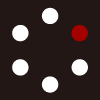
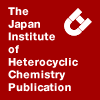
HETEROCYCLES
An International Journal for Reviews and Communications in Heterocyclic ChemistryWeb Edition ISSN: 1881-0942
Published online by The Japan Institute of Heterocyclic Chemistry
e-Journal
Full Text HTML
Received, 21st August, 2013, Accepted, 28th August, 2013, Published online, 12th September, 2013.
DOI: 10.3987/COM-13-12815
■ Simple and Stereocontrolled Preparation of Benzoylated Phenylalanine Using Friedel–Crafts Reaction in Trifluoromethanesulfonic Acid for Photoaffinity Labeling
Yuta Murai, Lei Wang, Yasuyuki Muto, Yasuko Sakihama, Yasuyuki Hashidoko, Yasumaru Hatanaka, and Makoto Hashimoto*
Division of Applied Science, Graduate School of Agriculture, Hokkaido University, Kita 9 Nishi 9, Kita-ku, Sapporo, Hokkaido 060-8589, Japan
Abstract
Simple and stereocontrolled preparation of benzoylated phenylalanine derivatives from optically pure phenylalanine using Friedel–Crafts reaction in trifluoromethanesulfonic acid (TfOH) is reported; these derivatives are useful for photoaffinity labeling. Protected or unprotected phenylalanine derivatives converted to benzoyl derivatives in TfOH at room temperature in a short time without loss of optical purity. The reaction condition was applied to synthesize novel photoreactive phenylalanine derivative, which has two photophores (benzophenone and diazirine). The detail analysis of photo-irradiation for two different photophores contained phenylalanine derivative was also investigated.Photoaffinity labeling is a valuable chemical method for studying the interactions of biologically active molecules with their target proteins. In photoaffinity labeling, a covalent bond is formed between ligand and target proteins upon irradiation with UV light.1 For instance, to study biologically active peptide interactions for a target protein, a photoreactive α-amino acid will be required and is a powerful probe for photoaffinity labeling. It has been reported for the preparation of benzophenone contained α-amino acid derivatives, which were synthesized from halomethyl derivatives of benzophenone and α-amino- malonate equivalents using racemic2 or asymmetric3 methods (Figure 1: A). But there are no reports on direct construction of benzophenone moiety on phenylalanine with Friedel–Crafts reaction due to low solubility of phenylalanine derivatives in appropriate solvent for the reaction. Here, we report a useful strategy for direct construction of benzophenone moiety on optically pure phenylalanine using a Friedel–Crafts reaction in trifluoromethanesulfonic acid (TfOH) with stereocontrolled manner (Figure 1: B).
We have recently reported that TfOH can effectively dissolve α-amino acid derivatives and catalyze Friedel-Crafts reaction.4 We attempted direct construction of benzoylated phenylalanine by using TfOH (Scheme 1). Optically pure unprotected phenylalanine (L-1 or D-1) was dissolved in TfOH at 0 °C and stirred for 1 h, followed by the addition of benzoyl chloride (5, 8 eq). The reaction mixture was stirred at room temperature. Although the starting material was consumed completely in 12 h, the reaction became a complex mixture. The isolated 4-benzoylphenylalanine (6) was less than 30 % yield without loss of optical purity (Table 1, Entries 1-2). No improvement was observed, when the reaction mixture was heated (Table 1, Entries 3-4). Next, we considered to prevent the acid-base reaction between amino group and TfOH. Optically pure N-Ac-L-Phe (L-2) was subjected to benzoylation at room temperature, 60 oC and 80 oC. Although chemical yield was improved using L-2, the product (7) was loss of its optical activity (Table 1, Entries 5-7). Optically pure N-Ac-L-Phe-OMe (L-3) was subject to Friedel-Crafts reaction at room temperature. Consumption of L-3 and construction of benzophenone were observed within 1 h. But the α-amino acid skeleton was converted to oxazole derivative (9) between acetamide and methyl ester (Table 1, Entry 8). And we observed that L-3 did not form an oxazole derivative 9 without benzoyl chloride 5 under the same conditions. These results indicated that oxazole formation was promoted by in situ formation of mixed anhydride from benzoyl chloride and TfOH then the mixed anhydride reacted with 3. It is consistent with the formation of oxazoles from N-acylamino acid methyl esters in the presence of triflic anhydride.5 To prevent oxazole formation, we changed the protecting group for amino moiety from acetamide to trifluoroacetamide: the trifluoromethyl group is highly electron-withdrawing and sterically-bulky to prevent imino-ester formation. N-TFA-L-Phe-OMe (L-4) was treated at room temperature and completely consumed for 10 h. The yield of desired product L-8 improved by using compound L-4 without loss of optical purity (Table 1, Entry 9). The same treatment of D-4 also afforded D-8 without loss of optical purity (Table 1, Entry 10).
