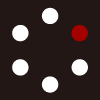
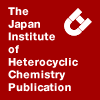
HETEROCYCLES
An International Journal for Reviews and Communications in Heterocyclic ChemistryWeb Edition ISSN: 1881-0942
Published online by The Japan Institute of Heterocyclic Chemistry
e-Journal
Full Text HTML
Received, 7th August, 2013, Accepted, 20th August, 2013, Published online, 23rd August, 2013.
DOI: 10.3987/COM-13-12804
■ C–H MODIFICATION OF 2-AZAADAMANTANE: SYNTHESIS OF C5-FUNCTIONALIZED AZADOS FOR ADVANCED USE
Yusuke Sasano, Tomohiro Nishiyama, Masaki Tomizawa, Masatoshi Shibuya, and Yoshiharu Iwabuchi*
Graduate School of Pharmaceutical Sciences, Tohoku University, 6-3 Aobayama, Sendai 980-8578, Japan
Abstract
Regioselective C–H functionalization of N-protected 2-azaadamantane was achieved via a radical-induced bromination. The obtained 5-bromo-2-azaadamantane is readily transformed to arylated/alkylated 2-azaadamantane N-oxyls [AZADOs], which exhibit high catalytic activities for alcohol oxidation.2-Azaadamantane (1) is a structurally salient class of polyalicyclic secondary amines that have found numerous applications in organic and medicinal chemistry.1 We have recently disclosed that N-monooxygenated derivatives of 2-azaadamantane, namely, 2-azaadamantane N-oxyl [AZADO (2)], 2-azaadamantan-2-ol [AZADOL (3)], and 2-oxo-2-azaadamantan-2-ium (4), function as highly active organocatalysts for alcohol oxidation and have exploited their versatile use in synthetic organic chemistry.2 To further advance the use of 2-azaadamantane, we envisioned the installation of a functional group onto the 2-azaadamantane skeleton, which will allow a covalent linking of 2-azaadamantanes to a variety of functional vehicles such as solid/fluorous phases, hydrophilic/hydrophobic terminals, and luminophores. Regarding the modification sites of 2-azaadamantane, C1 and C4 sites are well precedented: these substituents can be expediently installed in 2-azaadamantane during the construction of the 2-azaadamantane skeleton from bicyclic precursors.3 In contrast, C5/C6-substituted 2-azaadamantanes have rarely been synthesized.4,1a We herein report C5-selective modification methods for 2-azaadamantane that ultimately transform it into two distinct AZADO derivatives with an aryl/alkyl group for advanced use.
In designing tethered AZADOs, we reviewed the structure–activity relationship (SAR) of AZADOs, which indicates that (i) steric hindrance around the nitroxyl radical moiety significantly attenuates catalytic activities,2b (ii) electron-withdrawing groups on the azaadamantane skeleton decrease the rate of alcohol oxidation.5 Thus, the tether should be linked with AZADO not via a C–heteroatom bond but via a C–C bond at a remote position from the nitroxyl radical moiety (C5 or C6). We envisaged that 5-halogenated 2-azaadamantane 6 would be obtained by the C–H halogenation of N-protected 2-azaadamantane 7 and play roles as the key intermediate for the synthesis of 5-arylated/alkylated AZADO 5.
On the basis of our knowledge of the C–H oxygenation of N-protected 2-azaadamantanes,6 N-trifluoroacetyl-2-azaadamantane (8) was selected as a substrate and C–H bromination was conducted under standard conditions using AIBN as the radical initiator in the presence of NBS in boiling CCl4, from which an 11% and a 13% of 5-bromo-2-azaadamantane 9 and 5-chloro-2-azaadamantane 10, respectively, was obtained, accompanied by the recovery of amide 8 (Scheme 2). Note that other halogenated azaadamantanes (e.g., 1-bromo-2-azaadamantane) were not obtained.
