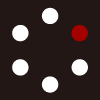
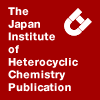
HETEROCYCLES
An International Journal for Reviews and Communications in Heterocyclic ChemistryWeb Edition ISSN: 1881-0942
Published online by The Japan Institute of Heterocyclic Chemistry
e-Journal
Full Text HTML
Received, 10th September, 2013, Accepted, 15th October, 2013, Published online, 21st October, 2013.
DOI: 10.3987/COM-13-12836
■ SYNTHESIS AND BIOLOGICAL EVALUATIONS OF A SERIES OF NOVEL AZOLYL, AZINYL, PYRANYL, CHROMONYL AND AZEPINYL PHOSPHONATES
Tarik E. Ali,* Salah A. Abdel-Aziz, Somaya M. El-Edfawy, El-Hossain A. Mohamed, and Somaia M. Abdel-Kariem
Department of Chemistry, Faculty of Education, Ain Shams University, Roxy, Cairo 11711, Egypt
Abstract
facile synthetic methodology of novel azolyl, azinyl and azepinyl phosphonates as cyclic α-aminophosphonates was described. Chromonyl α-aminophosphonates, γ-pyranyl and γ-pyridinyl phosphonates were also prepared. The methodology depends on reaction of 6-methyl-3-formylchromone, nitrogen and carbon nucleophiles in the presence of diethyl phosphite in one-pot three component under solvent-free conditions. The products were evaluated for their antimicrobial activities and antioxidant properties.INTRODUCTION
α-Aminophosphonic acid diesters, as phosphorus analogues of α-aminocarboxylic acids, are of great interest due to their reported biological activities.1 Some representatives of α-aminophosphonates have demonstrated promising enzyme inhibitory activities, as for example, HIV protease antagonists2 and collagenase inhibitor.3 Also, they have an important anticancer,4 antibacterial5 and antiviral activities.6 These biological properties are mostly associated with the tetrahedral structure of the phosphonyl group acting as ''a transition-state analogue''.7 In the last decades, intensive synthetic articles were performed in the preparation of α-aminophosphonic acids and their esters.8−13 The Kabachnik−Fields method is the most noteworthy and remarkable, generally using amines, dialkyl phosphites and carbonyl compounds.14,15 Although, a number of different methods have been reported for the preparation of acyclic α-aminophosphonates,16−19 there is still a need to search for new methods for the preparation of cyclic α-aminophosphonates which have found promising biological applications.20,21 As a part of our continuing interest in the preparation of acyclic and cyclic α-aminophosphonates,22−25 we describe a facile methodology to prepare some novel acyclic and cyclic α-aminophosphonates and also γ-heterocyclic phosphonates. The method is based on the reaction of 6-methyl-3-formylchromone with nitrogen and carbon mono- and bi-nucleophiles in the presence of diethyl phosphite in one-pot three-components under solvent-free conditions. The antimicrobial activities and antioxidant properties of the synthesized compounds were also evaluated.
RESULTS AND DISCUSSION
6-Methyl-3-formylchromone (1) was allowed to react with some aliphatic and aromatic amines as nitrogen mono-nucleophiles namely ethylamine, benzylamine, 4-chloroaniline and 4-hydroxyaniline in the presence of diethyl phosphite at 70−80 °C for 6 h under Kabachnik−Fields reaction conditions26,27 to produce the corresponding chromonyl α-aminophosphonates 2−5 in 62−100% yields (Scheme 1). The IR spectra of the α-aminophosphonates 2−5 showed the presence of NH (3289−3443 cm-1), C=Opyrone (1640−1646 cm-1) and P=O (1216−1225 cm-1) groups. Moreover, their 1H-NMR spectra recorded the characteristic ethoxy protons at the regions δ 0.93−1.29 ppm (CH3) and δ 3.60−4.00 ppm (CH2). The peaks of CH−P protons appeared as doublets at the regions δ 4.39−5.21 ppm with coupling constants in range 15−24 Hz. Furthermore, the protons H−2 of the chromone rings were displayed as singlets at the regions δ 8.20−8.95 ppm. The 13C-NMR spectral data of compounds 3 and 4 supported their structures due to the presence of the characteristic carbon atoms CH3, CH2, CH−P and C=Opyrone at the regions δ 16.0−16.5, 60.4−62.6, 45.2−45.5 (JPC = 148.5 and 158.5 Hz) and 174.6−174.8 ppm, respectively. Also, the 31P-NMR spectrum of the α-aminophosphonate 5 displayed a singlet at δ 21.6 ppm.
3-Formylchromones have attracted attention long ago as highly reactive compounds, which can serve as starting substances in the synthesis of a whole series of heterocycles. 3-Formylchromones have useful chemical properties due to presence of three strong electrophilic centers at C−2, C=Opyrone and formyl group.28,29 In the present article, the synthetic utility of 6-methyl-3-formylchromone (1) is derived from its reaction with nitrogen and carbon bi-nucleophiles that starts predominately from the attack on the formyl group to give the nonisolable condensation product A then pyrone ring opening at the unsubstituted C−2 forming the intermediate B. The latter intermediate B undergoes addition of diethyl phosphite at the cyclic azomethine bond to form the target phosphonates (route a, Scheme 2). Also, these phosphonates may be formed via addition of diethyl phosphite at the acyclic azomethine bond of the intermediate A leading to the formation of the nonisolable intermediate C, which undergoes pyrone ring opening (route b, Scheme 2).30 The resulted compounds gave characteristic red, green and blue colors with an alcoholic ferric chloride solution which support pyrone ring opening.
