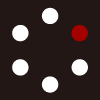
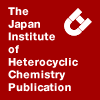
HETEROCYCLES
An International Journal for Reviews and Communications in Heterocyclic ChemistryWeb Edition ISSN: 1881-0942
Published online by The Japan Institute of Heterocyclic Chemistry
e-Journal
Full Text HTML
Received, 7th May, 2014, Accepted, 20th June, 2014, Published online, 25th June, 2014.
■ Synthetic Routes towards Benzofuro[2,3-b]pyrroles and Benzofuro[2,3-b]indoles
Yuta Ito, Masafumi Ueda,* and Okiko Miyata*
Department of Medicinal Chemistry, Kobe Pharmaceutical University, 4-19-1, Motoyamakitamachi, Higashinada, Kobe 658-8558, Japan
Abstract
This review provides a summary of the synthetic methods currently available for the construction of benzofuro[2,3-b]pyrroles and benzofuro[2,3-b]indoles. The latter of these two scaffolds features as a key structural element in characteristic bioactive natural products.CONTENTS
1. Introduction
2. Synthesis of benzofuro[2,3-b]pyrrole
2.1. Synthesis from benzofuran
2.2. Synthesis from azirinocyclobutabenzofuran
2.3. Synthesis from benzo[f]chromen-3-one
2.4. Synthesis via 4-imino-3-(2-hydroxyphenyl)butanoate intermediate
2.5. Synthesis via 2-(3,4-dihydro-2H-pyrrol-4-yl)phenol intermediate
3. Synthesis of benzofuro[2,3-b]indole
3.1 Synthesis from indole
3.2 Synthesis from oxindole
3.3 Synthesis from indoline
4. Conclusion
1. INTRODUCTION
Tricyclic heterocycles 1 are key structural elements in a large number of natural and synthetic products that exhibit a wide range of bioactivities (Scheme 1). For example, the hexahydropyrrolo[2,3-b]indole1 N,N–2 can be found in compounds such as physostigmine (7) and phenserine (8), which are used to treat Alzheimer’s disease and glaucoma,2,3 whereas the tetrahydrofuro[2,3-b]indole N,O–3 can be found in madindolines A (9) and B (10), which have been reported to be selective inhibitors of interleukin-6. 4 The tetrahydrofuro[2,3-b]benzofuran O,O–4 is present in the potent carcinogenic aflatoxins 11–14.5 However, very few biological studies of tetrahydrobenzofuro[2,3-b]pyrrole O,N–5 have been performed, despite their biological potential. Therefore, there is an urgent need for the development of synthetic methods capable of providing facile access to tetrahydrobenzofuro[2,3-b]pyrroles to allow for the effective evaluation of the biological properties.
Furthermore, indole-fused benzofurans, such as dihydrobenzofuro[2,3-b]indole 6, can be found in characteristic bioactive natural products such as diazonamide A (15) and azonazine (16).6,7 For this reason, there has been a significant increase in research efforts directed towards the development of novel methods for the construction of dihydrobenzofuro[2,3-b]indoles. In this review, we have provided a summary of the existing methods for the synthesis of benzofuro[2,3-b]pyrroles and benzofuro[2,3-b]indoles.8
2. SYNTHESIS OF BENZOFURO[2,3-b]PYRROLE
The methods available for the synthesis of benzofuro[2,3-b]pyrroles can be divided into several categories, including (i) synthesis from benzofuran; (ii) synthesis from azirinocyclobutabenzofuran; (iii) synthesis from benzo[f]chromen-3-one; (iv) synthesis via 4-imino-3-(2-hydroxyphenyl)butanoate intermediate; and (v) synthesis via 2-(3,4-dihydro-2H-pyrrol-4-yl)phenol intermediate.
2.1 Synthesis from benzofuran
Oshima and Shinokubo9 developed a novel method for the preparation of a benzofuro[2,3-b]pyrrole from benzofuran that involved the radical [3 + 2] annulation reaction of N-allyl-N-chlorotosylamide (Scheme 2). The reaction of benzofuran (17) with N-allyl-N-chlorotosylamide (18) in the presence of 10 mol% of triethylborane afforded 3-chloromethylbenzofuro[2,3-b]pyrrole 19 in 82% yield (endo:exo = 83:17).
