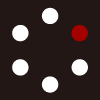
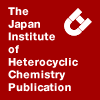
HETEROCYCLES
An International Journal for Reviews and Communications in Heterocyclic ChemistryWeb Edition ISSN: 1881-0942
Published online by The Japan Institute of Heterocyclic Chemistry
e-Journal
Full Text HTML
Received, 26th August, 2013, Accepted, 28th October, 2013, Published online, 8th November, 2013.
DOI: 10.3987/COM-13-12820
■ Design, Synthesis, and Biological Evaluation of 7H-Thiazolo[3,2-b]-1,2,4-triazin-7-one Derivatives as Dual Binding Site Acetylcholinesterase Inhibitors
Si-Jie Liu, Li-Bo Cui, Hiu-Lan Xu, Tie-Ying Wang, Chun Hu,* Shuo Li, Huang-Quan Lin, and David Chicheong Wan
Department of Organic Chemistry, Shenyang Pharmaceutical University, No. 103, Wenhua Road, Shenhe District, Shenyang 110016, China
Abstract
A series of 7H-thiazolo[3,2-b]-1,2,4-triazin-7-one derivatives were designed, synthesized and evaluated as dual binding site acetylcholinesterase inhibitors. The target compounds exhibited promising inhibitory activity for AChE. The structure-activity relationships were discussed and their binding conformation and simultaneous interactions mode were further clarified by the molecular docking studies.Alzheimer’s disease, a neurodegenerative disease affecting the elderly population throughout the world, is clinically characterized by an impairment of cognitive function.1 One of the current therapeutic strategies is to restore the brain acetylcholine level, which as the neurotransmitter is important for the regulation of the memory and the learning process.2 Recent evidence suggests that acetylcholinesterase (AChE) plays also a non-cholinergic role in the development of Alzheimer’s disease, as it works as a chaperone molecule, accelerating the amyloid beta (Aβ) peptide deposition, and the aggregation of Aβ into insoluble fibrils.3-,4 The inhibition of AChE could therefore improve Alzheimer’s disease symptoms. The crystallographic structure of AChE reveals that it possesses two separate ligand binding catalytic site (CAS) and peripheral anionic site (PAS). It implied that molecules that can interact with both binding sites of AChE could not only inhibit AChE, but prevent the aggregation of AChE with Aβ. Therefore, dual-site binding AChE inhibitors have been presented as a new therapeutic strategic option for Alzheimer’s disease in recent years.5
In our previous work, 3-aryl-6-arylmethyl-7H-thiazolo[3,2-b]-1,2,4-triazin-7-one derivatives were designed and synthesized, which exhibited inhibitory activity against AChE, and the molecular docking exhibited these derivatives interacted with the PAS and the CAS of AChE.6-8 In order to study the structure-AChE inhibitory activity relationships and the active sites of 7H-thiazolo[3,2-b]-1,2,4-triazin-7-one derivatives, a new series of 3-aryl-6-arylmethyl-7H-thiazolo[3,2-b]-1,2,4-triazin-7-one derivatives were designed and synthesized, and their inhibitory activity was determined.
Chemistry
The target 3-aryl-6-arylmethyl-7H-thiazolo[3,2-b]-1,2,4-triazin-7-one derivatives 6a-6h were obtained in satisfactory yields, and the synthetic pathway was described in Scheme 1. 4-(Arylmethylene)-2-methyl- 5(4H)-oxazolones (1) were cyclized by aromatic aldehydes and N-acetylglycine in acetic anhydride. 1 converted to intermediate 2-acetamido-3-aryl-2-propenoic acids (2) with acetone and water. 2-Acetamido-3-aryl-2-propenoic acids (2) were further hydrolyzed with 1N hydrochloric acid to provide arylpyruvic acids 3. 6-(Arylmethyl)-3,4-dihydro-3-thioxo-1,2,4-triazin-5(2H)-ones (4) were obtained under basic conditions (K2CO3) by the reaction of 3 and thiosemicarbazone in water. The cyclization of 4 with substituted phenacyl chlorides led to the 3-aryl-6-arylmethyl-7H-thiazolo[3,2-b]-1,2,4-triazin-7-one derivatives (5). The target compounds 6a-6h could be obtained with ordinary Williamson reaction. The chemical structures of all novel compounds synthesized herein were fully characterized by mass analysis, infrared spectra, and proton NMR spectroscopic data reported in the experimental section.