When 6-methyl-3-formylchromone (1) was treated with some nitrogen 1,2-bi-nucleophiles such as hydrazine hydrate, phenylhydrazine and hydroxylamine hydrochloride in the presence of diethyl phosphite at 70−80 °C for two hours afforded the corresponding novel azolyl phosphonates 6−8, respectively, as cyclic α-aminophosphonates (Scheme 3). Structures of the products 6−8 were elucidated from their spectroscopic data and elemental analysis. For example, their IR spectra revealed some characteristic absorption bands at 3132−3407 (NH, OH), 1636−1654 (C=O) and 1212−1216 cm-1 (P=O). Also, their 1H-NMR spectra revealed the appearance of the ethoxy protons in the regions δ 1.03−1.21 and 3.90−4.40 ppm while the H−5ˊ protons of the azole rings appeared as singlets at the region δ 7.30, 8.80 and 9.05 ppm, respectively. Furthermore, characteristic doublets were observed at δ 4.90, 4.94 and 5.65 ppm corresponding to the CH−P protons of compounds 6−8, respectively, besides D2O-exchangeable signals related to OH and NH protons. The 13C-NMR spectrum of compound 8 recorded the carbon atom of CH−P moiety as doublet at δ 46.0 ppm (J = 155.2 Hz) and the other carbon atoms for the isoxazole ring as singlets at δ 112.3 and 150.9 ppm. Moreover, it also showed highly deshielded carbon atom at δ 193.4 ppm, which indicated to the carbonyl group while the ethoxy carbon atoms appeared at δ 14.4 and 62.0 ppm. The phosphorus atom of phosphonate group resonated at δ 22.4 ppm in 31P-NMR spectrum of compound 6. The molecular ion peaks of compounds 6 and 7, were recorded at m/z 340 and 416, respectively, in their mass spectra.
In the present investigation, several subtypes of 1,3-bi-nucleophiles were used for recyclization of compound 1, in the presence of diethyl phosphite leading to the formation of some novel pyrimidinyl phosphonates as cyclic α-aminophosphonates. Thus, fusion of the aldehyde 1 with thiourea, guanidinium carbonate and cyanoguanidine in the presence of diethyl phosphite at 70−80 °C yielded a first type of pyrimidinyl phosphonates 9−11, respectively (Scheme 4). The latter phosphonates showed absorption bands at the regions 3134−3452, 1641−1648 and 1206−1230 cm-1 corresponding to OH, NH, C=O and P=O functions, while the nitrile group in compound 11 appeared at 2225 cm-1. The 1H-NMR spectra of compounds 9−11 displayed CH3 (δ 1.00−1.36 ppm) and CH2 (δ 3.60−4.00 ppm) protons of ethoxy groups in each product, while H−6ˊ protons of the pyrimidine rings were found in the aromatic region. Also, the doublets that appeared at δ 5.10, 5.20 and 5.03 ppm (J = 20, 19 and 18 Hz) for compounds 9−11, respectively, correspond to the CH−P protons. The 13C-NMR spectrum of compound 10 supported the presence of the carbonyl group which resonated at δ 186.1 ppm, while the carbon atoms of CH−P and C−2ˊ of the pyrimidine ring appeared at δ 47.0 and 163.6 ppm, respectively. Also, the 31P-NMR spectrum of compound 10 displayed a singlet at δ 26.2 ppm for the phosphonate group. Further confirmation of the structures 9 and 10 was obtained from their mass spectral data, where both structures showed their molecular ion peaks at m/z 384 and 367, in addition to their base peaks at m/z 64 and 64, respectively.
Seven-membered heterocycles with two heteroatoms are known to possess main fold biological activity.31−33 The present study was extended to investigate the behavior of the aldehyde 1 with classical nitrogen 1,4-bi-nucleophiles in the presence of diethyl phosphite with a view to synthesize phosphonates beard on seven-membered heterocyclic systems. Thus, treatment of aldehyde 1 with each one of nitrogen 1,4-bi-nucleophiles such as ethanolamine, ethylenediamine, 2-aminophenol and 1,2-phenylenediamine in the presence of diethyl phosphite at 70−80 °C for 4 hours furnished the corresponding phosphonate derivatives of 1,4-oxazepine 12, 1,4-diazepine 13, 1,5-benzoxazepine 14 and 1,5-benzodiazepine 15, respectively (Scheme 5). The IR spectra of the products 12−15 showed absorption bands at the regions 3232−3421, 1636−1646 and 1215−1228 cm-1 indicating the presence of NH, carbonyl and phosphonyl groups, respectively. The 1H-NMR spectra of compounds 12 and 13 exhibited characteristic broad singlets at δ 3.46, 4.34 and 3.42 ppm for CH2CH2 groups, respectively. Also, their 1H-NMR spectra showed characteristic doublets at δ 4.94 (J = 20 Hz) and 5.00 (J = 21 Hz) ppm for the CH−P while H−7ˊ protons of the seven-membered rings were found in the aromatic region. Furthermore, the 1H-NMR spectra of the benzo analogues 14 and 15 recorded coincident chemical shifts for CH−P protons at δ 5.10 and 5.18 ppm, respectively. In addition, the H−2ˊ protons of the 1,5-seven-membered rings appeared as singlets at δ 8.44 and 8.30 ppm for compounds 14 and 15, respectively. The 13C-NMR spectra of compounds 13 and 14 revealed the carbonyl groups at δ 185.0 and 189.6 ppm, respectively. In addition, the CH−P carbon atoms for them were recorded as doublets at δ 41.2 (J = 148.0 Hz) and 45.0 (J = 150 Hz) ppm, as well as other characteristic singlets at δ 150.1 and 155.3 ppm for the C−7ˊ and C−2ˊ atoms of the azepine rings 13 and 14, respectively. The mass spectra of compounds 12, 14 and 15 exhibited their molecular ion peaks at m/z 369, 417 and 416, respectively.
