was added to a solution of compound 1 (0.7 mg) and potassium carbonate (1.3 mg) in anhydrous DMF (1 mL). The reaction mixture was stirred at room temperature for 2 h. After quenching with 1 M HCl at 0 °C, the solvent was removed in vacuo and the residue was extracted with EtOAc. The reaction residue was purified in the same manner as in the purification of 3 to afford 5-O-methyldiospentenol (5, 0.5 mg).
5-O-Methyldiospentenol A (5): yellowish gum; 1H NMR (CD3OD, 500 MHz) δ 6.94 (1H, br d, J = 10.0 Hz, H-3), 6.26 (1H, br d, J = 10.0 Hz, H-2), 5.82 (1H, ddt, J = 17.0, 10.0, 7.0 Hz, H-9), 5.15 (1H, br d, J = 17.0 Hz, H2-10trans), 5.05 (1H, br d, J = 10.0 Hz, H2-10cis), 4.45 (1H, dt, J = 18.0, 2.5 Hz, H2-1a), 4.38 (1H, dd, J = 18.0, 4.0 Hz, H2-1b), 4.22 (1H, br d, J = 3.0 Hz, H-11), 3.99 (3H, br s, OCH3), 2.58 (1H, m, H2-8a), 2.43 (1H, m, H-7), 2.31 (1H, m, H2-8b); ESIMS m/z 229 [M+Na]+.
Reduction of 5 to Yield 6-Hydroxy-5-O-methyldiospentenol A (6). Compound 5 (0.5 mg) in MeOH was treated with excess sodium borohydride, and the reaction mixture was stirred at room temperature for 1 h. After quenching and concentration, the reaction residue was purified in the same manner as in the purification of 3 to afford 6-hydoxy-5-O-methyldiospentenol (6, 0.3 mg).
6-Hydroxy-5-O-Methyldiospentenol A (6): 1H NMR (CD3OD, 500 MHz) δ 6.67 (1H, dt, J = 10.0, 2.0 Hz, H-3), 5.94 (1H, ddt, J = 17.0, 10.0, 7.0 Hz, H-9), 5.69 (1H, ddd, J = 10.0, 4.0, 2.0 Hz, H-2), 5.12 (1H, ddt, J = 17.0, 3.5, 1.0 Hz, H-10trans), 5.02 (1H, ddt, J = 10.0, 2.0, 1.0 Hz, H-10cis), 4.43 (1H, dt, J = 17.5, 2.0 Hz, H2-1a), 4.26 (1H, br d, J = 6.5 Hz, H-6), 4.23 (1H, ddd, J = 17.5, 4.0, 2.0 Hz, H2-1b), 3.91 (1H, br d, J = 6.5 Hz, H-11), 3.90 (3H, br s, OCH3), 2.47 (1H, br dt, J = 14.0, 7.0 Hz, H2-8a), 2.39 (1H, br dt, J = 14.0, 7.0 Hz, H2-8b), 1.90 (1H, tt, J = 7.0, 6.5 Hz, H-7); ESIMS m/z 231 [M+Na]+.
Reduction of 2 to Yield 4-Deutero-5-hydro-6-hydroxydiospentenol B (7). Compound 2 (0.3 mg) was dissolved in 1 mL of CD3OD in a 5 mm NMR sample tube. An excess of sodium borohydride was added to the NMR tube. The formation of compound 7 was detected by collecting 1H NMR spectrum of the reaction mixture. After 1 h, the reaction was complete, and compound 7 was purified by JAI recycling HPLC with an RI detector using a JAI GS-150 column (20 x 500 mm) and eluting with MeOH.
4-Deutero-5-hydro-6-hydroxydiopentenol B (7): yellowish gum; 1H NMR (CD3OD, 500 MHz) δ 5.92-5.93 (2H, H-3, H-9), 5.85 (1H, br d, J = 10.0 Hz, H-2), 5.11 (1H, br d, J = 17.0 Hz, H-10trans), 5.00 (1H, br d, J = 10.0 Hz, H-10cis), 4.15 (1H, br d, J = 5.5 Hz, H-5), 4.12 (1H, br dd, J = 16.0, 4.0 Hz, H2-1a), 3.98 (1H, t, J = 5.5 Hz, H-6), 3.93 (1H, br d, J = 16.0 Hz, H2-1b), 3.90 (1H, br d, J = 3.5 Hz, H-11), 2.42 (2H, t, J = 7.5 Hz, H2-8), 1.87 (1H, tdd, J = 7.5, 5.5, 3.5 Hz, H-7); 13C NMR (CD3OD, 500 MHz); δ 137.0, 127.1, 123.0, 114.6, 78.7, 75.2, 73.1, 64.0, 48.2, 45.8, 27.7.
Preparation of Mosher MTPA Esters from 6. 5-O-Methyl-6-hydroxydiospentenol (6) was divided into two portions; each was dissolved in 600 μL of pyridine-d5 in a 5 mm NMR sample tube, and a catalytic amount of dimethylaminopyridine was then added. To each NMR tube was added either 5 μL of (R)-MTPA-Cl or 5 μL of (S)-MTPA-Cl. After 12 h, the reaction was complete and 1H NMR spectra for the (S)-MTPA ester and (R)-MTPA ester were collected (Figure S24).
