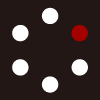
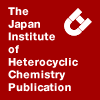
HETEROCYCLES
An International Journal for Reviews and Communications in Heterocyclic ChemistryWeb Edition ISSN: 1881-0942
Published online by The Japan Institute of Heterocyclic Chemistry
e-Journal
Full Text HTML
Received, 21st August, 2013, Accepted, 5th September, 2013, Published online, 13th September, 2013.
DOI: 10.3987/COM-12-12814
■ GYMNOCIN-A CARBOXYLIC ACID AND GYMNOCIN-A2, CYTOTOXIC POLYETHERS FROM THE RED TIDE DINOFLAGELLATE KARENIA MIKIMOTOI
Yoshihisa Tanaka, Masayuki Satake,* Mari Yotsu-Yamashita, and Yasukatsu Oshima
Department of Chemistry, Graduate School of Science, The University of Tokyo, 7-3-1 Hongo, Bunkyo-ku, Tokyo 113-0033, Japan
Abstract
Gymnocins are a series of cytotoxic polyether compounds isolated from the notorious red tide dinoflagellate Kareia (formerly Gymnodinium) mikimotoi. Among them, the structures of gymnocin-A and gymnocin-B have already been elucidated. Gymnocin-A carboxylic acid and a new analog of gymnocin-A, gymnocin-A2, were isolated from K. mikimotoi cells, and their structures were determined by detailed analysis of 2D-NMR spectra. The structural changes of gymnocin-A carboxylic acid and gymnocin-A2 occurred on the terminal side chain and ring A in gymnocin-A. Gymnocin-A carboxylic acid and gymnocin-A2 showed moderate cytotoxicity against P388 cells.INTRODUCTION
Red tides due to blooms of phytoplankton result in massive fish kills in the fields. Dinoflagellate Karenia (formerly Gymnodinium) mikimotoi is one of the most notorious red tide species, causing devastating damage to aquaculture and marine ecosystems worldwide, and the mechanism of its toxic effect on fish remains unknown.1,2 When a mixture of extract of K. mikimotoi was tested on a fresh water fish, Tanichthys albonubes, the toxicity was 250 times less potent than 42-dihydroBTXB (PbTx-3).3 Using a cytotoxicity assay instead of the elusive fish toxicity assay, we isolated new toxins, termed gymnocins. We successfully determined the relative stereostructures of gymnocin-A (1) and gymnocin-B (2) by the complementary use of nuclear magnetic resonance (NMR) and fast atom bombardment tandem mass spectrometry with collision-induced dissociation experiments,4,5 and their absolute configurations were elucidated by modified Mosher’s method for 1,4 and circular dichroism (CD) and fluorescence-detected CD exciton chirality method for 2.6 Their structures were characterized by saturated contiguous ether rings and a 2-methylbut-2-enal side chain (Figure 1). However, the arrangement and the number of ether rings in 1 and 2 differed from each other. Compound 1 possesses 14 contiguous ether rings (5/7/6/7/6/6/7/6/6/6/6/7/6/6) in the molecule, while 2 is constructed with 15 contiguous ether rings (5/7/6/6/6/6/7/7/6/7/6/6/6/6/7). The number of contiguous ether rings of 2 is larger than that of known polyether compounds: 11 for brevetoxin-B, 12 for ciguatoxin (CTX), 10 for maitotoxin, and 14 for C-CTX.7-9 In a recent study, brevisulcenal-F was isolated from K. brevisulcata and had 17 contiguous ether rings.10 Although the structural backbones of 1 and 2 were quite different, except for the terminal side chain, their cytotoxic potencies were very similar. The complex biosynthetic route to produce structurally and biologically diverse polyethers in dinoflagellates is intriguing. Total synthesis of 1 was already accomplished,11 and that of 2 was preceded by Sasaki and co-workers.12 Thus, structures of gymnocins are fascinating for not only natural product chemists but also synthetic chemists. In our continued efforts to clarify other congeners, we successfully isolated gymnocin-A carboxylic acid (3), which was already synthesized,13 and a new analog of gymnocin-A, gymnocin-A2 (4). In this paper, we report isolation and structures of 3 and 4 (Figure 1).
RESULTS AND DISCUSSION
Isolation of Gymnocin-A Carboxylic Acid and Gymnocin-A2
Compounds 3 and 4 were eluted at 25 and 50 min, respectively, on a Develosil C30 UG-5 column with 25% PrOH. From 110 L of cultured cells, 0.3 mg of 3 and 0.4 mg of 4 were obtained together with 1.1 mg of 1 and 0.6 mg of 2.