This radical reaction was initiated by the generation of the N-centered radical 20, which reacted with benzofuran to form the alkyl radical 21. Subsequent 5-exo radical cyclization would give the primary radical 22, which would abstract a chlorine atom from 18 to afford 3-chloromethylbenzofuro[2,3-b]pyrrole 19 and regenerate the N-centered radical 20.
The same authors also reported the synthesis of a benzofuro[2,3-b]pyrrole bearing a dichloroethyl group. Briefly, a mixture of benzofuran (17) and N-chloro-N-(4-chloro-2-butenyl)benzenesulfonamide (23) was treated with 10 mol% of triethylborane to give 3-(1,2-dichloroethyl)benzofuro[2,3-b]pyrrole 24 in 80% yield (Scheme 3).10
Padwa’s group11,12 reported that the reaction of 2-benzofuranylsulfilimine with dichloroketene afforded benzofuro[2,3-b]pyrrole. Benzofuran (17) reacted with n-butyllithium and diethyl disulfide to afford 2-ethylthiobenzofuran, which was then treated with chloramine-T to furnish the desired 2-benzofuranylsulfilimine 25 (Scheme 4). The subsequent reaction of 25 with dichloroketene afforded the 3,3-dichloro-8a-ethylthiobenzofuro[2,3-b]pyrrol-2-one 28 in 52% yield.
This process involved the nucleophilic addition of the nitrogen atom to the highly electrophilic dichloroketene to generate the zwitterionic intermediate 26. Intermediate 26 then underwent [3,3]-sigmatropic rearrangement to form the Pummerer cation 27, which was trapped by the intramolecular attack of the amido anion to give benzofuro[2,3-b]pyrrole 28.
Broggini and co-workers13 reported the intramolecular Pd(II)-catalyzed cyclization of 2-(N-allyl-N-carbethoxyamino)benzofuran as a method for the construction of benzofuro[2,3-b]pyrrole 31. 2-(N-allyl-N-carbethoxyamino)benzofuran (30) was prepared in four steps from benzofuran-2-carboxylic acid (29) (Scheme 5). The cyclization of 30 was carried out in the presence of catalytic amount of PdCl2(MeCN)2 and CuCl2 under oxygen atmosphere to give the aromatized benzofuro[2,3-b]pyrrole 31 in 23% yield.
A plausible mechanism for this intramolecular Pd(II)-catalyzed cyclization is shown in Scheme 6. The intramolecular nucleophilic attack of benzofuran (C3) to the palladium(II)-activated olefin would afford the σ-complex 32, which would undergo β-hydride elimination to give the cyclization product 33. The exomethylene 33 would then readily isomerize to give the final product 31 together with Pd(II).
2.2. Synthesis from azirinocyclobutabenzofuran
Benzofuro[2,3-b]pyrroles have also been synthesized by the thermal skeletal rearrangement of the azirinocyclobutabenzofurans 35a-c, which were prepared from the corresponding 1-benzoxepines 34a-c by photocycloaddition followed by treatment with ethoxycarbonylnitrene generated in situ from ethyl nosyloxycarbamate (NsONHCO2Et; Ns = 4-NO2C6H4SO2).14 Azirinocyclobutabenzofurans 35a-c were then heated in a sealed tube at 160 °C, which resulted in a rearrangement reaction to give the corresponding benzofuro[2,3-b]pyrroles 36a-c (40–70%) together with their [3,2-b]isomers 37a-c (5–20%) (Scheme 7).
A plausible mechanism for the thermolysis reaction is shown in Scheme 8. The conversion of the ring system in 35 to the regioisomers 36 and 37 most likely proceeds via the cleavage of the C–C bond of the aziridine ring by electrocyclic reaction to afford the azomethine ylide intermediates 38, which could undergo migration of the phenoxy group to give 36. The other regioisomer 37 could also be formed from 38 by a Wagner-Meerwein type phenyl group migration followed by a shift of the phenoxy group.