Encouraged by the excellent regioselectivity of the halogenation, the reaction conditions were optimized (Table 1). The use of CH2Br2 as the solvent instead of CCl4 suppressed the chlorination to generate 5-Br-2-azaadamantane 9 as a major product in modest conversion (entry 1). Lowering the reaction temperature to 70 °C improved the conversion (entry 2). Further improvement was achieved by the addition of 0.5 eq. of bromine to afford bromide 9 in 70% isolated yield (entry 3). This bromination proceeded in comparatively high yield on a 9-grams scale. Note that NBS was essential for the bromination of this particular substrate; however, the bromination of adamantane proceeded without the addition of NBS and AIBN (entry 4).7
Having obtained a good yield of 5-Br-2-azaadamantane 9, effort was next focused on installing an aryl/alkyl group using bromide 9. The hydroxyphenyl group was installed by treating 9 with phenol in the presence of FeCl38 at 100 °C to yield 78% 5-(p-hydroxyphenyl)-2-azaadamantane 11 together with 13% o-isomer 12. After chromatographic separation, phenol 11 was benzylated to yield ether 13. Upon a sequential deprotection of the trifluoroacetyl group using aqueous KOH in the presence of triethyl(benzyl)ammonium chloride as the phase-transfer catalyst,9 and the oxidation of the resulting amine under conventional conditions, trifluoroacetamide 13 yielded 5-(p-(benzyloxy)phenyl)-AZADO [5-BP-AZADO (14)].
On the other hand, a C3 alkyl chain was installed by treating bromide 9 with methyl acrylate in the presence of AIBN and Bu3SnH to yield 71% methyl propanoate 16 (Scheme 4). The treatment of ester 16 with LiAlH4 led to the reduction of the ester and the concomitant deprotection of the trifluoroacetyl group to yield the corresponding amino-alcohol, the oxidation of the amine moiety of which afforded 5-(3-hydoxypropyl)-AZADO [5-HP-AZADO (17)]. Acetylation of 5-HP-AZADO (17) yielded 5-(3-acetoxypropyl)-AZADO [5-AP-AZADO (19)].
With the intended arylated and alkylated AZADOs in hand, their catalytic activities were evaluated under Anelli’s conditions using NaOCl as the terminal oxidant (Table 2).10 Both 5-BP-AZADO (14) and 5-AP-AZADO (19) efficiently oxidized various hindered secondary alcohols, including a high yield of sugar derivative 20g at only 1 mol% catalyst loading, indicating their potential advanced use by attaching them to various materials.
In summary, regioselective C–H bromination of N-trifluoroacetyl-2-azaadamantane (8) was developed to afford a good yield of 5-Br-2-azaadamantane 9. 5-Br-2-azaadamantane 9 was readily converted to arylated/alkylated AZADOs, namely, 5-BP-AZADO (14) and 5-AP-AZADO (19), which efficiently catalyzed alcohol oxidation. The application of 5-BP-AZADO (14) and 5-AP-AZADO (19) to the synthesis of polymer-supported AZADO is under investigation.
EXPERIMENTAL
All reactions were stirred magnetically under argon atmosphere, unless otherwise noted. Dehydrated THF and CH2Cl2 were purchased from Kanto Chemical Co., Inc. Other anhydrous solvents were dried and distilled according to standard protocols. N-Bromosuccinimide (NBS) was recrystallized from boiling water before usage. Other reagents were obtained from commercial suppliers and used without further purification, unless otherwise noted.
Reactions were monitored by thin-layer chromatography (TLC) carried out on silica gel plates (Merck Silica gel 60 F254). Column chromatography was performed on Silica gel 60N (Kanto Chemical Co., Inc., spherical, neutral, 63–210 μm) and flash column chromatography was performed on Silica gel 60N (Kanto Chemical Co., Inc., spherical, neutral, 40–50 μm).
IR spectra were recorded on a JASCO FT/IR-410 Fourier Transform Infrared Spectrometer. NMR spectra were recorded on JEOL JNM-AL-400 spectrometers. Chemical shifts for 1H-NMR spectra are reported in parts per million (ppm) downfield from TMS (0.00 ppm) as the internal standard and coupling constants are expressed in Hertz (Hz). The following abbreviation are used for spin multiplicity: s = singlet, d = doublet, t = triplet, q = quartet, m = multiplet and br = broad. Chemical shifts for 13C-NMR are reported in ppm, relative to the central line of a triplet at 77.0 ppm for 13CDCl3. Mass spectra were recorded on JEOL JMS-DX303, JEOL JNM-AL500 and JEOL JMS-700. Elemental analyses were performed by Yanaco CHN CORDER MT-6.