Structure of Gymnocin-A Carboxylic Acid
Compound 3 was obtained as a white amorphous solid. The UV absorption maximum was observed at 205 nm (ε 9300). The high-resolution fast atom bombardment mass spectrum (HR-FAB MS) indicated that the molecular formula of 3 was C55H80O19 ([M+Na]+ 1067.5193, Δ +0.1 mmu). Thus, compound 3 was indicated to be 16 mass units larger than 1. It was suggested that the aldehyde in 1 was oxidized to carboxylic acid in 3 or that 3 has an extra hydroxyl group compared with the structure of 1. In the 1H NMR spectrum of 3, the conjugated aldehyde signal of 1 was not observed and an olefinic methine at H3 shifted 0.34 ppm to a lower field (Figure 2). Thus, UV, MS, and NMR spectra suggested that the terminal aldehyde in 1 was oxidized to carboxylic acid in 3. The small amount of 3 hampered the measurement of the 13C NMR spectrum. The long-range correlation from Me-53 to a carbonyl carbon at 169 ppm was observed in the HMBC spectrum. In the 1H–1H COSY, an allylic long-range coupling from Me-53 to H3 and the proton connectivity from H3 to H5 were observed as is the case with 1, and the chemical shifts from H5 to Me-52 of 3 were identical with those of 1 (Table 1). Therefore, 3 has the identical backbone structure as 1 and the terminal aldehyde oxidized to carboxylic acid. Compound 3 was determined to be gymnocin-A carboxylic acid, which was already synthesized (Figure 1).13 1H NMR and 1H–1H COSY spectra of 3 were completely consistent with those of synthesized gymnocin-A carboxylic acid by Tsukano and Sasaki.14
Structure of Gymnocin-A2
Compound 4 was obtained as a white powder. The UV absorption maximum was observed at 278 nm (ε 12200). The UV maximum of 4 shifted to a wavelength around 40 nm longer than that of 1. The HR-FAB MS indicated that the molecular formula of 4 was C55H80O18 ([M+H]+ 1029.5424, Δ +0.1 mmu). The molecular formula of 4 was identical with that of 1; however, NMR spectra of 4 slightly differed from those of 1. In the 1H NMR spectrum of 4, two conjugated olefinic methines were newly observed, and a conjugated aldehyde, an olefinic methine, and a methyl on a double bond were observed analogous with 1 (Figure 2). Thus, 4 had one aldehyde and two double bonds in the molecule. Considering the unsaturation number (16), 4 possessed 13 contiguous ether rings in the molecule. The above results indicated that one of the ether rings in 1 opens in 4. Methylene protons at H4 and an oxymethine proton at H5 of 1 were not observed in the 1H NMR spectrum of 4. Therefore, UV, MS, and NMR spectra suggested that structural changes in 4 occurred at the terminal side chain and that ring A was lacking. The small amount of 4 hampered the measurement of the 13C NMR spectrum. In the 1H–1H COSY, a long-range coupling from Me-53 to H3 was observed as is the case with 1, and the proton couplings from H3 to H5 were assigned. Thus, a new double bond C4=C5 was connected with the double bond C2=C3 in a conjugated system. The geometries of the C2=C3 and C4=C5 double bonds were determined as E based on 3JH4,H5 = 15.0 Hz and NOE correlations of H1/H3 and Me-53/H4. The proton couplings from H5 to H23 were assigned by the detailed analysis of the 1H–1H COSY. The connectivity and chemical shifts of other signals belonging to a skeletal structure from H25 to H2-39 and from H41 to Me-52 including angular methyls on C24 and C40 in 4 were identical with those in 1 (Table 1). The observed NOE correlation of H11/H15 and 1H chemical shifts of angular protons confirmed ether ring C. The chemical shifts from H17 to Me-52 of 4 were identical with those of 1 (Table 1) and confirming ether rings D–N. The unsaturation number suggested existence of another ether ring except for rings C–N. It was considered that another ether oxygen was attached at C7 and C12 (oxepane ring), at C8 and C12 (tetrahydropyran ring), or at C7 and C10 (tetrahydrofuran ring). Although close chemical shifts of H7 and H12 hampered the assignment of the NOE correlation, an NOE of H8/H11 and chemical shifts of H2-9, which were similar to that of H2-16 on oxepane ring D, confirmed existence of oxepane ring B and a 2-methylhexa-2,4-dienal side chain at C7. An NOE correlation of H8/H11 indicated α orientation of 8-OH. The stereostructure of 10-OH was determined by coupling constants and NOEs analogous with 1. A small coupling constant between H10 and H11, and NOEs of H8/H9β, H8/H11, H9α/H10, H9β/H10, H9β/H11, and H10/H11 indicated pseudo axial disposition for 10-OH on oxepane ring B. Therefore, the structure of gymnocin-A2 was shown as in 4 (Figure 1). Gymnocin-A2 has 13 saturated ether rings and a 2-methylhexa-2,4-dienal side chain.