2.3. Synthesis from benzo[f]chromen-3-one
Soman and Thaker15 reported that the condensation of 2-bromo-1-methyl-3H-benzo[f]chromen-3-one (39) with a series of aromatic primary amines gave the tetracyclic naphthofuro[2,3-b]pyrroles 40a–d (Scheme 9).
2.4. Synthesis via 4-imino-3-(2-hydroxyphenyl)butanoate intermediate
The first documented synthesis of a benzofuro[2,3-b]pyrrole was reported by Connor and Strandtmann in 1973.16 The treatment of imines 41a and 41b with zinc in acetic acid gave the benzofuro[2,3-b]pyrrol-2-ones 42a and 42b via the reduction of the C–C double bond followed by a transannulation reaction (Scheme 10).
In contrast, the application of the same conditions to oxime 43 afforded the condensation product 44 instead of the N-hydroxybenzofuro[2,3-b]pyrrole 45. The reaction mechanism proposed for this transformation is shown in Scheme 11. There is an equal probability for the reduction of either C–C or C–N double bond resulting in the production of approximately equal amounts of 46 and 47. These compounds could then condense with each other to give 48, which would undergo a double cyclization reaction to afford 44.
The application of the these conditions to the oxime derivative 49 also afforded the corresponding naphthofuro[2,3-b]pyrrol-2-one 50 in 73% yield (Scheme 12).
Usgaonkar’s group17,18 expanded on this work when they reported that benzofuro[2,3-b]pyrroles bearing a variety of different substituents could be synthesized by the reductive transannulation of oximes 51a–e (Scheme 13).
Harada et al.19 reported that the hydrogenation of the 4-methoxycarbonylmethylidene-1,2-benzoxazines 53a–e with PtO2 in acetic acid produced the benzofuro[2,3-b]pyrrol-2-ones 54a–e in moderate yields (Scheme 14). This transformation was presumably initiated by the reductive cleavage of the 1,2-oxazine ring followed by a double cyclization sequence to give 54.
Our research group20 recently reported the development of a facile method for the construction of benzofuro[2,3-b]pyrroles using a novel domino reaction. Treatment of the O-phenyl conjugated oxime ether 55 with triethylborane as an ethyl radical source afforded the desired 3-ethylbenzofuro[2,3-b]pyrrol-2-one 56a in 95% yield as a 1:1 mixture of diastereomers at the C3 position (Table 1, entry 1).
Pleasingly, the use of secondary alkyl radicals in this reaction led to a significant improvement in the stereoselectivity (Table 1, entries 3 and 4). Interestingly, the use of a tert-butyl iodide led to the formation of the corresponding exo-product 56e as a single stereoisomer (Table 1, entries 5 and 6).
We then proceeded to investigate the impact of different substituents on the phenyl group, with respect to the domino reaction initiated by the addition of the ethyl and tert-butyl radicals (Scheme 15). The results of this investigation revealed that trifluoromethyl, methyl and bromo substituents were well tolerated under these reaction conditions. Particularly, domino reactions conducted with the tert-butyl radical gave the corresponding exo-3-tert-butylbenzofuro[2,3-b]pyrroles 59 in good yields with excellent stereoselectivities.
A possible reaction pathway for this transformation that accounts for the formation of 56e is shown in Scheme 16. This mechanism involves the regioselective addition of a tert-butyl radical to the C–C double bond of 55 to afford the aminyl radical 60, which is then trapped by Et3B to form the N-borylenamine 61 (Scheme 16-(a)). The N-boryl-N-phenoxyenamine 61 then undergoes a [3,3]-sigmatropic rearrangement via a six-membered transition state, which minimizes the steric repulsion between the tert-butyl and phenyl groups, to allow for the stereoselective formation of the syn-α-arylimine 62 (Scheme 16-(b)). Given that the subsequent cyclization of 62 would be reversible, an equilibrium would exist between the cis- and trans-isomers of 63. The irreversible lactamization would then push the equilibrium towards the formation of the sterically favored cis-fused tricycle 56e.