(Trifluoromethyl)phenyldiazirine (TPD) is one of the important photophores in photoaffinity labeling.6 Although TPD is expensive and sensitive to high temperature under strong acid condition,7 we have reported that TPD can be applied to the Friedel–Crafts reaction in TfOH at room temperature to synthesize TPD contained (bis) homophenylalanine derivatives.8 Although both benzophenone and TPD are excited at 350 nm, the reactive species generated from TPD was faster than that of benzophenone.9 But it has not been reported the construction of two photophores in one molecule and comprehensive analysis of photoreactive properties. We tried to construct two kinds of photophore (benzophenone and diazirine) on phenylalanine using our development synthesis. N-TFA-L-Phe-OMe (L-4) was treated with benzoyl chloride derivative (11)10 in TfOH at room temperature until L-4 was consumed completely. The desired product (L-12) was obtained in high yield without decomposition of diazirine moiety. Deprotection of L-12 proceeded with 1 M NaOH to yield compound L-13 (Scheme 2). The same treatment of N-TFA-D-Phe-OMe (D-4) afforded optically active D-13 as above without loss of optical purity.
We also confirmed the photoreactive properties of 13. We have already demonstrated that the concentration of the diazirinyl compound had to be set to less than 1 mM to minimize isomerization to the diazo compound.11 Methanolic solution of L-13 was irradiated with black light (100 W) for 10 min. The maximum absorption at 350 nm for diazirine moiety of L-13 decreased with time-dependent manner (Figure 2: A). The half-life of L-13 was determined to be 35 seconds. The photolyzed mixture was subjected to UPLC-TOF-MS. The major product afforded a molecular weight of m/z 382, which was consisted with methanol insertion to diazirine moiety and benzophenone is still remained (L-14a, Figure 2: B, I). One of the minor product afforded a molecular weight of m/z 414, which arose from the reaction of both photophores with methanol (L-15a, Figure 2: C, I). It was slightly difficult to predict detail structure of the reaction product in methanol from mass spectrometric analysis. Deuterated methanol (CD3OD) was subjected to photolysis. For major product, which was activated only TPD, 4 mass number increased, m/z 386 in CD3OD (Figure 2: B, II). The result indicated “D” and “OCD3” were incorporated to the diazirine moiety (L-14b). On the other hand, minor product, which was activated both TPD and benzophenone, afforded 6 mass number increased peak m/z 420 (L-15b).
These results indicated that benzophenone part increased two mass units. The photoirradiated structure was considered to be generated by the bond formation between carbonyl carbon of benzophenone and carbon of methanol (Figure 2C, II). Additionally we confirmed that methanolic or deuterated methanolic solution of benzophenone derivatives 6 did not decompose by photo-irradiation within 10 min. These results indicated that diazirine photophore substitutions at p-position of benzophenone promoted activation of benzophenone faster than unsubstituted one.
In summary, Friedel–Crafts reaction with TfOH as solvent and catalyst enabled us to employ simple and stereocontrolled benzoylation of optically active phenylalanine to prepare photoreactive phenylalanine derivatives. Additionally, this synthetic method was applicable to construction of two kinds of photophore (benzophenone and diazirine) in phenylalanine derivative. And photolysis study of 13 revealed that we could control the reactivity between TPD and benzophenone in the same molecule by photo-irradiation times. These simple preparations will be acceptable for all biochemist and contribute to understanding peptide-receptor interactions with photoaffinity labeling.
EXPERIMENTAL
1H, 13C and 19F NMR spectra were measured by JEOL ECA 500 and EX 270 spectrometers for structural determinations. ESI-TOF-MS spectra were measured with a Waters LCT Premier XE spectrometer.
General procedure for Friedel-Crafts reaction
TfOH (0.5 mL) was added to phenylalanine derivatives (0.1 mmol) at 0 °C in a tube with screw cap and PTFE-faced rubber liner. After solution became homogeneous, benzoyl chloride (3~8 equivalents) was added at 0 °C. The reaction mixture was stirred at indicated temperature until the stating material was consumed completely, then poured into cold water and AcOEt. The organic layer was washed with saturated aqueous NaCl, dried over MgSO4, and then filtrated. The filtrate was concentrated, and the residue was subjected to silica column chromatography (AcOEt/MeOH/H2O = 8/1/0.5 for unprotected Phe) to purify product.