Cytotoxicity of Gymnocin-A Carboxylic Acid and Gymnocin-A2
Compounds 3 and 4 showed cytotoxicity against mouse lymphoide P388 cells at 3.4 and 1.4 µg/mL, respectively (Table 2). Interestingly, their potency was very close to those of 1 (1.3 µg/mL) and 2 (1.7 µg/mL). It has been previously reported that the cytotoxicity of 3 was >10 µg/mL, which is more than three times that in this study.13 It was suggested that the difference in the cytotoxicity of 3 is attributable to the lot-to-lot variation of P388 cells used in the cytotoxic test. Cytotoxicity of synthetic allyl alcohol analog (5) prepared by Sasaki et al. markedly decreased more than 100 times.15 The cytotoxicity of 10,17,50-triacetylgymnocin-A (6), prepared by the chemical reaction using acetic anhydride, was more than 20 times less potent than that of 1. Therefore, terminal aldehyde and ring A seem nonessential, but a terminal electrophilic conjugated system and hydroxyl groups on the ether rings are essential for gymnocin activity. Although these toxins may have shown relatively weak activity compared with other cytotoxic natural marine products isolated from dinoflagellates, K. mikimotoi produces much more potent congeners.16,17 Structural elucidation of other congeners will ultimately elucidate the mechanism of the massive fish kills and cytotoxicity of gymnocins.
EXPERIMENTAL
Chemicals and Instruments
All solvents for purification were purchased from Wako Pure Chemical Industries. 1-Propanol (PrOH) was used after distillation. UV spectra were obtained with a Shimadzu UV-400 spectrometer. The HR-FAB mass spectra were measured with a JEOL JMS 700 spectrometer. NMR spectra were recorded on a Varian Unity INOVA 600 spectrometer (1H at 600 MHz) at 20 °C in CDCl3.
Laboratory Culture of Kareia mikimotoi
Dinoflagellate K. mikimotoi was collected at Kushimoto Bay, Wakayama, Japan. It was cultured in 3 L Fernbach flasks each containing two L of seawater media enriched with T1 nutrients for 28 days at 25 °C.
Isolation of Gymnocins
K. mikimotoi cells were harvested by centrifugation, defatted with acetone–hexane (1:9), and extracted three times with 80% PrOH. After evaporating PrOH, the residue was partitioned between hexane and 80% MeOH. The 80% MeOH fraction was evaporated, and the residue redissolved in 40% MeOH was extracted with CHCl3. The toxins obtained in the CHCl3 phase were chromatographed on DEAE cellulose (2.0 cm i.d. × 50 cm, Wako Pure Chemical Industries, Japan) with CHCl3, MeOH, and CHCl3–AcOH (3:1) in this order. The CHCl3 fraction was evaporated and redissolved in 50% tetrahydrofuran (THF) and chromatographed on a Develosil Lop ODS column (2.2 cm i.d. × 22 cm, Nomura Chemical, Japan) with 50% and 80% THF in this order. The toxins in the 50% THF fraction were chromatographed on a JAIGEL W 251 column (2.0 cm i.d. × 50 cm, Nihon Bunseki Kogyo, Japan) with 80% PrOH and then on a Develosil ODS UG-5 column (10 mm i.d. × 250 mm, Nomura Chemical, Japan) with 50% THF. Finally, 3 and 4 were purified on a Develosil C30 UG-5 column (10 mm i.d. × 250 mm, Nomura Chemical, Japan) with 25% PrOH. Throughout the purification, elution of gymnocins was monitored with a Shimadzu SPD-M10A VP photodiode array detector and by a cytotoxic test.