Furthermore, we demonstrated the asymmetric synthesis of benzofuro[2,3-b]pyrroles via the traceless cleavage of a chiral auxiliary (Scheme 17). Triethylborane was added to a solution of the chiral conjugated oxime ether 64 and trimethylaluminum in acetonitrile at −40 °C, and the resulting mixture was heated at reflux. In contrast to the reaction involving the oxime ether 55 bearing a pentafluorophenyl ester, this reaction afforded the endo-isomer 65 as the major product in 57% yield with 93% ee. This result therefore confirmed that the [3,3]-sigmatropic rearrangement had proceeded in such a way as to avoid the occurrence of a steric interaction of the camphorsultam moiety with the phenyl group in the six-membered transition state.
2.4. Synthesis via 2-(3,4-dihydro-2H-pyrrol-4-yl)phenol intermediate
Benzofuro[2,3-b]pyrrole-1-oxides were synthesized to explore the reactivity and utility of the nitrone 66.21 The treatment of nitrone 66 with AlCl3 and Me2S led to the formation of the benzofuro[2,3-b]pyrrole-1-oxides 67a and 67b in a combined yield of 76% (Scheme 18).
Tius and Kerr22 reported the preparation of benzofuro[2,3-b]pyrrole as an intermediate in the synthesis of a series of morphine alkaloids. Exposure of 68 to boron trifluoride etherate at −30 °C led to the formation of 69 via sequential aromatization and hemiaminalization reactions (Scheme 19). This method has also been used for the synthesis of dihydrobenzofuro[2,3-b]indoles, and this work will be discussed in the following chapter.
3. SYNTHESIS OF DIHYDROBENZOFURO[2,3-b]INDOLE
One of the most commonly used strategies for the synthesis of dihydrobenzofuro[2,3-b]indoles involves the cyclization of 3-(2-hydroxyphenyl)indolenine 70 or indolenium, which can be prepared from the corresponding indoles, oxindoles and indolines (Scheme 20).
3.1. Synthesis from indole
As part of synthetic studies towards the total synthesis of diazonamide A, Harran’s group23 reported the intramolecular oxidative cycloaddition of a hydroxyphenyl group with an indole. In this case, 71 reacted with PhI(OAc)2 and LiOAc to give the benzofuro[2,3-b]indoles 73 in 20–25% yield (Scheme 21). Mechanistically, oxidation of the phenol would initiate an annulation reaction between the phenoxenium ion and the tethered indole to give the cyclohexadienone-linked indolenium species 72, which would undergo a ring closing reaction to give 73. The possibility that the reaction was initiated by the single electron oxidation of the indole subunit, however, cannot be excluded.
Yao and co-workers24 have successfully accomplished the first total synthesis of (–)-azonazine using a biomimetic oxidative cyclization as a key step for the construction of the benzofuro[2,3-b]indole core. Treatment of the diketopiperazine 74 with PhI(OAc)2 led to the formation of two diastereomeric products 75 and 76 bearing the hexacyclic core structure of azonazine in 16 and 12% yields, respectively (Scheme 22). The poor yields of the cyclized products suggested that there was severe ring strain in the congested hexacyclic core, and that this strain was making it particularly difficult for cyclization to take place in a rapid and efficient manner. Subsequent hydrolysis of ketal 75 followed by reduction with NaBH4 afforded the benzofuro[2,3-b]indole 77, which was then converted to originally proposed structure of (+)-azonazine. This structure, however, was later revealed to be a diastereomer of natural (+)-azonazine. The total synthesis of (–)-azonazine was also accomplished in the same way using 76.
In studies toward the total synthesis of phalarine using biomimetic coupling strategies, Danishefsky et al.25 reported the intermolecular oxidative cycloaddition of the tetrahydro-β-carboline with phenol. Phenol 78 was treated with PhI(OCOCF3)2 followed by addition of tetrahydro-β-carboline 79 to give the benzofuro[2,3-b]indole 80 (Scheme 23).
Nicolaou and Chen26,27 achieved the total synthesis of (+)-haplophytine via an intermolecular oxidative cycloaddition reaction. Treatment of a mixture of dihydroxyindoline 81 and tetrahydro-β-carboline 82 with PhI(OCOCF3)2 provided the benzofuro[2,3-b]indole 83 in 23% yield (Scheme 24).