Synthesis of Functionalized AZADOs
5-Bromo-N-trifluoroacetyl-2-azaadamantane (9)
A solution of N-trifluoroacetyl-2-azaadamantane (8, 9.39 g, 40.3 mmol)4 in CH2Br2 (81 mL) was degassed by repeated sonication under argon atmosphere. To the solution were added NBS (35.8 g, 0.201 mol), Br2 (1.03 mL, 20.1 mmol), and AIBN (661 mg, 4.03 mmol) at room temperature. After the mixture was stirred for 12 h at 70 °C, the mixture was concentrated in vacuo. Et2O was added to the residue, and the suspended white solids were removed by filtration through a Celite pad. The filtrate was concentrated in vacuo and the residue was purified by flash column chromatography (1:30 to 1:15 AcOEt:hexane) to give bromide 9 (7.59 g, 24.3 mmol, 60%) as a colorless oil.
IR (neat, cm-1): 2938, 1687, 1508. 1H-NMR (400 MHz, CDCl3): δ 4.87 (br s, 1H), 4.34 (br s, 1H), 2.54-2.37 (m, 6H), 2.34 (br s, 1H), 2.00-1.75 (m, 4H). 13C-NMR (100 MHz, CDCl3): δ 154.3 (q, J = 36.0 Hz), 116.5 (q, J = 288.4 Hz), 58.5, 52.3 (q, J = 3.8 Hz), 49.1, 47.1, 46.8, 46.5, 33.4, 33.5, 30.2. MS m/z: 311 (M+), 232 (100%). HRMS (EI): Calcd. for C11H1379BrF3NO 311.0133 (M+), found: 311.0114.
5-(p-Hydroxyphenyl)-N-trifluoroacetyl-2-azaadamantane (11)
To a solution of bromide 9 (5.04 g, 16.2 mmol) in phenol (32 mL) was added FeCl3 (3.14 g, 19.4 mmol) at 40 °C. After the mixture was stirred for 3 h at 100 °C, the mixture was concentrated in vacuo. CHCl3 and 2 N HCl were added to the residue, and the mixture was extracted with CHCl3. The organic extract was washed with brine, dried (MgSO4), and concentrated in vacuo. The residue was purified by column chromatography (1:8 to 1:4 AcOEt:hexane) to give phenol 11 (4.08 g, 12.5 mmol, 78%) as a white solid.
IR (neat, cm-1): 3301, 1661. 1H-NMR (400 MHz, CDCl3): δ 7.16 (d, J = 8.7 Hz, 2H), 6.81 (d, J = 8.7 Hz, 2H), 5.11 (br s, 1H), 4.93 (br s, 1H), 4.40 (br s, 1H), 2.34 (brs, 1H), 2.12-1.90 (m, 8H), 1.90-1.70 (m, 2H). 13C-NMR (100 MHz, CDCl3): δ 154.3 (q, J = 35.2 Hz), 154.1, 140.4, 125.8, 116.8 (q, J = 288.4 Hz), 115.3, 50.2 (q, J = 3.6 Hz), 46.7, 41.7, 41.3, 40.7, 35.4, 34.8, 34.5, 27.1. MS m/z: 325 (M+), 325 (100%). HRMS (EI): Calcd. for C17H18F3NO2 325.1290 (M+), found: 325.1298.
5-(p-Benzyloxyphenyl)-N-trifluoroacetyl-2-azaadamantane (13)
To a solution of phenol 11 (1.00 g, 3.07 mmol) in DMF (15.4 mL) were added K2CO3 (1.27 g, 9.19 mmol), benzyl chloride (0.53 mL, 4.6 mmol), tetrabutylammonium iodide (114 mg, 0.309 mmol) at 0 °C. After the mixture was stirred for 24 h at room temperature, saturated aqueous NH4Cl was added and the mixture was extracted with CHCl3. The organic extract was dried (MgSO4), and concentrated in vacuo. The residue was purified by flash column chromatography (1:8 AcOEt:hexane) to give ether 13 (1.21 g, 2.91 mmol, 95%) as a white solid.
IR (neat, cm-1): 1679. 1H-NMR (400 MHz, CDCl3): δ 7.52-7.27 (m, 5H), 7.21 (d, J = 8.9 Hz, 2H), 6.95 (d, J = 8.9 Hz, 2H), 5.04 (s, 2H), 4.93 (br s, 1H), 4.39 (br s, 1H), 2.33 (br s, 1H), 2.13-1.88 (m, 8H), 1.88-1.72 (m, 2H). 13C-NMR (100 MHz, CDCl3): δ 157.3, 154.1 (q, J = 35.2 Hz), 140.6, 137.0, 128.6, 128.0, 127.5, 125.6, 116.8 (q, J = 288.4), 114.7, 70.0, 50.1 (q, J = 3.6 Hz), 46.6, 41.7, 41.3, 40.7, 35.4, 34.8, 34.5, 27.1. MS m/z: 415 (M+), 91 (100%). HRMS (EI): Calcd. for C24H24F3NO2 415.1759 (M+), found: 415.1748.