Inhibition of AChE
Table 1 illustrates the biological activity of target compounds against freshly prepared human AChE, in comparison to the huperzine-A. As shown in Table 1, the most target compounds showed good activities for AChE inhibition. The compounds (6a) which the substituents at the ortho position on the aryl ring at C3 position of parent nucleus led to a huge decrease in activity than that bearing the substituents at the para position (6b), indicating the steric hindrance could limit the access of the target compounds to the enzyme-binding site. While the compounds 6c, 6e and 6h with morpholinyl group led to a huge decrease in activity than that bearing other groups on the phenyl ring, which suggested that the morpholinyl group is not good group in the hydrophobic pocket of AChE. At best the mean activity with the IC50 values of the target compound 6g which possessed chloro groups reached to 0.49 μM.
These phenomena show that the steric, electronic effects and hydrophobic effect might play a significant role in influencing the biological activity of 3-aryl-6-arylmethyl-7H-thiazolo[3,2-b]-1,2,4-triazin-7-one derivatives.
Docking Studies
We also carried out docking simulations on the AChE-7H-thiazolo[3,2-b]-1,2,4-triazin-7-one complexes, with the aim to rationalize at molecular level interactions between the target molecules and AChE. Solution of the three-dimensional structure of Torpedo californica acetylcholinesterase (TcAChE) in 1991 opened up new horizons in research on an enzyme that had already been the subject of intensive investigation. One of the striking structural features of the AChE revealed from the X-ray analysis is the presence of a narrow, long, hydrophobic gorge which is approximately 20Å deep.9 AChE has a CAS (Ser200, His440 and Glu327), meanwhile, in AChE Phe295 and Phe297 residues are near the catalytic triad. In the middle of the gorge, there is an anionic binding site formed by Trp84 and Phe330.10 Further, peripheral anionic site (PAS) of AChE including Tyr70, Asp72, Tyr121, Glu278, Tyr334, and Trp279 residues existed at the gorge mouth.11 The structure of human AChE and Torpedo californica AChE was compared using the skeleton retrieved from the Protein Database (PDB code 1B41 and 1EVE). The conformational analyses were carried out by structure comparison tools MatchMaker and MatchAlign.12 Comparison result has revealed a high degree of similarity, especially with regard to the active sites. The most difference between human AChE and TcAChE in the binding site was that Phe330 of TcAChE was replaced by Tyr337 of human AChE, therefore Phe330 was mutated to Tyr337, which might reflect a real difference between human AChE and TcAChE. According to the compared result between human AChE and TcAChE, our molecular modeling studies were performed using human AChE.
Compound 6g, with strong inhibitory activity, exhibited multiple binding modes with AChE, which were in agreement with those of our previous derivatives. The 2-diethylaminoethoxy of 6g adopted an appropriate orientation for its binding to PAS, via a hydrogen bond interaction with Ser125 and the hydrophobic interaction with Tyr72, Tyr124, Trp286, and Tyr341. The conformation of the 4-chlorophenyl side chain could fit well with the shape of the gorge and occupy the large CAS of AChE, which made hydrophobic interaction with Ser203 and His447 as well as π-π stacking interaction with Tyr337. For compound 6c, the morpholine moiety occupies the PAS through hydrophobic interactions with Tyr72, Tyr124, Ser125, Trp286, and Tyr341. The thiazolo[3,2-b]-1,2,4-triazin-7-one ring can form hydrophobic interactions with Ser203 and His447. Meanwhile, the phenyl group can establish a π-π interaction with the aromatic ring of Trp86. But this preferred binding mode was different with that of 6a, which did not interact with CAS, and without strong interaction between with the residues of PAS was found except a π-π stacking interaction with Tyr341. These results may rationalize the huge decrease in activity of compound 6a for AChE.
EXPERIMENTAL
Chemistry
All reagents and solvents were purchased from common commercial suppliers and were used without further purification. All melting points were taken in open capillary tubes and the thermometer was uncorrected. The MS were obtained by electrospray (ESI) in a Waters spectrometer at 3.5 kV spray voltage, acetonitrile was used for solvent. The IR spectra were determined using a Bruker AFS55 spectrometer. 1H-NMRspectra were obtained in CDCl3 or DMSO-d6 on a Bruker spectrometers instrument operating at 300 MHz or 600 MHz.