Next, we also studied the effect of acyclic and cyclic carbon nucleophiles which can construct various novel γ-heterocyclic phosphonates in an efficient and economic way. Thus, a simple fusion of 6-methyl-3-formylchromone (1) with each one of acyclic carbon nucleophiles namely, malononitrile and cyanoacetamide in the presence of diethyl phosphite and few drops of triethylamine at 70−80 °C for 6 hours afforded the same product 16 in moderate yields (Scheme 6). The latter product existed in two tautomeric forms which named diethyl {3-cyano-5-[(2-hydroxy-5-methylphenyl)carbonyl]-2-oxo-1,2,3,4-tetrahydropyridin-4-yl}phosphonate (16A) and diethyl {3-cyano-2-hydroxy-5-[(2-hydroxy-5- methylphenyl)carbonyl]-1,4-dihydropyridin-4-yl}phosphonate (16B) (Scheme 6). The suggested mechanism for construction of compound 16 may be the simple Knoevenagel condensation between compound 1 and the methylene compounds to form the condensation products A, followed by addition of diethyl phosphite to give the nonisolable intermediates B. The water molecules that resulted from condensation process, can hydrolyze the intermediate B (X=CN) to yield the corresponding acetamide intermediate C, which underwent opening of chromone ring at C−2 affording the stable pyridine ring bearing phosphonate moiety (Scheme 6). The structural elucidation of forms 16A and 16B was based on spectral data. The IR spectrum of compound 16 supported the form 16A which recorded the specific absorption bands at 3411 (br, NH), 2229 (C≡N), 1733 (C=Oamide), 1683 (C=Opyrone) and 1242 cm-1 (P=O) functions. Its 1H-NMR spectrum showed two multiplets at δ 1.08−1.35 and 3.80−3.95 ppm corresponding to CH3 and CH2 protons, respectively, while the doublet at δ 4.94 (J = 20 Hz) ppm due to the CH−P proton. Also, its 1H-NMR spectrum displayed a singlet at δ 8.40 ppm corresponds to H−6` of pyridine ring, and three D2O−exchangeable signals at δ 9.60, 10.70 and 11.75 ppm due to two OH and one NH protons, respectively, which supported the form 16B. Consequently, its 13C-NMR spectrum confirmed the existence of the enol form 16B through appearance of C−3` atom of the pyridine ring at δ 104.1 ppm, which means that this carbon atom has sp2 and not sp3 hybridized. In addition, there are three characteristic signals at δ 110.1, 187.1 and 197.2 ppm due to C≡N, C=Opyrone and C=Oamide, respectively. Moreover, its 31P-NMR spectrum displayed one singlet at δ 24.5 ppm. The mass spectrum of 16 recorded the M+1 and M+ peaks at m/z 393 and 392, respectively.
The high point in this work was the synthesis of some novel fused pyran systems containing phosphonate substrate at γ-position that would expected to have biological properties. Thus, heating of 6-methyl-3-formylchromone (1) and each one of cyclic carbon nucleophiles such as dimedone, 1-phenylpyrazolidine-3,5-dione and barbituric acid in the presence of diethyl phosphite and few drops of triethylamine at 70−80 °C for 8 h afforded the γ-pyranyl phosphonates 17−19, respectively (Scheme 7). A plausible mechanism for the formation of compounds 17−19 is depicted in Scheme 8. Initially, condensation process occurred between the formyl group and the cyclic active methylene groups forming the intermediate A, which underwent addition of diethyl phosphite at the exo CH=C bond to yield the phosphonate intermediate B. The keto-enol tautomerism between the intermediates B and C facilitates the nucleophilic attack of OH group on C−2 of chromone ring producing the new pyran ring (Scheme 8). Structures of the latter phosphonates were established from IR, MS and NMR spectral data. The mass spectra of products 18 and 19 recorded their molecular ion peaks at m/z 484 and 436, respectively. The IR spectra of compounds 17−19 indicated the appearance of the OH groups at 3444, 3392 and 3414 cm-1, respectively, and revealed in each product, two types of C=O bands at 1681, 1655; 1713, 1641 and 1713, 1628, respectively. Also, their 1H-NMR spectra showed characteristic singlets at δ 8.26, 8.40 and 8.60 ppm, respectively, due to α-protons of the pyran rings. Furthermore, each compound displayed a doublet for the CH−P proton at δ 4.51, 4.92 and 5.05 ppm for them with coupling constants 18, 18 and 15.6 Hz, respectively. In addition, the 13C-NMR spectrum of compound 19 confirmed the presence of the pyranopyrimidine system because of three characteristic signals were found at δ 43.0, 150.9 and 162.2 ppm corresponding to CH−P, C−7` and C=Oamide respectively. The 31P-NMR spectrum signal of the phosphonate 18 was found at δ 21.6 ppm.
Biological evaluations
1. Antimicrobial activity
All the newly synthesized compounds were evaluated in vitro for their antibacterial activities against Staphylococcus aureus (ATCC 25923) and Bacillus subtilis (ATCC 6635), as representatives of Gram−positive bacteria and Escherichia coli (ATCC 25922) and Salmonella typhimurium (ATCC 14028) as examples of Gram−negative bacteria. They were also examined against Candida albicans (ATCC 10231) as yeast and Aspergillus fumigatus as fungus. Agar diffusion technique was used for the determination of the preliminary antibacterial and antifungal activities.34,35 The test was performed on medium potato dextrose agar (PDA) which contained infusion of 200 g potatoes, 6 g dextrose and 15 g agar. Uniform size filter paper disks (3 disks per compound) were impregnated by equal volume (10 µL) from the concentrations of 500 and 1000 µg/mL dissolved compounds in dimethylformamide (DMF) and carefully placed on inoculated agar surface. After incubation for 36 hours at 27 °C in the case of bacteria and for 48 h at 24 °C in the case of fungi, the antimicrobial activities were determined by measuring the inhibition zones. Cephalothin, Chloramphenicol and Cycloheximide were used as reference drugs (30 µg/mL) for Gram positive bacteria, Gram negative bacteria and fungi, respectively. The minimum inhibitory concentration (MIC, µg/mL) for some selected compounds against some species of microbes was also determined. The tube dilution technique36 was applied for the determination of MIC of the tested compounds against microbes. Dilution series were set up with 250, 125, 62.5………3.25 µg/mL of nutrient broth medium to each tube, 100 mL of standardized suspension of the test microbes (107 cell/ mL) were added and incubated at 37 °C for 24 hours. The obtained results on the antimicrobial activities of the compounds and control drugs are given in Table 1. In general, the tested compounds recorded variable antimicrobial activities towards the used microorganism. The most compounds recorded low to moderate inhibitory effects towards all the microorganisms. The antimicrobial spectrum of the synthesized compounds against Gram-negative bacteria demonstrated very low inhibitions. Compound 16 exhibited moderate inhibition against Staphylococcus aureus with high MIC value >250 µg/mL. Similarly, compounds 8 and 11 recorded moderate inhibitions against Bacillus subtilis with high MIC value >250 µg/mL. All the tested compounds except 2 and 4 exhibited relatively low to high inhibitory activities against Candida albicans. Furthermore, compounds 5−9, 11 and 16 exhibited relatively moderate inhibitory activities against Candida albicans especially compounds 8 and 9 which recorded MIC values at 250 µg/mL. Compound 17 is the most effective one against Staphylococcus aureus and Bacillus subtilis with MIC values of 125 µg/mL. For activity against Candida albicans and Aspergillus fumigatus, compound 19 recorded the best activity with MIC values of 62.5 and 125 µg/mL, respectively, in comparison to the other synthesized compounds.