Although the hypervalent iodine-mediated oxidative cycloaddition reaction provides rapid and efficient access to benzofuro[2,3-b]indoles from phenols and indoles, the overall utility of this method has been limited by low yields. Based on these limitations, Chen’s group28 reported the development of an improved strategy for the synthesis of benzofuro[2,3-b]indoles via a 1,4-addition/cyclization reaction in their second-generation formal synthesis of (+)-haplophytine. In this study, the authors began by conducting a model study involving the reaction of p-quinone 84 with β-carboline 85 in the presence of CF3SO3H, which gave the benzofuro[2,3-b]indoles 86 and 87 as a 3:1 mixture of diastereomers (Scheme 25).
The preferential formation of 86 was attributed to a transition state where the steric interaction between 84 and the methyl propanoate side chain of 85 was minimized.
The 1,4-addition/cyclization reactions of several other functionalized p-quinone were also investigated in terms of their application to the synthesis of haplophytine (Scheme 26). When the p-quinones 88 and 89 were reacted with β-carboline 90, the 1,4-addition proceeded at the 5-position of the quinone ring to afford the benzofuro[2,3-b]indoles 91 and 92 in 56 and 44% yields, respectively. In contrast, when p-quinone 93 was used as the substrate, the addition reaction proceeded at the 6-position of the quinone ring to give benzofuro[2,3-b]indole 94 in 75% yield. Furthermore, the reaction of 3-amino-2-methoxy-p-quinone 93 with β-carboline 95 furnished benzofuro[2,3-b]indole 96 in 75% yield as a single stereoisomer, which proved to be an enabling intermediate in the formal synthesis of (+)-haplophytine.
MacMillan et al.29 developed a chiral imidazolidinone-catalyzed enantioselective alkylation-cyclization cascade reaction for the asymmetric synthesis of a benzofuro[2,3-b]indoles. The reaction of 3-(2-hydroxyphenyl)indole 97 with acrolein 98 in the presence of the imidazolidinone catalyst 99•TFA (20 mol%) gave the benzofuro[2,3-b]indole 101 in 90% yield with 82% ee (Scheme 27). The authors suggested that the addition of indole 97 to the activated iminium ion resulting from the reaction of catalyst 99 with acrolein 98 would lead to the formation of the C(3)-quaternary carbon-substituted indolenium ion 100, which would be trapped through an intramolecular reaction with the phenol moiety to afford the benzofuro[2,3-b]indole 101.
This cascade reaction was subsequently applied as one of the key transformations in the total synthesis of diazonamide A.30 Indole 102 was reacted with an excess of propynal in the presence of the imidazolidinone catalyst 103•TCA (30 mol%) in a ternary solvent mixture consisting of toluene, chloroform and methanol to give the benzofuro[2,3-b]indole core 104 of diazonamide A (Scheme 28).
Gouverneur and co-workers31 have developed a synthetic method for the construction of the fluorinated benzofuro[2,3-b]indole 108 using a catalytic asymmetric fluorocyclization reaction. The 3-(2-hydroxyphenyl)indole 107 was prepared in three-steps from 2-bromoanisole (105) and indole (106) (Scheme 29). The treatment of 107 with N-fluorobenzenesulfonimide and K2CO3 in the presence of a catalytic amount of (DHQ)2PHAL afforded 108 in 44% yield and 80% ee.
3.2. Synthesis from oxindole
In studies towards the first and second total syntheses of diazonamide A, Nicolaou’s group32-35 developed a synthetic approach to the benzofuro[2,3-b]indole structure of this natural product via the reductive cyclization of an oxindole. Oxindole 109 was selected as a model substrate for this reaction and treated with borane dimethyl sulfide to give the desired benzofuro[2,3-b]indole 111 via the formation of the indolenium intermediate 110 (Scheme 30).
The authors went on to apply this method to the total synthesis of diazonamide A. Thus, the treatment of 112 with DIBAL-H afforded benzofuro[2,3-b]indole 113 in 56% yield (Scheme 31).