4-(4-Benzoylbenzy)-5-methoxy-2-methyloxazole (9)
1H NMR (CDCl3) δ 7.77 (2H, d, J = 6.9 Hz), 7.73 (2H, d, J = 8.0 Hz), 7.57 (1H, t, J = 7.2 Hz), 7.46 (2H, t, J = 7.7 Hz), 7.36 (2H, d, J = 8.6 Hz), 3.88 (3H, s), 3.78 (2H, s), 2.33 (3H, s); 13C NMR (CDCl3) δ 196.50, 155.03, 152.49, 144.52, 137.79, 135.56, 132.24, 130.48, 129.99, 128.44, 128.20, 61.15, 30.87, 14.30; ESI-TOF-MS, M+H+ Calcd for C19H18NO3 308.1281, Found 308.1257.
(S)-Methyl 2-(2,2,2-trifluoroacetamido)-3-(4-(4-(3-(trifluoromethyl)-3H-diazirin-3-yl)benzoyl)-phenyl)propanoate (L-12)
TfOH (1 mL) was added to N-L-TFA-Phe-OMe (27.2 mg, 0.1 mmol) and 4-(3-(trifluoromethyl)-3H-diazirin-3-yl)benzoyl chloride (98.3 mg, 0.396 mmol) at 0 °C. The reaction mixture was warmed to room temperature and stirred until the stating material was consumed completely, then poured into cold water and AcOEt. The organic layer was washed with saturated aqueous NaCl, dried over MgSO4, and then evaporated. The crude product was purified by silica column chromatography (AcOEt/hexane = 1/8 to 1/3) to yield L-12 (37.5 mg, 77%) as colorless solid. 1H NMR (CDCl3) δ 7.80 (2H, d, J = 8.6 Hz), 7.74 (2H, d, J = 7.9 Hz), 7.30 (2H, d, J = 8.6 Hz), 7.22 (2H, d, J = 7.9 Hz), 6.87 (1H, brs, NH), 4.96 (1H, dd, J = 5.6, 5.9 Hz), 3.82 (3H, s), 3.37 (1H, dd, J = 14.2, 5.9 Hz), 3.24 (1H, dd, J = 14.2, 5.6 Hz); 13C NMR (CDCl3) δ 194.94, 170.10, 156.59 (q, 2JCF = 38.2 Hz), 140.09, 138.31, 136.13, 133.22, 130.50, 130.13, 129.35, 126.35, 121.86 (q, 1JCF = 275.4 Hz), 115.48 (q, 1JCF = 289.4 Hz), 53.31, 53.07, 37.22, 28.40 (q, 2JCF = 39.7 Hz); 19F NMR (CDCl3) δ -66.19; ESI-TOF-MS, M+H+ Calcd for C21H16F6N3O4 488.1032, Found 488.1022; [α]D +82.0 (c 1.0 CHCl3, 25 °C).
The same treatment of N-TFA-D-Phe-OMe (26.9 mg, 0.1 mmol) as described above gave D-12 (38 mg, 79%) as a colorless solid. [α]D -82.0 (c 1.0 CHCl3, 25 °C).
(S)-2-Amino-3-(4-(4-(3-(trifluoromethyl)-3H-diazirin-3-yl)benzoyl)phenyl)propanoic acid (L-13)
Compound L-12 (29.4 mg, 0.06 mmol) in MeCN (2.0 mL), MeOH (1.0 mL) and 1 M NaOH (1.0 mL) was stirred for 2 h at room temperature. The solution was evaporated, then the residue was purified by silica column chromatography (AcOEt/MeOH/H2O = 4/1/1) to yield L-13 (21.0 mg, 92%) as colorless solid. 1H NMR (CD3OD) δ 7.86 (2H, d, J = 8.6 Hz), 7.77 (2H, d, J = 7.9 Hz), 7.48 (2H, d, J = 7.9 Hz), 7.42 (2H, d, J = 8.6 Hz), 4.23 (1H, t, J = 6.9 Hz), 3.41 (1H, dd, J = 14.7, 6.9 Hz), 3.23 (1H, dd, J = 14.2, 6.9 Hz); 13C NMR (CD3OD) δ 196.66, 170.93, 141.30, 139.96, 137.61, 134.12, 131.67, 131.46, 130.86, 127.63, 123.41 (q, 1JCF = 274.3 Hz), 54.76, 37.17, 29.51 (q, 2JCF = 40.8 Hz); 19F NMR (CD3OD) δ -66.78; ESI-TOF-MS, M+H+ Calcd for C18H15F3N3O3 378.1066, Found 378.1052; [α]D -6.0 (c 0.2 MeOH, 25 °C).