2. Antioxidant activity
1,1-Diphenyl-2-picrylhydrazyl (DPPH) has often been used to characterize antioxidants. It is reversibly reduced and the odd electron in the DPPH free radical gives a strong absorption maximum at λ 517 nm, which is purple in color. This property makes it suitable for spectrophotometric studies. A radical scavenging antioxidant reacts with DPPH stable free radical and converts into 1,1-diphenyl-2-picrylhydrazine. The resulting decolorization is stoichiometric with respect to the number of electrons captured. The change in the absorbance produced in this reaction has been used to measure antioxidant properties. The solutions of tested compounds (150, 300 and 450 µmol L-1) were added to DPPH (100 µmol L-1) in DMSO/EtOH. The tubes were kept at an ambient temperature for 20 minutes and the absorbance was measured at λ 517 nm. The difference between the test and the control experiments was taken and expressed as the percent scavenging of the DPPH radical using the following formula % inhibition = (AB−AA/AB) x 100 where AB=absorption of blank and AA=Absorption of tested compound. The radical scavenging activity of ascorbic acid was also measured and compared with that of the different synthesized compounds.37,38 The observed data on the antioxidant activities of the compounds and control are shown in Table 2 and illustrated in Figure 1. The results of scavenging the stable DPPH radical recorded variable antioxidant activities towards the synthesized compounds at the different concentrations 150, 300 and 450 µmol L-1. Compounds 2, 5, 7, 11, 12 and 15 showed moderate activities. In the meantime, compounds 3, 6, 8, 10, 14, 16, 17 and 19 displayed good activities. On the other hand, compounds 4, 9, 13 and 18 proved to exhibit potent antioxidative activities. The structure activity relationships of the tested compounds demonstrated that all the synthesized compounds recorded remarkable inhibition activities in range 37.81−78.35% at the different concentrations due to the presence of 4-methylphenol group in all the compounds except compounds 2−5 which have other free NH groups attached to phosphonate moieties. The cyclic α-aminophosphonates 6−15 and phosphonates 16−19 recorded noticeable antioxidative properties more than the acyclic α-aminophosphonates 2−5. This may due to the presence of free phenolic OH groups in compounds 6−19 which can scavenge the DPPH radical. The appearance of isoxazole unit in compound 8 exhibited greater activity than those having pyrazole units in compounds 6 and 7. Similarly, the thioxopyrimidinyl derivative 9 was more active than the other amino/cyanoiminopyrmidinyl derivatives 10 and 11. Amongst compounds having seven-membered rings 12−15, the diazepinyl derivative 13 exhibited the highest inhibition activity. On the other hand, the pyridine system 16 did not record the hoped antioxidative properties. The pyrazolopyranyl phosphonate 18 was the most active one between the pyranyl phosphonates 17−19. In this study, the systems 4, 9, 13 and 18 displayed the higher scavenging activities. However, the result exemplified that compound 13 having the diazepinyl unit in combination with phosphonic diester moiety is the most powerful antioxidant agent.
CONCLUSION
In conclusion, we have explored one-pot three component’s reaction, which furnished novel classes of functionalized heterocyclic analogues of acyclic and cyclic α-aminophosphonates and phosphonates from readily available 6-methyl-3-formylchromone, nitrogen and carbon mono- and bi-nucleophiles and diethyl phosphite. The procedure is efficient and general. The reactions have been shown to display relatively good functional group tolerance and good yields. We hope that this approach may be value to others seeking novel synthetic fragments with unique properties for medicinal chemistry.
EXPERIMENTAL
The melting point was determined in an open capillary tube on a digital Stuart SMP-3 apparatus. Infrared spectra were measured on FT-IR (Nicolet IS10) spectrophotometer using KBr disks. 1H-NMR spectra were measured on Gemini-300BB spectrometer (300 MHz), using DMSO-d6 as a solvent and TMS (δ) as the internal standard. 13C-NMR spectra were measured on Mercury-300BB (75 MHz using DMSO-d6 as a solvent) and Bruker-600 (150 MHz using CDCl3 as a solvent) spectrometer and TMS (δ) as the internal standard. 31P-NMR spectra were registered on a Bruker-600 (242 MHz) spectrometer at room temperature using DMSO-d6 as a solvent and TMS as internal standard and 85% H3PO4 as external reference. Mass spectra recorded on a Gas Chromatographic GCMSqp 1000 ex Shimadzu instrument at 70 ev. Elemental microanalyses were performed Perkin-Elmer 2400 II at the Chemical War department, Ministry of Defense. The purity of the synthesized compounds was checked by thin layer chromatography (TLC). 6-Methyl-3-formylchromone (1) was prepared according to the reported method.39
General procedure for the preparation of target compounds 2-19
A mixture of 6-methyl-3-formylchromone (1) (5 mmol, 0.94 g), nucleophile (5 mmol) and diethyl phosphite (10 mmol, 1.38 mL) was heated under reflux at 70−80 °C for 2−8 h (in case of 2, 8 and 16−19 few drops of triethylamine were added). The reaction mixture was cooled then poured into ice and left for complete precipitation. The precipitate formed was filtered off, dried and crystallized from the proper solvent.
