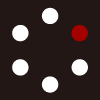
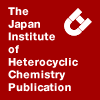
HETEROCYCLES
An International Journal for Reviews and Communications in Heterocyclic ChemistryWeb Edition ISSN: 1881-0942
Published online by The Japan Institute of Heterocyclic Chemistry
e-Journal
Full Text HTML
Received, 3rd September, 2013, Accepted, 15th October, 2013, Published online, 30th October, 2013.
DOI: 10.3987/COM-13-12828
■ SYNTHESIS OF CIS OR TRANS 4-HETEROAROMATIC SUBSTITUTEd FURANO AND PYRANO[3,2-C]TETRAHYDROQUINOLINES BY ONE-POT IMINO DIELS-ALDER REACTIONS
Rui Zhou, Wei Huang, Wen Ren, Li Guo,* Shi Zeng, Pan Liu, Liang Ouyang, Xiangrong Song, Cheng Peng, and Gu He*
West China Hospital, Sichuan University, No.17 3rd section, People's Road South 610041, China
Abstract
A mild and an efficient method for the synthesis of substitute furano and pyrano[3,2-c]tetrahydroquinoline derivatives was described, using the imino Diels-Alder reaction between 2-(4-aminophenyl)benzofuran, aromatic aldehyde, and cyclic enol ethers. Furthermore, 2-hydroxyalkyl-4-heteroaromatic substituted tetrahydrofurano[3,2-c]quinoline was synthesized with good yields through the same imino Diels-Alder reaction in the absence of aldehydes. The advantages of this procedure are mild reaction conditions, and good yields with high diastereoselectivity.In the latest years, numerous efforts have been directed towards the discovery of 2-(4-aminophenyl)benzothiazole because its analogues comprise a novel mechanistic class of antitumor agents.1 This nucleus is derived from related structures such as polyhydroxylated 2-phenylbenzothiazoles, flavone quercetin, and the isoflavone genistein, which are tyrosine kinase inhibitors with potent antitumor activity.2 Furthermore, some 2-phenylbenzofuran analogues have showed potent anticancer activities.
Meanwhile, it is a remarkable fact that tetrahydroquinoline moiety, as an important fragment of various natural products and pharmaceutical agents that have exhibited a broad range of biological characteristics.3 In addition, tetrahydroquinoline derivatives are used as potent pharmaceuticals,4 for example a group containing a tricyclic tetrahydroquinoline nucleus have been developed and investigated as the selective androgen receptor modulator or strong platelet inhibitors. Methods for tetrahydroquinoline derivative synthesis were developed, including the Lewis-acid catalyzed imino Diels–Alder reaction (Povarov reaction) of N-arylimines with dienophiles.5 Tricyclic compounds (furano or pyranoquinoline derivatives) were obtained when cyclic enol ethers such as 2,3-dihydrofuran or 3,4-dihydro-2H-pyran were employed as dienophiles.6
Although numerous methods for tetrahydroquinoline derivative synthesis have been reported in recent years, an efficient and highly diastereoselective method for the synthesis of novel tetrahydroquinoline scaffolds, an intriguing combination of two synthetically important pharmacophores, is still in demand.
To the best of our knowledge, the synthesis of 4-heteroaromatic substitute furano and pyrano[3,2-c]tetrahydroquinoline derivatives have never been reported. Such methodical variations may contribute to the bioactivity differences and enrich the tetrahydroquinoline library for biomedical screening. This report focuses on the synthesis of 4-heteroaromatic substitute furano and pyrano[3,2-c]tetrahydroquinoline derivatives through the reaction of aromatic aldehyde, 2-(4-aminophenyl)benzofuran, and 2,3-dihydrofuran (or 2,3-dihydropyran) catalyzed by indium chloride as a continuation of our previous research on new methods for the heterocyclic compound preparation by multi-component reactions.7
In this study, imino Diels-Alder reaction is a LUMO-diene controlled Diels-Alder reaction between an electron-deficient 2-azadiene (formed in situ from 7 with an aromatic aldehyde) and electron-rich dienophiles, such as cyclic enol ethers.6 This imino Diels-Alder reaction was first optimized using 2-(4-aminophenyl)benzofuran, benzaldehyde, and 3,4-dihydro-2H-furan (DHF). In the presence of 20 mol% indium chloride (InCl3) at room temperature, the corresponding 4-(2-benzofuranyl)furano[3,2-c]tetrahydroquinoline derivatives are derived with good yields. Obviously, these results are better than those reactions catalyzed by BF3, AlCl3, p-toluenesulfonic acid (p-TsOH), trifluoroacetic acid (TFA), or iodine using simple aromatic amines as reactants at similar conditions (Table 1, entries 1–4). Thereafter, the same imino Diels-Alder reaction of 2-(4-aminophenyl)benzofuran with benzaldehyde in the presence of 2,3-dihydrofuran (DHF) was performed, using InCl3 as catalyst and acetonitrile as solvent at different conditions (Table 1, entries 5–9). Notably, reactions run faster at room temperatures, and 20 mol% is the optimal catalyst amount.