5-(p-Benzyloxyphenyl)-2-azaadamantane N-oxyl [5-BP-AZADO (14)]
To a solution of ether 13 (32.5 mg, 78.2 μmol) in THF (0.39 mL) were added 20% aqueous KOH (0.22 mL), benzyltriethylammonium chloride (1.8 mg, 7.9 μmol) at room temperature and the mixture was stirred for 35 h at 90 °C. After cooling, CHCl3 and H2O were added and the mixture was extracted with CHCl3. The organic extract was dried (K2CO3), and concentrated in vacuo to give the amine product that was used in the next reaction without further purification. A solution of the crude amine and Na2WO4·2H2O (12.9 mg, 39.1 μmol) in MeCN (0.16 mL) was stirred for 30 min at room temperature. To the mixture was added urea·H2O2 (29.4 mg, 0.313 mmol) at room temperature. After 2 h, additional urea·H2O2 (7.35 mg, 78.2 μmol) was added and the mixture was stirred for 1 h. The mixture was diluted with saturated aqueous NaHCO3 and the mixture was extracted with CHCl3. The organic extract was dried (K2CO3), and concentrated in vacuo. The residue was purified by flash column chromatography (1:2 AcOEt:hexane) to give 5-BP-AZADO (14, 8.63 mg, 25.8 μmol, 33% for 2 steps) as a yellow solid.
IR (neat, cm-1): 2910, 1507. MS m/z: 334 (M+), 262 (100%). HRMS (EI): Calcd. for C22H24NO2 334.1807 (M+), found: 334.1800.
Methyl 3-(N-(Trifluoroacetyl)-2-azaadamantan-5-yl)propanoate (16)
A solution of bromide 9 (1.14 g, 3.64 mmol) in toluene (7.3 mL) was degassed by argon bubbling for 10 min. To the solution were added methyl acrylate (0.982 mL, 10.91 mmol), Bu3SnH (1.96 mL, 7.17 mmol), and AIBN (59.7 mg, 0.364 mmol) at room temperature and the mixture was stirred for 1 h at reflux. After cooling to room temperature, 10% aqueous KF (10 mL) was added. After the mixture was stirred for 1 h, the mixture was extracted with AcOEt. The organic extract was washed with brine, dried (MgSO4), and concentrated in vacuo. The residue was purified by flash column chromatography (1:4 AcOEt:hexane) to give ester 16 (828 mg, 2.59 mmol, 71%) as a colorless oil.
IR (neat, cm-1): 1739, 1685. 1H-NMR (400 MHz, CDCl3): δ 4.82 (br s, 1H), 4.28 (br s, 1H), 3.68 (s, 3H), 2.31-2.14 (m, 3H), 1.86 (br s, 2H), 1.78-1.46 (m, 10H). 13C-NMR (100 MHz, CDCl3): δ 174.2, 154.1 (q, J = 35.2 Hz), 116.8 (q, J = 288.4 Hz), 51.7, 49.8, 46.3, 40.5, 39.7, 39.5, 37.5, 35.7, 34.7, 31.5, 27.6, 26.7. MS m/z: 319 (M+), 232 (100%). HRMS (EI): Calcd. for C15H20F3NO3 319.1395 (M+), found: 319.1394.