Diethyl [(ethylamino)(6-methyl-4-oxo-4H-chromen-3-yl)methyl]phosphonate (2): Brown crystals from EtOH in 100% yield; mp 247−249 °C. IR (KBr), (νmax, cm-1): 3443 (br, NH), 3083 (C–Harom), 2987, 2893 (C–Haliph), 1640 (C=Opyrone), 1621 (C=C), 1228 (P=O), 1059 (P–O–C). 1H-NMR (300 MHz, DMSO–d6): 0.93 (t, 3H, J = 6.9 Hz, CH3), 1.00–1.15 (m, 6H, OCH2CH3), 2.41 (s, 3H, Ar–CH3), 2.92 (brs, 1H, NH exchangeable with D2O), 3.69 (q, 2H, J = 6.9 Hz, CH2), 3.93–4.00 (m, 4H, OCH2CH3), 4.39 (d, 1H, J = 24 Hz, CH–P), 7.58–7.66 (m, 2H, H–8 and H–7), 7.89 (s, 1H, H–5), 8.76 (s, 1H, H–2). MS (EI, m/z): 353 (M+). Anal. Calcd for C17H24NO5P (353.36): C, 57.79; H, 6.85; N, 3.96%. Found: C, 57.70; H, 6.92; N, 4.22%.
Diethyl [(benzylamino)(6-methyl-4-oxo-4H-chromen-3-yl)methyl]phosphonate (3): Pale yellow crystals from EtOAc in 75% yield; mp 229−230 °C. IR (KBr), (νmax, cm-1): 3439 (br, NH), 3070, 3032 (C–Harom), 2973, 2932 (C–Haliph), 1640 (C=Opyrone), 1620 (C=C), 1225 (P=O), 1053 (P–O–C). 1H-NMR (300 MHz, DMSO–d6): 0.93 (t, 6H, J = 7.5 Hz, OCH2CH3), 2.44 (s, 3H, Ar–CH3), 3.60 (q, 4H, OCH2CH3), 3.88 (brs, 1H, NH exchangeable with D2O), 4.22 (d, 2H, CH2), 4.48 (d, 1H, J = 15 Hz, CH–P), 7.29–7.67 (m, 7H, H–8, H–7 and Ph–H), 7.87 (s, 1H, H–5), 8.95 (s, 1H, H–2). 13C-NMR (75 MHz, DMSO): 16.5 (OCH2CH3), 20.4 (CH3), 45.5 (d, J = 148.5 Hz, CH–P), 56.0 (CH2), 60.4 (OCH2CH3), 117.4 (C–3), 118.2 (C–8), 122.4 (C–4a), 124.4 (C–4ˊphenyl), 128.1 (C–2ˊ, 6ˊphenyl), 128.4 (C–3ˊ, 5ˊphenyl), 130.4 (C–5), 131.9 (C–6), 135.2 (C–7), 135.3 (C–1ˊphenyl), 153.7 (C–2), 157.5 (C–8a), 174.6 (C=O). Anal. Calcd for C22H26NO5P (415.43): C, 63.61; H, 6.31; N, 3.37%. Found: C, 63.34; H, 6.49; N, 3.63%.
Diethyl [(4-chlorophenylamino)(6-methyl-4-oxo-4H-chromen-3-yl)methyl]phosphonate (4): Pale yellow crystals from EtOH in 70% yield; mp 196–198 °C (lit.,26 199–201 °C). IR (KBr), (νmax, cm-1): 3290 (NH), 3064 (C–Harom), 2991, 2897 (C–Haliph), 1646 (C=Opyrone), 1622 (C=C), 1221 (P=O), 1023 (P–O–C). 1H-NMR (300 MHz, DMSO–d6): 1.12 (t, 3H, J = 7.1 Hz, OCH2CH3), 1.28 (t, 3H, J = 7.1 Hz, OCH2CH3), 2.40 (s, 3H, Ar–CH3), 3.67–3.97 (m, 4H, OCH2CH3), 4.56 (brs, 1H, NH exchangeable with D2O), 5.21 (d, 1H, J = 23.2 Hz, CH–P), 6.37–7.70 (m, 7H, Ar–H), 8.20 (s, 1H, H–2). 13C-NMR (75 MHz, DMSO–d6): 16.0 (OCH2CH3), 20.3 (CH3), 45.2 (d, J = 158.5 Hz, CH–P), 62.6 (OCH2CH3), 114.6 (C–2ˊ,6ˊaryl), 118.2 (C–3), 120.1 (C–8), 120.9 (C–4`aryl), 122.3 (C–4a), 128.6 (C–3ˊ,5ˊaryl), 124.4 (C–5), 135.5 (C–6), 135.6 (C–7), 145.7 (C–1ˊaryl), 153.9 (C–2), 155.7 (C–8a), 174.8 (C=O). Anal. Calcd for C21H23ClNO5P (435.85): C, 57.87; H, 5.32; N, 3.21%. Found: C, 57.52; H, 5.28; N, 3.43%.
Diethyl [(4-hydroxyphenylamino)(6-methyl-4-oxo-4H-chromen-3-yl)methyl]phosphonate (5): Brown crystals from aq. DMF in 62% yield; mp 243–245 °C. IR (KBr), (νmax, cm-1): 3393 (br, OH, NH), 3079 (C–Harom), 2982, 2926 (C–Haliph), 1640 (C=Opyrone), 1617 (C=C), 1216 (P=O), 1046 (P–O–C). 1H-NMR (300 MHz, DMSO–d6): 1.02–1.20 (m, 6H, OCH2CH3), 2.21 (s, 3H, Ar–CH3), 3.66–3.81 (m, 4H, OCH2CH3), 4.00 (brs, 1H, NH exchangeable with D2O), 5.01 (d, 1H, J = 21.9 Hz, CH–P), 6.85–7.81 (m, 6H, Ar–H), 7.91 (s, 1H, H–5), 8.50 (s, 1H, H–2), 10.00 (brs, 1H, OH exchangeable with D2O). 31P-NMR (242 MHz, DMSO–d6): 21.6 ppm. Anal. Calcd for C21H24NO6P (417.40): C, 60.43; H, 5.80; N, 3.36%. Found. C, 60.09; H, 5.42; N, 3.54%.