Cytotoxic Test
Cytotoxicity was tested by the XTT method.18 Mouse lymphoid P388 D1 cells were cultured in RPMI media containing 9.4 g/L RPMI (ICN, France), 2.0 g/L NaHCO3 (Wako Pure Chemical Industries, Japan), 10% fatal bovine serum (ICN, France), 100 units/mL penicillin G (Sigma-Aldrich, Japan), and 100 µg/mL streptomycin (Sigma-Aldrich, Japan). These cells (200 µL) were seeded into 96-well microplates at 200,000 cells/well and incubated with 5% CO2 at 37 °C for 20 h. During incubation, the cells were treated with each concentration from 0.02 to 10.0 µg/mL of gymnocins. The control cells were treated with 80% PrOH, which was a vehicle of gymnocins. A 50 µL XTT solution containing 50 g/L XTT sodium salt (Sigma-Aldrich, Japan) and 1.53 g/L phenazine methosulfate (Sigma-Aldrich, Japan) was added and incubated for another 4 h. Cytotoxicity was examined by measuring optical density at 490 nm of triplicate experiments. The values were calculated as the percentage of the control cells treated with 80% PrOH. Concentrations equal to killing 50% of the cells were calculated.
ACKNOWLEDGEMENTS
We are grateful to Prof. C. Tsukano of Kyoto University and Prof. M. Sasaki of Tohoku University for providing NMR data of synthetic gymnosin-A carboxylic acid, and Ms. M. Abe of Tohoku University for culturing K. mikimotoi. This study was supported by JSPS KAKENHI (No. 22404006).
References
1. T. Honjo, Rev. Fish Sci., 1994, 2, 225. CrossRef
2. I. R. Jenkinson and G. Arzul, 'Harmful Algae,' B. Reguera, J. Blanco, M. L. Fernandez, and T. Wyatt, ed. by Xunta de Galicia and IOC of UNESCO France, 1998; pp. 425-428.
3. T. Igarashi, S. Aritake, and T. Yasumoto, Nat. Toxins, 1999, 7, 71. CrossRef
4. M. Satake, M. Shoji, Y. Oshima, H. Naoki, and T. Yasumoto, Tetrahedron Lett., 2002, 43, 5829. CrossRef
5. M. Satake, Y. Tanaka, Y. Ishikura, Y. Oshima, H. Naoki, and T. Yasumoto, Tetrahedron Lett., 2005, 46, 3537. CrossRef
6. K. Tanaka, Y. Itagaki, M. Satake, H. Naoki, T. Yasumoto, K. Nakanishi, and Berova, N. J. Am. Chem. Soc., 2005, 127, 9561. CrossRef
7. a) Y. Y. Lin, M. Risk, S. M. Ray, D. Van Engen, J. Clardy, J. Golik, J. C. James, and K. Nakanishi, J. Am. Chem. Soc., 1981, 103, 6773; CrossRef b) Y. Shimizu, H. N. Chou, H. Bando, G. Duyne, and J. Clardy, J. Am. Chem. Soc., 1986, 108, 514; CrossRef c) D. G. Baden, FASEB J., 1989, 3, 1807.
8. T. Yasumoto and M. Murata, Chem. Rev., 1993, 93, 1897. CrossRef
9. R. J. Lewis, J. Am. Chem. Soc., 1998, 120, 5914. CrossRef
10. Y. Hamamoto, K. Tachibana, P. T. Holland, F. Shi, V. Beuzenberg, Y. Itoh, and M. Satake, J. Am. Chem. Soc., 2012, 134, 4963. CrossRef
11. C. Tsukano and M. Sasaki, J. Am. Chem. Soc., 2003, 125, 14294. CrossRef
12. C. Tsukano and M. Sasaki, Tetrahedron Lett., 2005, 46, 4617. CrossRef
13. C. Tsukano and M. Sasaki, Tetrahedron Lett., 2006, 47, 6803. CrossRef
14. C. Tsukano, Ph.D. Thesis, Tohoku University, 2006, Total synthesis and structure-activity relationship of gymnocin-A, a cytotoxic polycyclic ether.
15. C. Tsukano, M. Ebine, and M. Sasaki, J. Am. Chem. Soc., 2005, 127, 4326. CrossRef
16. J. Kobayashi and M. Tsuda, Nat. Prod. Rep., 2004, 21, 77. CrossRef
17. M. Konishi, X. Yang, B. Li, C. R. Fairchild, and Y. Shimizu, J. Nat. Prod., 2004, 67, 1309. CrossRef
18. D. A. Scudiero, R. H. Shoemaker, K. D. Paull, A. Monks, S. Tierney, T. H. Nofziger, M. J. Currens, D. Seniff, and M. R. Boyd, Cancer Res., 1988, 48, 4827