Vedejs and Zajac36 reported the stepwise construction of benzofuro[2,3-b]indole 115 in excellent yield by the reduction of the N-alloc activated oxindole 114 with NaBH4 followed by the dehydration of the resulting hemiaminal with Ms2O and Et3N (Scheme 32).
Using similar conditions, Moody and co-workers37 reported the synthesis of the benzofuro[2,3-b]indoles 117a–c from the N-Boc or Alloc protected oxindoles 116a–c (Scheme 33).
This methodology was successfully applied to the synthesis of the benzofuro[2,3-b]indole core 119 of diazonamide A (Scheme 34).
Magnus and Turnbull38 attempted to apply this methodology to the reductive cyclization of oxindole 120, but their reactions proved to be unsuccessful because of over reduction to the indoline rather than the benzofuro[2,3-b]indole. In light of this failure, the authors went on to develop a mild and selective procedure from indoline-2-thione 121 prepared by thionation with Lawesson’s reagent. The indoline-2-thione 121a was treated with iodomethane to give 122a in 80% yield. Subsequent exposure of 122a to Raney nickel resulted in a clean desulfurization reaction to provide 123a in 82% yield (Scheme 35). The indoline-2-thione 121b was converted to 122b in a similar manner and then desulfurized to give 123b in 60% yield. The indoline-2-thiones 121a and 121b were also treated directly with Raney nickel to afford 123a and 123b in 72 and 63% yields, respectively.
3.3. Synthesis from indoline
Benzofuro[2,3-b]indoles can also be prepared by the oxidative cyclization of 3-(2-hydroxyphenyl)indolines. Nicolaou’s group35 reported that the oxidation of 3-(2-hydroxyphenyl)indoline 124 with MnO2 led to the formation of the benzofuro[2,3-b]indoles 125 and 126 for the synthesis of diazonamide A analogues (Scheme 36).
Bisai et al.39 reported the development of a similar process for the oxidative cyclization of 3-(2-hydroxyphenyl)indolines. Indoline 129 was prepared by the Lewis acid-catalyzed Friedel-Crafts alkylation of p-cresol (127) with 3-hydroxy-3-methyl-2-oxindole (128) followed by a reduction step. The subsequent treatment of indoline 129 with MnO2 afforded benzofuro[2,3-b]indole 133 in 72% yield. The authors proposed a mechanism for this transformation, which is shown in Scheme 37. α-Elimination reaction of the intermediate Mn(IV)-complex 130 under an oxidative environment would lead to the formation of indolenium intermediate 132 via the intermediacy of 131. The phenoxide 132 would then undergo an intramolecular reaction to afford the benzofuro[2,3-b]indole 133.
Vincent and co-workers40 reported that the regioselective C3-hydroarylation of a 3-substituted N-acetylindoles with phenols led to the formation of 3,3-disubstituted indolines, which could be oxidized to the corresponding benzofuro[2,3-b]indoles. The treatment of N-acetylindole 135 with para-substituted phenol 134 in the presence of iron(III) chloride afforded the indolines 136 in 24–99% yield (Scheme 38). Subsequent removal of the N-acetyl substituent of 136 under acidic conditions followed by oxidation of the resulting indoline using either tetrapropylammonium perruthenate (TPAP) or diisopropyl azodicarboxylate (DIAD) gave the benzofuro[2,3-b]indoles 137a–q in 50–86% yields.
To access enantioenriched benzofuro[2,3-b]indoles, N-mesyl-protected proline was employed as a chiral auxiliary and attached to the nitrogen atom of skatole (Scheme 39). The hydroarylation of 138 with p-cresol (127) afforded indolines 139a and 139b as a 2.8:1 mixture of C3-epimers. Hydrolysis of 139a followed by DIAD oxidation allowed for the isolation of (–)-137a in 94% ee.
4. CONCLUSION
We have described a variety of synthetic methods for the construction of benzofuro[2,3-b]pyrroles and benzofuro[2,3-b]indoles. Although effective synthetic approaches have been reported for the preparation of benzofuro[2,3-b]indoles, reports pertaining to the facile and efficient synthesis of benzofuro[2,3-b]pyrroles are relatively scarce. To study on the biological activity of benzofuro[2,3-b]pyrroles, the development of new methodologies for the construction of benzofuro[2,3-b]pyrrole skeleton will be further requested in the future.