Diethyl {4-[(2-hydroxy-5-methylphenyl)carbonyl]-2,3-dihydro-1H-pyrazol-3-yl}phosphonate (6): Yellow crystals from EtOAc in 55% yield; mp 210–212 °C. IR (KBr), (νmax, cm-1): 3407 (br, OH), 3139 (br, NH), 2974, 2940 (C–Haliph), 1636 (C=O), 1614 (C=C), 1212 (P=O), 1046 (P–O–C). 1H-NMR (300 MHz, DMSO–d6): 1.03 (t, 6H, J= 7.2 Hz, OCH2CH3), 2.17 (s, 3H, Ar–CH3), 4.00–4.20 (m, 4H, OCH2CH3), 4.90 (d, 1H, J = 20 Hz, CH–P), 6.84 (d, 1H, H–4), 7.03 (d, 1H, H–3), 7.30 (s, 1H, H–5ˊpyrazole), 7.65 (s, 1H, H–6), 8.70 (brs, 1H, NH exchangeable with D2O), 9.50 (brs, 1H, NH exchangeable with D2O), 10.70 (brs, 1H, OH exchangeable with D2O). 31P-NMR (242 MHz, DMSO–d6): 22.4 ppm. MS (EI, m/z): 341 (M+1), 340 (M+). Anal. Calcd for C15H21N2O5P (340.32): C, 52.94; H, 6.22; N, 8.23%. Found: C, 52.61; H, 5.87; N, 8.02%.
Diethyl {4-[(2-hydroxy-5-methylphenyl)carbonyl]-1-phenyl-2H-pyrazol-3-yl}phosphonate (7): Beige crystals from EtOH in 72% yield; mp 255–256 °C. IR (KBr), (νmax, cm-1): 3334 (br, OH), 3132 (br, NH), 3061 (C–Harom), 2982, 2914 (C–Haliph), 1654 (C=O), 1598 (C=C), 1212 (P=O), 1084 (P–O–C). 1H-NMR (300 MHz, DMSO–d6): 1.05 (t, 3H, J = 6.4 Hz, OCH2CH3), 1.20 (t, 3H, J = 6.4 Hz, OCH2CH3), 2.17 (s, 3H, Ar–CH3), 3.91–4.00 (m, 4H, OCH2CH3), 4.94 (d, 1H, J = 20 Hz, CH–P), 6.70–7.71 (m, 7H, Ar–H), 7.78 (s, 1H, Ar–H), 8.80 (brs, 1H, H–5ˊpyrazole), 9.40 (brs, 1H, NH exchangeable with D2O), 10.60 (brs, 1H, OH exchangeable with D2O). MS (EI, m/z): 416 (M+). Anal. Calcd for C21H25N2O5P (416.42): C, 60.57; H, 6.05; N, 6.73%. Found: C, 60.28; H, 5.82; N, 6.49%.
Diethyl {4-[(2-hydroxy-5-methylphenyl)carbonyl]-2,3-dihydro-isoxazol-3-yl}phosphonate (8): Brown crystals from EtOH in 52% yield; mp 100–102 °C. IR (KBr), (νmax, cm-1): 3390 (br, OH), 3246 (br, NH), 3020 (C–Harom), 2976, 2923 (C–Haliph), 1642 (C=O), 1622 (C=C), 1216 (P=O), 1041 (P–O–C). 1H-NMR (300 MHz, DMSO–d6): 1.07–1.21 (m, 6H, OCH2CH3), 2.36 (s, 3H, Ar–CH3), 4.20–4.40 (m, 4H, OCH2CH3), 5.65 (d, 1H, J = 18 Hz, CH–P), 7.23–7.80 (m, 2H, Ar–H), 7.98 (s, 1H, Ar–H), 9.05 (s, 1H, H–5ˊisoxazole), 9.66 (s, 1H, NH exchangeable with D2O), 10.43 (s, 1H, OH exchangeable with D2O). 13C-NMR (75 MHz, DMSO–d6): 14.4 (OCH2CH3), 20.4 (CH3), 46.0 (d, J = 155.2 Hz, CH–P), 62.0 (OCH2CH3), 112.3 (C–4ˊisoxazole), 116.0 (C–3), 121.3 (C–1), 134.3 (C–6), 135.6 (C–5), 137.0 (C–4), 150.9 (C–5ˊisoxazol), 154.3 (C–2), 193.4 (C=O). Anal. Calcd for C15H20NO6P (341.30): C, 52.79; H, 5.91; N, 4.10%. Found: C, 52.53; H, 5.63; N, 3.86%.
Diethyl {5-[(2-hydroxy-5-methylphenyl)carbonyl]-2-thioxo-1,2,3,4-tetrahydropyrimidin-4-yl}- phosphonate (9): Pale brown crystals from EtOH in 53% yield; mp 196–198 °C. IR (KBr), (νmax, cm-1): 3293 (br, OH, NH), 3050 (C–Harom), 2980, 2927 (C–Haliph), 1641 (C=O), 1619 (C=C), 1214 (P=O), 1044 (P–O–C), 1165 (C=S). 1H-NMR (300 MHz, DMSO–d6): 1.08–1.36 (m, 6H, OCH2CH3), 2.28 (s, 3H, Ar–CH3), 3.80–4.00 (m, 4H, OCH2CH3), 5.10 (d, 1H, J = 20 Hz, CH–P), 7.40–7.80 (m, 3H, Ar–H and H–6ˊpyrimidine), 7.95 (s, 1H, Ar–H), 8.40 (brs, 1H, NH exchangeable with D2O), 9.10 (brs, 1H, OH exchangeable with D2O), 9.80 (brs, 1H, NH exchangeable with D2O). MS (EI, m/z): 384 (M+). Anal. Calcd for C16H21N2O5PS (384.39): C, 50.00; H, 5.51; N, 7.29; S, 8.33%. Found: C, 49.71; H, 5.24; N, 6.97; S, 7.97%.