5-(3-Hydoxypropyl)-AZADO [5-HP-AZADO (17)]
To a solution of ester 16 (657 mg, 2.06 mmol) in THF (10.3 mL) was added LiAlH4 (312 mg, 8.22 mmol) portionwise at 0 °C. After the mixture was stirred for 30 min, Et2O (5 mL) and 30% aqueous NH3 (2.03 mL) were added slowly. After the mixture was stirred for 1 h, the mixture was filtered through a Celite pad. The filtrate was concentrated by in vacuo to give an amino-alcohol product that was used in the next reaction without further purification. A solution of the crude amino-alcohol and Na2WO4·2H2O (339 mg, 1.03 mmol) in MeOH (4.1 mL) was stirred for 30 min at room temperature. To the mixture was added urea·H2O2 (774 mg, 8.23 mmol) at room temperature. After 30 min, additional urea·H2O2 (193 mg, 2.06 mmol) was added and the mixture was stirred for another 30 min. The mixture was diluted with saturated aqueous NaHCO3 and the mixture was extracted with CHCl3. The organic extract was dried (K2CO3), and concentrated in vacuo. The residue was purified by flash column chromatography (1:20 MeOH:AcOEt) to give 5-HP-AZADO (17, 178 mg, 0.846 mmol, 41% for 2 steps) as a yellow solid.
IR (neat, cm-1): 3371. MS m/z: 210 (M+), 210 (100%). HRMS (EI): Calcd. for C12H20NO2 210.1492 (M+), found: 210.1486. Anal. Calcd for C12H20NO2: C, 68.54; H, 9.59; N, 6.66; found: C, 67.38; H, 9.29; N, 6.44.
5-(3-Acetoxypropyl)-AZADO [5-AP-AZADO (19)]
To a solution of 5-HP-AZADO (17, 45.4 mg, 0.216 mmol) in CH2Cl2 (1.1 mL) were added Et3N (90.2 μL, 0.647 mmol), AcOH (18.5 μL, 0.323 mmol), DIC (0.101 mL, 0.645 mmol), and DMAP (5.27 mg, 43.1 μmol) at room temperature. After the mixture was stirred for 6 h, CH2Cl2 and H2O were added and the mixture was extracted with CH2Cl2. The organic extract was dried (K2CO3), and concentrated in vacuo. The residue was purified by column chromatography (1:1 AcOEt:hexane) to give 5-AP-AZADO (19, 50.4 mg, 0.200 mmol, 92%) as a red oil.
IR (neat, cm-1): 3298, 1739, 1703, 1662. MS m/z: 252 (M+), 180 (100%). HRMS (EI): Calcd. for C14H22NO3 252.1600 (M+), found: 252.1582.
General Procedure for Alcohol Oxidation
A test tube equipped with magnetic stirring bar was charged with a solution of alcohol 20 (1.00 mmol), ethered AZADO 14 or 19 (10 μmol) and KBr (11.9 mg, 0.100 mmol) in CH2Cl2 (2.7 mL) and sat. NaHCO3 (1 mL). To this cooled (0 °C, a water-ice bath) and well-stirred (800 rpm) mixture was added pre-mixed solution of aqueous NaOCl (1.0 mL, 1.5 mmol: 1.45 M, purchased from Junsei Chemical Co., Ltd. and titrated) and sat. NaHCO3 (1.7 mL) dropwise over 5 min. The reaction mixture was stirred for 20 min at 0 °C, then quenched with 20% aqueous Na2S2O3 (3 mL). The aqueous layer was separated and extracted with CH2Cl2. The combined organic layers were washed with brine, dried over MgSO4 and concentrated in vacuo. The residue was purified by flash column chromatography (Et2O/hexane) to give the corresponding carbonyl compound 21 in 87–98% yield.
ACKNOWLEDGEMENTS
This work was partly supported by a Grant-in-Aid for Scientific Research on Innovative Areas “Advanced Molecular Transformations by Organocatalysis” from the Ministry of Education, Culture, Sports, Science and Technology, Japan, by a Grant-in-Aid for Scientific Research (B) (No. 24390001), and by a Grant-in-Aid for Young Scientists (B) (No. 25860001) from the Japan Society for the Promotion of Science (JSPS).
References
1. † Current address: Department of Basic Medicinal Sciences, Graduate School of Pharmaceutical Sciences, Nagoya University, Furo-cho, Chikusa-ku, Nagoya 464-8601, Japan..