ACKNOWLEDGEMENTS
This work was supported by Grants-in-Aid from the Ministry of Education, Culture, Sports, Science and Technology of Japan (MEXT), the MEXT-Supported Program for the Strategic Research Foundation at Private Universities, and Takeda Science Foundation.
References
1. (a) P. Ruiz-Sanchis, S. A. Savina, F. Albericio, and M. Álvarez, Chem. Eur. J., 2011, 17, 1388; CrossRef (b) M. A. Schmidt and M. Movassaghi, Synlett, 2008, 313; CrossRef (c) D. Crich and A. Banerjee, Acc. Chem. Res., 2007, 40, 151. CrossRef
2. J. Liu, T. Ng, Z. Rui, O. Ad, and W. Zhang, Angew. Chem. Int. Ed., 2014, 53, 136. CrossRef
3. A. W. Schammel, G. Chiou, and N. K. Garg, J. Org. Chem., 2012, 77, 725. CrossRef
4. M. Hayashi, M.-C. Rho, A. Enomoto, A. Fukami, Y.-P. Kim, Y. Kikuchi, T. Sunazuka, T. Hirose, K. Komiyama, and S. Omura, Proc. Natl. Acad. Sci. U.S.A., 2002, 99, 14728. CrossRef
5. S. Bräse, A. Encinas, J. Keck, and C. F. Nising, Chem. Rev., 2009, 109, 3903. CrossRef
6. (a) N. Lindquist, W. Fenical, G. D. Van Duyne, and J. Clardy, J. Am. Chem. Soc., 1991, 113, 2303; CrossRef (b) J. Li, A. W. G. Burgett, L. Esser, C. Amezcua, and P. G. Harran, Angew. Chem. Int. Ed., 2001, 40, 4770. CrossRef
7. Q.-X. Wu, M. S. Crews, M. Draskovic, J. Sohn, T. A. Johnson, K. Tenney, F. A. Valeriote, X.-J. Yao, L. F. Bjeldanes, and P. Crews, Org. Lett., 2010, 12, 4458. CrossRef
8. Although benzofuro[3,2-b]pyrroles and benzofuro[3,2-b]indoles are found in natural products and bioactive compounds, we will not discuss in this review. For selected example, see: (a) H. J. Kim, S. H. Cho, and S. Chang, Org. Lett., 2012, 14, 1424; CrossRef (b) H. Ding and D. Y.-K. Chen, Angew. Chem. Int. Ed., 2011, 50, 676; CrossRef (c) J. D. Trzupek, D. Lee, B. M. Crowley, V. M. Marathias, and S. J. Danishefsky, J. Am. Chem. Soc., 2010, 132, 8506; CrossRef (d) I. Kim, H.-K. Na, K. R. Kim, S. G. Kim, and G. H. Lee, Synlett, 2008, 2069.