4-(Arylmethylene)-2-methyl-5(4H)-oxazolones (1), 2-acetamido-3-aryl-2-propenoic acids (2), arylpyruvic acids (3), 6-(arylmethyl)-3,4-dihydro-3-thioxo-1,2,4-triazin-5(2H)-ones (4) and 6-arylmethyl-3-(hydroxyphenyl)-7H-thiazolo[3,2-b]-1,2,4-triazin-7-ones (5) were prepared by previously reported procedures.6-7
General procedure for the synthesis of 7H-thiazolo[3,2-b]-1,2,4-triazin-7-ones (6):
5 (10 mmol) was solved in acetone (20 mL), K2CO3 (2 g) and alkyl chloride (10 mmol) were added, and refluxed for 8-24 h until the TLC assay indicated that the reaction was completed. The mixture was filtered, and evaporated to remove solvents. The residues were collected and recrystallized from EtOH, to give the target compounds 6a-6h.
6-Benzyl-3-(2-[(2-diethylamino)ethoxy]phenyl)-7H-thiazolo[3,2-b]-1,2,4-triazin-7-one (6a): White crystals, 43.7% yield; mp 115-117 °C; 1H-NMR (600 MHz, DMSO-d6): δ 0.72 (6H, m), 2.27 (4H, m), 2.41 (2H, t, J = 4.8 Hz), 3.89 (2H, s), 3.91 (2H, t, J = 4.8 Hz), 7.03 (1H, m), 7.11 (1H, d, J = 8.4 Hz), 7.15-7.22 (5H, m), 7.40 (1H, s), 7.41 (1H, m), 7.50 (1H, d, J = 8.4 Hz); ESI-MS (m/z): 435.0 (M+H)+; IR (KBr): 3029, 2969, 2841, 1648, 1594, 1571, 1489, 1451, 1382, 1354, 1282, 1259, 1207, 1163, 1105, 1061, 1038, 1003, 951, 895, 861, 824, 770.
6-Benzyl-3-(4-[(2-diethylamino)ethoxy]phenyl)-7H-thiazolo[3,2-b]-1,2,4-triazin-7-one (6b): White crystals, 75.6 % yield; mp 176-178 °C; 1H-NMR (300 MHz, CDCl3): δ 1.11 (6H, m), 2.69 (4H, m), 2.93 (2H, t, J = 6.2 Hz), 4.10 (2H, t, J = 6.2 Hz), 4.12 (2H, s), 6.71 (1H, s), 6.92 (2H, d, J = 8.7 Hz), 7.25-7.36 (5H, m), 7.46 (2H, d, J = 8.7 Hz); ESI-MS (m/z): 435.0 (M+H)+; IR (KBr): υ 3062, 2967, 2801, 1632, 1576, 1560, 1489, 1413, 1385, 1354, 1309, 1291, 1257, 1173, 1124, 1032, 954, 803, 752, 701.
6-Benzyl-3-(4-[(4-[2-(4-morpholinyl)ethoxy]phenyl)-7H-thiazolo[3,2-b]-1,2,4-triazin-7-one (6c): White crystals, 38.9 % yield; mp 205-207 °C; 1H-NMR (300 MHz, DMSO-d6): δ 2.48 (4H, m), 2.71 (2H, t, J = 5.7 Hz), 3.59 (4H, m), 3.97 (2H, s), 4.15 (2H, t, J = 5.7 Hz), 6.98 (2H, d, J = 8.8 Hz), 7.24-7.33 (5H, m), 7.40 (1H, s), 7.57 (2H, d, J = 8.8 Hz); ESI-MS (m/z): 449.1 (M+H)+; IR (KBr): υ 3107, 2947, 2851, 2787, 1630, 1580, 1478, 1415, 1388, 1356, 1294, 1254, 1152, 1116, 1072, 1040, 954, 858 , 768, 755.
6-(4-Methoxybenzyl)-3-(4-[(2-diethylamino)-2-oxoethoxy]phenyl)-7H-thiazolo[3,2-b]-1,2,4-triazin-7-one (6d): White crystals, 81.3 % yield; mp 168-170 °C; 1H-NMR (600 MHz, CDCl3): δ 1.17 (3H, t, J = 7.0 Hz), 1.27 (3H, t, J = 7.4 Hz), 3.43 (4H, m), 3.79 (3H, s), 4.05 (2H, s), 4.78 (2H, s), 6.72 (1H, s), 6.84 (2H, d, J = 8.4 Hz), 7.00 (2H, d, J = 9.0 Hz), 7.26 (2H, d, J = 9.0 Hz),7.49 (2H, d, J = 8.4 Hz); ESI-MS (m/z): 478.9 (M+H)+; IR (KBr): υ 3103, 2974, 2934, 1631, 1581, 1480, 1384, 1360, 1302, 1249, 1177, 1032, 817, 756.