Under optimized conditions, various aromatic aldehydes 8 are then subjected to react with 7 and 2,3-dihydrofuran (9a) to generate a library of 4-(2-benzofuranyl)furano[3,2-c]tetrahydroquinoline derivatives (Table 3). For aldehyde 8, the products were not sensitive to the aromatic ring’s electronic properties in the presence of electron-withdrawing groups (such as halide) or electron-donating groups (such as alkoxy group) (Table 2). The corresponding products were observed in the reaction system solution monitored by TLC.
Subsequently the product was separated by filtration before crystallization, and was also confirmed by 1H NMR as the isomer type.5,9 As expected, the 2,3-dihydrofuran substrates were extended to 3,4-dihydro-2H-pyran, which is also chosen to react with the aromatic aldehyde, 2-(4-aminophenyl) benzofuran, and were found to generate the corresponding 4-(2-benzofuranyl)pyrano[3,2-c]tetrahydro-quinoline derivatives (12a-i, 13a-i). The results are summarized in Table 3. The structure of 13f was also confirmed by COSY, HMBC, and NOE experiments.
In addition, the imino Diels-Alder procedure was performed without adding the aldehyde.6,8 The 2-alkoxy-4-heteroaromatic-substituted furano[3,2-c]tetrahydroquinolines 14 preparation is shown in Table 4. Using 2.0 equiv enol ether, DHF, and InCl3 catalyst provided good yields. In these reactions, only cis isomers were observed. Relative isomer configurations were obtained from 1H NMR spectral data, and assigned by comparison with NMR literature.8
In conclusion, a mild and efficient procedure for the synthesis of 4-heteroaromatic substituted furano and pyrano[3,2-c]tetrahydro-quinolines, using the one pot imino Diels-Alder approach was reported. Different catalysts and reaction conditions were examined, and the best results were obtained with InCl3 catalyst at room temperature. The isomer types (trans/cis) were assigned by 1H NMR spectra analysis. These tetrahydroquinoline derivatives were obtained in moderate to good yields with electron-withdrawing and electron-donating aromatic aldehydes. Without the addition of aldehyde, the imino Diels-Alder reaction using electron-rich olefins results in 2-alkoxy-4-heteroaromatic-substituted tetrahydroquinoline formation.
ACKNOWLEDGEMENTS
We gratefully acknowledge the supported by the National Natural Science Foundation of China (No. 81001357, 81273471 and 81303208) and the Open Research Fund of State Key Laboratory Breeding Base of Systematic Research, Development and Utilization of Chinese Medicine.