Diethyl {2-amino-5-[(2-hydroxy-5-methylphenyl)carbonyl]-3,4-dihydropyrimidin-4-yl}phosphonate (10): Pale beige crystals from EtOH in 65% yield; mp 303–305 °C. IR (KBr), (νmax, cm-1): 3452 (OH), 3302, 3134 (NH2, NH), 3020 (C–Harom), 2984, 2920 (C–Haliph), 1648 (C=O), 1600 (C=N), 1583 (C=C), 1206 (P=O), 1028 (P–O–C). 1H-NMR (300 MHz, DMSO–d6): 1.07 (t, 3H, J = 6.9 Hz, OCH2CH3), 1.15 (t, 3H, J = 6.9 Hz, OCH2CH3), 2.31 (s, 3H, Ar–CH3), 3.60–3.95 (m, 4H, OCH2CH3), 5.20 (d, 1H, J = 19 Hz, CH–P), 6.92 (d, 1H, J = 8.4 Hz, Ar–H), 7.25 (d, 1H, J = 6 Hz, Ar–H), 7.38 (brs, 2H, NH2 exchangeable with D2O), 7.88 (s, 1H, Ar–H), 8.00 (brs, 1H, NH exchangeable with D2O), 8.24 (s, 1H, H–6ˊpyrimidine), 9.46 (s, 1H, OH exchangeable with D2O). 13C-NMR (75 MHz, DMSO–d6): 16.0 (OCH2CH3), 20.3 (CH3), 47.0 (d, J = 148.5 Hz, CH–P), 61.8 (OCH2CH3), 113.7 (C–3), 117.6 (C–5ˊpyrimidine), 123.9 (C–1), 130.2 (C–6), 133.1 (C–5), 135.0 (C–4), 152.1 (C–6ˊpyrimidine), 156.2 (C–2), 163.6 (C–2ˊpyrimidine), 186.1 (C=O). 31P-NMR (242 MHz, DMSO–d6): 26.2 ppm. MS (EI, m/z): 368 (M+1), 367 (M+). Anal. Calcd for C16H22N3O5P (367.34): C, 52.32; H, 6.04; N, 11.44%. Found: C, 52.01; H, 5.81; N, 11.07%.
Diethyl {2-(cyanoimino)-5-[(2-hydroxy-5-methylphenyl)carbonyl]-1,2,3,4-tetrahydro-pyrimidin-4-yl}- phosphonate (11): Beige crystals from EtOH in 48% yield; mp 201–202 °C. IR (KBr), (νmax, cm-1): 3405 (br, OH, NH), 2982, 2928 (C–Haliph), 2225 (C≡N), 1643 (C=O), 1620 (C=N), 1230 (P=O), 1046 (P–O–C). 1H-NMR (300 MHz, DMSO–d6): 1.00–1.20 (m, 6H, OCH2CH3), 2.29 (s, 3H, Ar–CH3), 3.80–4.00 (m, 4H, OCH2CH3), 5.03 (d, 1H, Jˊ = 18 Hz, CH–P), 6.96–7.61 (m, 3H, Ar–H and H–6ˊpyrimidine), 7.82 (s, 1H, Ar–H), 8.39 (brs, 1H, NH exchangeable with D2O), 9.42 (brs, 1H, NH exchangeable with D2O), 10.89 (brs, 1H, OH exchangeable with D2O). Anal. Calcd for C17H21N4O5P (392.35): C, 52.04; H, 5.39; N, 14.28%. Found: C, 51.77; H, 5.30; N, 14.15%.
Diethyl {6-[(2-hydroxy-5-methylphenyl)carbonyl]-2,3,4,5-tetrahydro-1,4-oxazepin-5-yl}- phosphonate (12): Pale yellow crystals from EtOAc in 100% yield; mp 302–303 °C. IR (KBr), (νmax, cm-1): 3417 (br, OH, NH), 3056 (C–Harom), 2974, 2893 (C–Haliph), 1645 (C=O), 1622 (C=C), 1216 (P=O), 1073 (P–O–C). 1H- NMR (300 MHz, DMSO–d6): 1.00–1.16 (m, 6H, OCH2CH3), 2.27 (s, 3H, Ar–CH3), 3.46 (brs, 2H, CH2), 3.80–4.00 (m, 4H, OCH2CH3), 4.34 (brs, 2H, CH2), 4.94 (d, 1H, J = 20 Hz, CH–P), 7.50–7.68 (m, 3H, Ar–H and H–7ˊoxazepine), 7.90 (s, 1H, Ar–H), 8.87 (brs, 1H, NH exchangeable with D2O), 9.60 (brs, 1H, OH exchangeable with D2O). MS (EI, m/z): 370 (M+1), 369 (M+). Anal. Calcd for C17H24NO6P (369.36): C, 55.28; H, 6.55; N, 3.79%. Found: C, 54.98; H, 6.23; N, 3.56%.
Diethyl {6-[(2-hydroxy-5-methylphenyl)carbonyl]-2,3,4,5-tetrahydro-1H-1,4-diazepin-5-yl}- phosphonate (13): Brown crystals from EtOAc in 43% yield; mp 210–212 °C. IR (KBr), (νmax, cm-1): 3397 (br, OH, NH), 3030 (C–Harom), 2980, 2920 (C–Haliph), 1646 (C=O), 1622 (C=C), 1228 (P=O), 1045 (P–O–C). 1H-NMR (300 MHz, DMSO–d6): 0.91–1.20 (m, 6H, OCH2CH3), 2.27 (s, 3H, Ar–CH3), 3.42 (brs, 4H, CH2CH2), 3.80–4.00 (m, 4H, OCH2CH3), 5.00 (d, 1H, J = 21 Hz, CH–P), 6.08 (brs, 1H, NH exchangeable with D2O), 6.84–7.62 (m, 3H, Ar–H and H–7ˊdiazepine), 7.90 (s, 1H, Ar–H), 9.60 (brs, 2H, OH and NH exchangeable with D2O). 13C-NMR (150 MHz, CDCl3): 14.9 (OCH2CH3), 22.1 (CH3), 41.2 (d, J = 148.0 Hz, CH–P), 54.9 (CH2N), 58.5 (CH2N), 59.9 (OCH2CH3), 115.5 (C–3), 117.5 (C–6ˊdiazepine), 121.1 (C–1), 131.2 (C–6), 133.5 (C–4), 150.1 (C–7ˊdiazepine), 151.5 (C–2), 185.0 (C=O). 31P-NMR (242 MHz, DMSO˗d6): 28.8 ppm. Anal. Calcd for C17H25N2O5P (368.37): C, 55.43; H, 6.84; N, 7.60%. Found: C, 55.20; H, 6.56; N, 7.32%.