6-(4-Methoxybenzyl)-3-(4-[2-(4-morpholinyl)-2-oxoethoxy]phenyl)-7H-thiazolo[3,2-b]-1,2,4-triazin-7-one (6e): White crystals, 75.6 % yield; mp 176-178 °C; 1H-NMR (300 MHz, CDCl3): δ 3.67 (8H, br), 3.80 (3H, s), 4.05 (2H, s), 4.77 (2H, s), 6.72 (1H, s), 6.84 (2H, d, J = 8.7 Hz), 7.00 (2H, d, J = 8.7 Hz), 7.26 (2H, d, J = 8.7 Hz),7.50 (2H, d, J = 8.7 Hz); ESI-MS (m/z): 492.9 (M+H)+; IR (KBr): υ 3113, 2953, 2858, 1642, 1576, 1512, 1486, 1363 , 1246, 1212, 1182, 1064, 1026, 996, 921, 876, 815, 792.
6-(4-Chlorobenzyl)-3-(4-[(2-diethylamino)-2-oxoethoxy]phenyl)-7H-thiazolo[3,2-b]-1,2,4-triazin-7- one (6f): White crystals, 37.8 % yield; mp 198-201 °C; 1H-NMR (600 MHz, DMSO-d6): δ 1.05 (3H, t, J = 7.2Hz), 1.18 (3H, t, J = 7.2 Hz), 3.32 (4H, m), 3.98 (2H, s), 4.86 (2H, s), 6.91 (2H, d, J = 9.0 Hz), 7.32 (2H, d, J = 8.4 Hz), 7.37 (2H, d, J = 8.4 Hz), 7.42 (1H, s), 7.52 (2H, d, J = 9.0 Hz); ESI-MS (m/z): 483.0 (M+H)+; IR (KBr): υ 3088, 2918, 2849, 1628, 1577, 1508, 1478, 1384, 1310, 1255, 1187, 1094, 1028, 836, 809.
6-(4-Chlorobenzyl)-3-(4-[(2-diethylamino)ethoxy]phenyl)-7H-thiazolo[3,2-b]-1,2,4-triazin-7-one (6g): White crystals, 43.2% yield; mp 184-186 °C; 1H-NMR (600 MHz, DMSO-d6): δ 1.02 (6H, m), 2.57 (4H, m), 2.80 (2H, t, J = 6.0 Hz), 3.99 (2H, s), 4.07 (2H, t, J = 6.0 Hz), 6.93 (2H, d, J = 8.4 Hz), 7.32 (2H, d, J = 8.4 Hz), 7.38 (2H, d, J = 8.4 Hz), 7.41 (1H, s), 7.50 (2H, d, J = 8.4 Hz); ESI-MS (m/z): 469.0 (M+H)+; IR (KBr): υ 3077, 2968, 1632, 1577, 1508, 1485, 1415, 1385, 1354, 1293, 158, 1179, 1120 , 1043, 882, 807, 766.
6-(4-Chlorobenzyl)-3-(4-[2-(4-morpholinyl)ethoxy]phenyl)-7H-thiazolo[3,2-b]-1,2,4-triazin-7-one (6h): White crystals, 47.3% yield; mp 162-165 °C; 1H-NMR (600 MHz, DMSO-d6): δ 2.49 (4H, br), 2.73 (2H, t, J = 6.0 Hz), 3.59 (4H, m), 3.98 (2H, s), 4.15 (2H, t, J = 6.0 Hz), 6.95 (2H, d, J = 9.0 Hz), 7.31 (2H, d, J = 8.4 Hz), 7.38 (2H, d, J = 8.4 Hz), 7.41 (1H, s),7.51 (2H, d, J = 9.0 Hz); ESI-MS (m/z): 483.0 (M+H)+; IR (KBr): υ 3075, 2930, 2854, 1631, 1508, 1480, 1415, 1385 , 1294, 1255, 1179, 1117, 1046, 958, 808, 768.