References
1. (a) R. Dubey, P. K. Shrivastava, P. K. Basniwal, S. Bhattacharya, and N. Moorthy, Mini-Rev. Med. Chem., 2006, 6, 633; CrossRef (b) E. Kashiyama, I. Hutchinson, M. S. Chua, S. F. Stinson, L. R. Phillips, G. Kaur, E. A. Sausville, T. D. Bradshaw, A. D. Westwell, and M. F. G. Stevens, J. Med. Chem., 1999, 42, 4172; CrossRef (c) T. D. Bradshaw, S. Wrigley, D. F. Shi, R. J. Schultz, K. D. Paull, and M. F. G. Stevens, Br. J. Cancer, 1998, 77, 745. CrossRef
2. (a) X. D. Yang, W. C. Wan, X. Y. Deng, Y. Li, L. J. Yang, L. Li, and H. B. Zhang, Bioorg. Med. Chem. Lett., 2012, 22, 2726; CrossRef (b) C. G. Fortuna, V. Barresi, G. Berellini, and G. Musumarra, Bioorg. Med. Chem., 2008, 16, 4150; CrossRef (c) F. P. Ballistreri, V. Barresi, P. Benedetti, G. Caltabiano, C. G. Fortuna, M. L. Longo, and G. Musumarra, Bioorg. Med. Chem., 2004, 12, 1689; CrossRef (d) M. F. G. Stevens, C. J. McCall, P. Lelieveld, P. Alexander, A. Richter, and D. E. Davies, J. Med. Chem., 1994, 37, 1689; CrossRef (e) P. C. Yates, C. J. McCall, and M. F. G. Stevens, Tetrahedron, 1991, 47, 6493. CrossRef
3. K. Faber, H. Stückler, and T. Kappe, J. Heterocycl. Chem., 1984, 21, 1177. CrossRef
4. (a) O. B. Wallace, K. S. Lauwers, S. A. Jones, and J. A. Dodge, Bioorg. Med. Chem. Lett., 2003, 13, 1907; CrossRef (b) H. Nejishima, N. Yamamoto, M. Suzuki, K. Furuya, N. Nagata, and S. Yamada, Prostate, 2012, 72, 1580; CrossRef (c) K. Furuya, N. Yamamoto, Y. Ohyabu, A. Makino, T. Morikyu, H. Ishige, K. Kuzutani, and Y. Endo, Biol. Pharm. Bull., 2012, 35, 1096; CrossRef (d) N. Nagata, M. Miyakawa, S. Amano, K. Furuya, N. Yamamoto, H. Nejishima, and K. Inoguchi, Bioorg. Med. Chem. Lett., 2011, 21, 6310. CrossRef
5. (a) E. Vicente-García, R. Ramon, and R. Lavilla, Synthesis, 2011, 14, 2237; CrossRef (b) E. Vicente-García, R. Ramon, S. Preciado, and R. Lavilla, Beilstein J. Org. Chem., 2011, 7, 980; CrossRef (c) B. H. Sacoman Torquato da Silva, L. M. Martins, and L. C. da Silva-Filho, Synlett, 2012, 1973; CrossRef (d) J. Li, S. C. Pei, Y. X. Zhu, J. B. Wu, Y. Chen, W. Y. Zhang, and Y. Wu, Lett. Drug Des. Discov., 2012, 9, 379; CrossRef (e) A. T. Khan, D. K. Das, K. Islam, and P. Das, Tetrahedron Lett., 2012, 53, 6418; CrossRef (f) R. Wang, and Z. Q. Liu, Med. Chem. Res., 2013, 22, 1563. CrossRef
6. (a) P. Buonora, J. C. Olsen, and T. Oh, Tetrahedron, 2001, 57, 6099; CrossRef (b) V. V. Kouznetsov, Tetrahedron, 2009, 65, 2721; CrossRef (c) D. Bello, R. Ramon, and R. Lavilla, Curr. Org. Chem., 2010, 14, 332; CrossRef (d) V. Sridharan, P. A. Suryavanshi, and J. C. Menéndez, Chem. Rev., 2011, 111, 7157. CrossRef
7. (a) G. Wu, L. Ouyang, J. Liu, S. Zeng, W. Huang, B. Han, F. Wu, G. He, and M. Xiang, Mol. Divers., 2013, 17, 271; CrossRef (b) X. Hou, H. Luo, H. Zhong, F. Wu, M. Zhou, W. Zhang, X. Han, G. Yan, M. Zhang, L. Lu, Z. Ding, G. He, and R. Li, Rapid Commun. Mass Spectr., 2013, 27, 1222; CrossRef (c) X. Xie, C. Peng, G. He, H. J. Leng, B. Wang, W. Huang, and B. Han, Chem. Commun., 2012, 48, 10487. CrossRef
8. F. Z. Yang, L. Y. Zheng, J. B. Xiang, Q. Dang, and X. Bai, J. Comb. Chem., 2010, 12, 476. CrossRef
9. The relative configuration of 10-13 was assigned by comparing the coupling constants for proton with other analogues, see references 13, 17 and 18, for more detailed information, see the supporting information.