9. T. Tsuritani, H. Shinokubo, and K. Oshima, Org. Lett., 2001, 3, 2709. CrossRef
10. T. Tsuritani, H. Shinokubo, and K. Oshima, J. Org. Chem., 2003, 68, 3246. CrossRef
11. Q. Wang, S. Nara, and A. Padwa, Org. Lett., 2005, 7, 839. CrossRef
12. A. Padwa, S. Nara, and Q. Wang, J. Org. Chem., 2005, 70, 8538. CrossRef
13. E. M. Beccalli, E. Borsini, G. Broggini, M. Rigamonti, and S. Sottocornola, Synlett, 2008, 1053.
14. J. Kurita, H. Sakai, S. Yamada, and T. Tsuchiya, J. Chem. Soc., Chem. Commun., 1987, 285. CrossRef
15. S. S. Soman and T. H. Thaker, J. Chem. Res., 2010, 502.
16. D. T. Connor and M. von Strandtmann, J. Org. Chem., 1973, 38, 3874. CrossRef
17. S. D. Joshi and R. N. Usgaonkar, Indian J. Chem., 21B, 1982, 399.
18. P. Waykole and R. N. Usgaonkar, Indian J. Chem., 21B, 1982, 707.
19. K. Harada, E. Kaji, K. Takahashi, and S. Zen, Chem. Pharm. Bull., 1994, 42, 1562. CrossRef
20. M. Ueda, Y. Ito, Y. Ichii, M. Kakiuchi, H. Shono, and O. Miyata, Chem. Eur. J., 2014, 20, 6763. CrossRef
21. J.-L. Avril, B. Marçot, M. Coquillay, C. De Rango, H. Moskowitz, and J. Mayrargue, New J. Chem., 1999, 23, 743. CrossRef
22. M. A. Tius and M. A. Kerr, J. Am. Chem. Soc., 1992, 114, 5959. CrossRef
23. A. W. G. Burgett, Q. Li, Q. Wei, and P. G. Harran, Angew. Chem. Int. Ed., 2003, 42, 4961. CrossRef
24. J.-C. Zhao, S.-M. Yu, Y. Liu, and Z.-J. Yao, Org. Lett., 2013, 15, 4300. CrossRef
25. C. Chan, C. Li, F. Zhang, and S. J. Danishefsky, Tetrahedron Lett., 2006, 47, 4839. CrossRef
26. K. C. Nicolaou, S. M. Dalby, S. Li, T. Suzuki, and D. Y.-K. Chen, Angew. Chem. Int. Ed., 2009, 48, 7616. CrossRef
27. K. C. Nicolaou, U. Majumder, S. P. Roche, and D. Y.-K. Chen, Angew. Chem. Int. Ed., 2007, 46, 4715. CrossRef
28. W. Tian, L. R. Chennamaneni, T. Suzuki, and D. Y.-K. Chen, Eur. J. Org. Chem., 2011, 1027. CrossRef
29. J. F. Austin, S.-G. Kim, C. J. Sinz, W.-J. Xiao, and D. W. C. MacMillan, Proc. Natl. Acad. Sci. U.S.A., 2004, 101, 5482. CrossRef
30. R. R. Knowles, J. Carpenter, S. B. Blakey, A. Kayano, I. K. Mangion, C. J. Sinz, and D. W. C. MacMillan, Chem. Sci., 2011, 2, 308. CrossRef
31. O. Lozano, G. Blessley, T. Martinez del Campo, A. L. Thompson, G. T. Giuffredi, M. Bettati, M. Walker, R. Borman, and V. Gouverneur, Angew. Chem. Int. Ed., 2011, 50, 8105. CrossRef
32. K. C. Nicolaou, M. Bella, D. Y.-K. Chen, X. Huang, T. Ling, and S. A. Snyder, Angew. Chem. Int. Ed., 2002, 41, 3495. CrossRef
33. K. C. Nicolaou, D. Y.-K. Chen, X. Huang, T. Ling, M. Bella, and S. A. Snyder, J. Am. Chem. Soc., 2004, 126, 12888. CrossRef
34. K. C. Nicolaou, P. B. Rao, J. Hao, M. V. Reddy, G. Rassias, X. Huang, D. Y.-K. Chen, and S. A. Snyder, Angew. Chem. Int. Ed., 2003, 42, 1753. CrossRef
35. K. C. Nicolaou, J. Hao, M. V. Reddy, P. B. Rao, G. Rassias, S. A. Snyder, X. Huang, D. Y.-K. Chen, W. E. Brenzovich, N. Giuseppone, P. Giannakakou, and A. O’Brate, J. Am. Chem. Soc., 2004, 126, 12897.
36. M. A. Zajac and E. Vedejs, Org. Lett., 2004, 6, 237. CrossRef
37. C. Poriel, M. Lachia, C. Wilson, J. R. Davies, and C. J. Moody, J. Org. Chem., 2007, 72, 2978. CrossRef
38. P. Magnus and R. Turnbull, Tetrahedron Lett., 2006, 47, 6461. CrossRef
39. S. Ghosh, L. K. Kinthada, S. Bhunia, and A. Bisai, Chem. Commun., 2012, 48, 10132. CrossRef
40. R. Beaud, R. Guillot, C. Kouklovsky, and G. Vincent, Angew. Chem. Int. Ed., 2012, 51, 12546. CrossRef