The same treatment of compound D-12 (30.3 mg, 0.06 mmol) as described above gave D-13 (22.1 mg, 94%) as a colorless solid. [α]D +6.0 (c 0.2 MeOH, 25 °C).
Photolysis of compound 13 in methanol
A 1 mM solution of compound 13 in MeOH or CD3OD (1 mL) was placed in a quartz cuvette. After the sample atmosphere was replaced with nitrogen, photolysis was carried out with 100 W blacklight at a distance 1 cm from the surface of light source. Spectra were measured after each minute, and then the half-life was calculated from the decrease of the absorbance around 350 nm.
Determination of enantiomeric excess
Compound 6 and 8
1 mM MeOH solution of 6 was performed with Chirobiotic T (Astec), 4.6×250 mm, eluted with 10 % MeOH/H2O; flow rate, 1 mL/min.; UV detection at 210 nm. L-6; tR = 25.4 min., D-6; tR = 27.1 min.
For 8; After deprotection of 8 with 1 M NaOH, which was performed in the same manner as compound 6.
Compound 7
After methylation of 7 with thionyl chloride in MeOH at room temperature for 12 h, 1 mM MeOH solution of the compound was performed with CHIRALPAK AD (Daicel), eluted with hexane/iso-propanol 7/3; flow rate, 1 mL/min.; UV detection at 210 nm. L-7; tR = 10.8 min., D-7; tR = 7.8 min.
ACKNOWLEDGEMENTS
MH thanks the Fugaku Foundation for financial support. Part of this work was performed under the Cooperative Research Program of “Network Joint Research Center for Materials and Devices”
References
1. a) J. Brunner, Annu. Rev. Biochem., 1993, 62, 483; CrossRef b) F. Kotzyba-Hilbert, I. Kapfer, and M. Goeldner, Angew. Chem., Int. Ed. Engl., 1995, 34, 1296; CrossRef c) Y. Hatanaka, H. Nakayama, and Y. Kanaoka, Rev. Heteroatom Chem., 1996, 14, 213; d) G. Dorman and G. D. Prestwich, Trends Biotechnol., 2000, 18, 64; CrossRef e) Y. Hatanaka and Y. Sadakane, Curr. Top. Med. Chem., 2002, 2, 271. CrossRef
2. J. C. Kauer, S. EricksonViitanen, H. R. Wolfe, Jr., and W. F. DeGrado, J. Biol. Chem., 1986, 261, 10695.
3. G. Dorman, J. D. Olszewski, G. D. Prestwich, Y. Hong, and D. G. Ahern, J. Org. Chem., 1995, 60, 2292. CrossRef
4. a) R. Murashige, Y. Hayashi, and M. Hashimoto, Tetrahedron Lett., 2008, 49, 6566; CrossRef b) R. Murashige, Y. Hayashi, S. Ohmori, A. Torii, Y. Aizu, Y. Muto, Y. Murai, Y. Oda, and M. Hashimoto, Tetrahedron, 2011, 67, 641; CrossRef c) Y. Murai, Y. Hashidoko, and M. Hashimoto, Biosci. Biotechnol. Biochem., 2011, 75, 352. CrossRef
5. A. Thalhammer, J. Mecinović, and C. J. Schofield, Tetrahedron Lett., 2009, 50, 1045. CrossRef
6. R. A. G. Smith and J. R. Knowles, J. Am. Chem. Soc., 1973, 95, 5072. CrossRef
7. R. A. Moss, J.-M. Fede, and S. Yan, Org. Lett., 2001, 3, 2305. CrossRef
8. a) Y. Murai, Y. Hatanaka, Y. Kanaoka, and M. Hashimoto, Heterocycles, 2009, 79, 359; CrossRef b) R. Murashige, Y. Murai, Y. Hatanaka, and M. Hashimoto, Biosci. Biotechnol. Biochem., 2009, 73, 1377. CrossRef
9. M. Hashimoto and Y. Hatanaka, Eur. J. Org. Chem., 2008, 2513. CrossRef
10. A. Y. L. Shu and J. R. Heys, Tetrahedron Lett., 2000, 41, 9015. CrossRef
11. M. Hashimoto and Y. Hatanaka, Anal. Biochem., 2006, 348, 154. CrossRef