Computational Chemistry for ECD Calculation. The ground-state geometries were optimized with density functional theory (DFT) calculations, using Turbomole at the basis set def-SV(P) for all atoms and functional B3LYP/DFT level, the ground states were further confirmed by the harmonic frequency calculation. The calculated ECD data corresponding to the optimized structures were obtained with TDDFT at the functional B3LYP/DFT level and the basis set TZVPP. The CD spectra were simulated by overlapping for each transition, where σ is the width of the band at 1/e height. ΔEi and Ri are the excitation energies and rotatory strengths for transition i, respectively. In the current work a value of σ was 0.10 eV, the excitation number was 15.
ACKNOWLEDGEMENTS
This study was supported by the Global Leading Technology Program of the Office of Strategic R&D Planning (10039303) funded by the Ministry of Knowledge Economy, Republic of Korea, and the Korea Institute of Science and Technology institutional program, grant number 2Z03840. We thank the Center for Scientific Instruments at Gangneung-Wonju National University for use of the HPLC/MS.
References
1. G. Haferburg and E. Kothe, J. Basic Microbiol., 2007, 47, 453. CrossRef
2. D. B. Stierle, A. A. Stierle, J. D. Hobbs, J. Stokken, and J. Clardy, Org. Lett., 2004, 6, 1049. CrossRef
3. J. S. Park, H. O. Yang, and H. C. Kwon, J. Antibiot., 2009, 62, 171. CrossRef
4. H. Günther, NMR Spectroscopy: Basic Principles, Concepts, and Applications in Chemistry, 2nd ed., John Wiley & Sons, Chichester, 1996.
5. C. H. Depuy, C. E. Lyons, and L. B. Rodewald, J. Chem. Eng. Data, 1966, 11, 102. CrossRef
6. B. Dugovic and H. U. Reissig, Synlett, 2008, 769. CrossRef
7. X. F. Li, D. H. Zhang, U. Lee, X. G. Li, J. G. Cheng, W. L. Zhu, J. H. Jung, H. D. Choi, and B. W. Son, J. Nat. Prod., 2007, 70, 307. CrossRef
8. T. Doi, Y. Iijima, M. Takasaki, and T. Takahashi, J. Org. Chem., 2007, 72, 3667. CrossRef
9. R. Feling, K. Polborn, W. Steglich, J. Muhlbacher, and G. Bringmann, Tetrahedron, 2001, 57, 10231. CrossRef
10. M. Zora, T. A. Tumay, and O. Buyukgungor, Tetrahedron, 2007, 63, 4018. CrossRef
11. X. C. Li, D. Ferreira, and Y. Ding, Curr. Org. Chem., 2010, 14, 1678. CrossRef
12. W. H. Lin, L. Y. Li, H. Z. Fu, I. Sattler, X. S. Huang, and S. Grabley, J. Antibiot., 2005, 58, 594. CrossRef
13. J. Piel, Nat. Prod. Rep., 2004, 21, 519. CrossRef
14. Y. Q. Tang, I. Sattler, R. Thiericke, S. Grabley, and X. Z. Feng, Eur. J. Org. Chem., 2000, 2401. CrossRef
15. S. Lee, W. G. Kim, E. Kim, I. J. Ryoo, H. K. Lee, J. N. Kim, S. H. Jung, and I. D. Yoo, Bioorg. Med. Chem. Lett., 2005, 15, 471. CrossRef
16. X. F. Li, M. K. Kim, U. Lee, S. K. Kim, J. S. Kang, H. D. Choi, and B. W. Son, Chem. Pharm. Bull., 2005, 53, 453. CrossRef
17. L. Vertesy, H. J. Burger, J. Kenja, M. Knauf, H. Kogler, E. F. Paulus, N. V. S. Ramakrishna, K. H. S. Swamy, E. K. S. Vijayakumar, and P. Hammann, J. Antibiot., 2000, 53, 677. CrossRef
18. A. J. Barlow, B. J. Compton, U. Hertewich, S. D. Lorimer, and R. T. Weavers, J. Nat. Prod., 2005, 68, 825. CrossRef
19. G. B. Mitre, N. Kamiya, A. Bardon, and Y. Asakawa, J. Nat. Prod., 2004, 67, 31. CrossRef
20. M. Takahashi, H. Fuchino, S. Sekita, and M. Satake, Phytother. Res., 2004, 18, 573. CrossRef
21. C. Yao, B. Jiang, T. Li, B. Qin, X. Feng, H. Zhang, C. Wang, and S. Tu, Bioorg. Med. Chem. Lett., 2011, 21, 599. CrossRef
22. T. Ogi, J. Taira, P. Margiastuti, and K. Ueda, Molecules, 2008, 13, 595. CrossRef
23. B. N. Su, E. J. Park, Z. H. Mbwambo, B. D. Santarsiero, A. D. Mesecar, H. H. Fong, J. M. Pezzuto, and A. D. Kinghorn, J. Nat. Prod., 2002, 65, 1278. CrossRef
24. S. J. Wratten and D. J. Faulkner, Tetrahedron Lett., 1978, 961. CrossRef
25. A. G. Guzii, T. N. Makar'eva, V. A. Denisenko, P. S. Dmitrenok, A. S. Dmitrenok, B. B. Grebnev, and V. A. Stonik, Chem. Nat. Compd., 2008, 44, 372. CrossRef