Inhibition of AChE
The inhibitory potency against AChE was evaluated by means of an Ellman’s test.13 AChE stock solution was prepared by dissolving human AChE 0.5 unit in 100 mM PBS buffer (pH 7.4). Five different concentrations of each compound were used in order to obtain inhibition of AChE activity comprised between 20% and 90%. The assay solution consisted of 100 mM PBS buffer (pH 7.4), with the addition of 10 mM 5,5’-dithiobis(2-nitrobenzoic acid) (DTNB, Ellman’s reagent), AChE (5 μL), drug (10 μL), and 12.5 mM acetylthiocholine iodide water solution. The final assay volume was 900 μL. Incubate the reaction at 37 °C for 15 min with continuous gentle shaking. Add 50 mL acetylthiocholine iodide and 50 mL DTNB. Incubate at 37 °C for about 20 min with continuous gentle shake, wait until the yellow color developed and measure at 412 nm. The 50% inhibitory concentration (IC50) was calculated from a dose-response curve obtained by plotting the percentage of inhibition versus the log concentration.
Docking Studies
The docking program AUTODOCK 4.0 package was be used to study the binding mode of the target compounds with AChE.14 Discovery Studio (DS) Visualizer2.5 software were used for visualization protein-ligand interactions.15 Affinity grid files on the molecule target were generated using the auxiliary program AUTOGRID. The dimensions of the grid were 60 Å ×60 Å ×60 Å centered on the Trp86 and Trp286 for the CAS and PAS, respectively, with grid points separated by 0.375 Å. The flexible docking of the ligand structures was done by the Lamarckian genetic algorithm (LGA), searching for favourable bonding conformations of the ligands at the sites of the target protein. After docking, the 100 solutions were clustered in groups with rms deviations of less than 1.0 Å.
ACKNOWLEDGEMENTS
This work was supported by the National Natural Science Foundation of China (No. 21072130) and Program for Innovative Research Team of the Ministry of Education and Program for Liaoning Innovative Research Team in Universities.
References
1. A. Skrzypek, J. Matysiak, A. Niewiadomy, and M. Bajda, Eur. J. Med. Chem., 2013, 62, 311. CrossRef
2. I. Tomassoli, L. Ismaili, and M. Pudlo, Eur. J. Med. Chem., 2011, 46, 1. CrossRef
3. L. Pan, J. H. Tan, J. Q. Hou, S. L. Huang, L. Q. Gu, and Z. S. Huang, Bioorg. Med. Chem. Lett., 2008, 18, 3790. CrossRef
4. M. Bartolini, C. Bertucci, V. Cavrini, and V. Andrisano, Biochem. Pharmacol., 2003, 65, 407. CrossRef
5. R. Sheng, X. Lin, J. Zhang, K. S. Chol, W. H. Huang, B. Yang, Q. J. He, and Y. Z. Hua, Bioorg. Med. Chem., 2009, 17, 6692. CrossRef
6. S. J. Liu, L. Yang, X. G. Liu, D. C. C. Wan, H. Q. Lin, and C. Hu, Lett. Drug. Des. Discov., 2010, 7, 5. CrossRef
7. S. J. Liu, L. Yang, Z. Jin, E. F. Huang, D. C. C. Wan, H. Q. Lin, and C. Hu, ARKIVOC, 2009, x, 333. CrossRef
8. H. N. Xu, Z. Jin, S. J. Liu, H. M. Liu, D. C. C. Wan, H. Q. Lin, and C. Hu, Chin. Chem. Lett., 2012, 23, 765. CrossRef
9. P. Kapková, N. Stiefl, and U. Sürig, Arch. Pharm., 2003, 336, 523. CrossRef
10. X. C. He, F. Song, and Z. F. Wang, Bioorg. Med. Chem., 2007, 15, 1394. CrossRef
11. K. Asaad, U. H. Zaheer, and A. Shazia, Bioorg. Med. Chem., 2004, 12, 1995. CrossRef
12. E. F. Pettersen, T. D. Goddard, C. C. Huang, G. S. Couch, and D. M. Greenblatt, J. Comput. Chem., 2004, 25, 1605. CrossRef
13. P. Elsinghorst, C. Tanarro, and M. Gutschow, J. Med. Chem., 2006, 49, 7540. CrossRef
14. J. Q. Araújo, J. A. Lima, A. C. Pinto, R. B. Alencastro, and M. G. Albuquerque, J. Mol. Model., 2011, 17, 1401. CrossRef
15. N. Chitranshi, N. Chitranshi, P. K. Tripathi, and P. K. Seth, Med. Chem. Res., 2013, 22, 2328. CrossRef