2. For recent examples, see the following: (a) M. C. Frantz, E. M. Skoda, J. R. Sacher, M. W. Epperly, J. P. Goff, J. S. Greenberger, and P. Wipf, Org. Biomol. Chem., 2013, 11, 4147; CrossRef (b) E. Darout, R. P. Robinson, K. F. McClure, M. Corbett, B. Li, A. Shavnya, M. P. Andrews, C. S. Jones, Q. F. Li, M. L. Minich, V. Mascitti, C. R. W. Guimaraes, M. J. Munchhof, K. B. Bahnck, C. M. Cai, D. A. Price, S. Liras, P. D. Bonin, P. Cornelius, R. D. Wang, V. Bagdasarian, C. P. Sobota, S. Hornby, V. M. Masterson, R. M. Joseph, A. S. Kalgutkar, and Y. Chen, J. Med. Chem., 2013, 56, 301; CrossRef See also: (c) S. D. Banister, D. T. Yoo, S. W. Chua, J. Q. Cui, R. H. Mach, and M. Kassiou, Bioorg. Med. Chem. Lett., 2011, 21, 5289; CrossRef (d) D. D. Dixon, D. Sethumadhavan, T. Benneche, A. R. Banaag, M. A. Tius, G. A. Thakur, A. Bowman, J. T. Wood, and A. Makriyannis, J. Med. Chem., 2010, 53, 5656. CrossRef
3. (a) M. Shibuya, Y. Sasano, M. Tomizawa, T. Hamada, M. Kozawa, N. Nagahama, and Y. Iwabuchi, Synthesis, 2011, 3418; CrossRef (b) M. Shibuya, M. Tomizawa, I. Suzuki, and Y. Iwabuchi, J. Am. Chem. Soc., 2006, 128, 8412. CrossRef
4. (a) B. Graetz, S. Rychnovsky, W. H. Leu, P. Farmer, and R. Lin, Tetrahedron: Asymmetry, 2005, 16, 3584; CrossRef (b) J. T. Hane and J. G. Henkel, Tetrahedron Lett., 1990, 31, 2949; CrossRef (c) T. Sasaki, S. Eguchi, N. Toi, T. Okano, and Y. Furukawa, J. Chem. Soc., Perkin Trans. 1, 1983, 2529; CrossRef (d) T. Sasaki, S. Eguchi, T. Okano, and Y. Wakata, J. Org. Chem., 1983, 48, 4067; CrossRef (e) T. Sasaki, S. Eguchi, and T. Okano, Tetrahedron Lett., 1982, 23, 4969; CrossRef (f) J. G. Henkel, W. C. Faith, and J. T. Hane, J. Org. Chem., 1981, 46, 3483; CrossRef (g) J. G. Henkel and W. C. Faith, J. Org. Chem., 1981, 46, 4953; CrossRef (h) W. P. Meyer and J. C. Martin, J. Am. Chem. Soc., 1976, 98, 1231; CrossRef (i) W. H. Staas and L. A. Spurlock, J. Org. Chem., 1974, 39, 3822; CrossRef (j) R. J. Schultz, W. H. Staas, and L. A. Spurlock, J. Org. Chem., 1973, 38, 3091; CrossRef (k) H. Stetter, P. Tacke, and J. Gartner, Chem. Ber., 1964, 97, 3480. CrossRef
5. M. Shibuya, Y. Osada, Y. Sasano, M. Tomizawa, and Y. Iwabuchi, J. Am. Chem. Soc., 2011, 133, 6497. CrossRef
6. M. Shibuya, F. Pichierri, M. Tomizawa, S. Nagasawa, I. Suzuki, and Y. Iwabuchi, Tetrahedron Lett., 2012, 53, 2070. CrossRef
7. A preliminary set of experiments of C–H oxygenation of N-protected 2-azaadamantanes indicated that the trifluoroacetyl group is the protecting group of choice not only for durability against oxidative conditions, but also for reasonable reactivity under alkaline deprotection conditions: The N-Cbz substrate was poorly reactive and the N-Boc substrate suffered from the deprotection of the Boc group under Sharpless’ conditions using NaIO4 and RuCl3. See, ref. 4.
8. S. E. Denmark and B. R. Henke, J. Am. Chem. Soc., 1991, 113, 2177. CrossRef
9. R. Perkins, S. Bennett, E. Bowering, J. Burke, K. Reid, and D. Wall, Chem. Ind. (London), 1980, 790.
10. D. Albanese, F. Corcella, D. Landini, A. Maia, and M. Penso, J. Chem. Soc., Perkin Trans. 1, 1997, 247. CrossRef
11. (a) P. L. Anelli, S. Banfi, F. Montanari, and S. Quici, J. Org. Chem., 1989, 54, 2970; CrossRef (b) P. L. Anelli, C. Biffi, F. Montanari, and S. Quici, J. Org. Chem., 1987, 52, 2559. CrossRef