Diethyl {3-[(2-hydroxy-5-methylphenyl)carbonyl]-4,5-dihydro-1,5-benzoxazepin-4-yl}phosphonate (14): Yellow crystals from EtOH in 7% yield; mp 186–187 °C. IR (KBr), (νmax, cm-1): 3417 (br, OH), 3232 (br, NH), 3020 (C–Harom), 2981, 2934 (C–Haliph), 1641 (C=O), 1617 (C=C), 1228 (P=O), 1026 (P–O–C). 1H-NMR (300 MHz, DMSO–d6): 1.11 (t, 3H, J = 6.9 Hz, OCH2CH3), 1.22 (t, 3H, J = 6.9 Hz, OCH2CH3), 2.42 (s, 3H, Ar–CH3), 3.94–4.15 (m, 4H, OCH2CH3), 5.10 (d, 1H, J = 15 Hz, CH–P), 6.44–6.92 (m, 4H, Ar–H), 7.53–7.66 (m, 2H, Ar–H), 7.88 (s, 1H, Ar–H), 8.44 (s, 1H, H–2ˊbenzoxazepine), 9.56 (s, 1H, NH exchangeable with D2O), 10.35 (brs, 1H, OH exchangeable with D2O). 13C-NMR (75 MHz, DMSO–d6): 16.0 (OCH2CH3), 20.4 (CH3), 45.0 (d, J = 150 Hz, CH–P), 62.5 (OCH2CH3), 110.8 (C–6ˊ), 113.8 (C–3), 117.7 (C–3ˊ), 118.2 (C–8ˊ), 119.7 (C–9ˊ), 122.3 (C–1), 124.2 (C–7ˊ), 134.5 (C–6), 134.7 (C–5), 135.4 (C–4), 144.7 (C–5ˊa), 145.7 (C–9ˊa), 153.8 (C–2ˊ), 155.3 (C–2), 189.6 (C=O). MS (EI, m/z): 417 (M+). Anal. Calcd for C21H24NO6P (417.40): C, 60.34; H, 5.80; N, 3.36%. Found: C, 60.10; H, 5.94; N, 3.55%.
Diethyl {3-[(2-hydroxy-5-methylphenyl)carbonyl]-4,5-dihydro-1H-1,5-benzo-diazepin-4-yl}- phosphonate (15): Yellow crystals from EtOH in 67% yield; mp 228–230 °C. IR (KBr), (νmax, cm-1): 3421 (br, OH, NH), 3055 (C–Harom), 2979, 2917 (C–Haliph), 1636 (C=O), 1618 (C=C), 1215 (P=O), 1049 (P–O–C). 1H-NMR (300 MHz, DMSO–d6): 1.04–1.35 (m, 6H, OCH2CH3), 2.23 (s, 3H, Ar–CH3), 4.20–4.40 (m, 4H, OCH2CH3), 5.18 (d, 1H, J = 20 Hz, CH–P), 6.83–6.96 (m, 4H, Ar–H), 7.31–7.65 (m, 2H, Ar–H), 7.87 (s, 1H, Ar–H), 8.30 (s, 1H, H–2ˊbenzodiazepine), 9.57 (brs, 1H, NH exchangeable with D2O), 11.82 (brs, 2H, OH and NH exchangeable with D2O). MS (EI, m/z): 416 (M+). Anal. Calcd for C21H25N2O5P (416.42): C, 60.57; H, 6.05; N, 6.73%. Found: C, 60.2; H, 5.89; N, 6.41%.
Diethyl {3-cyano-5-[(2-hydroxy-5-methylphenyl)carbonyl]-2-oxo-1,2,3,4-tetrahydropyridin-4-yl}- phosphonate (16A) and diethyl {3-cyano-2-hydroxy-5-[(2-hydroxy-5-methyl-phenyl)carbonyl]- 1,4-dihydropyridin-4-yl}phosphonate (16B): Yellow crystals from EtOH in 61 and 58% yields; mp 198–200 °C. IR (KBr), (νmax, cm-1): 3411 (br, OH, NH), 3050 (C–Harom), 2982, 2929, 2861 (C–Haliph), 2229 (C≡N), 1733 (C=Oamide), 1683 (C=O), 1622, 1598 (C=C), 1242 (P=O), 1032 (P–O–C). 1H NMR (300 MHz, DMSO–d6): 1.08–1.35 (m, 6H, OCH2CH3), 2.27 (s, 3H, Ar–CH3), 3.80–3.95 (m, 4H, OCH2CH3), 4.94 (d, 1H, J = 20 Hz, CH–P), 7.37–7.60 (m, 2H, Ar–H), 7.80 (brs, 1H, Ar–H), 8.40 (brs, 1H, H–6ˊpyridine), 9.60 (brs, 1H, OH exchangeable with D2O), 10.70 (brs, 1H, OH exchangeable with D2O), 11.75 (brs, 1H, NH exchangeable with D2O). 13C-NMR (150 MHz, CDCl3): 16.0 (OCH2CH3), 23.8 (CH3), 43.5 (d, J = 147.6 Hz, CH–P), 62.9 (OCH2CH3), 104.1 (C–3ˊ), 110.1 (C≡N), 115.3 (C–3), 117.9 (C–5ˊ), 120.1 (C–1), 131.9 (C–6), 134.0 (C–4), 134.1 (C–5), 140.8 (C–6ˊ), 155.7 (C–2), 187.1 (C=O), 197.2 (C–2ˊ). 31P-NMR (242 MHz, DMSO–d6): 24.5 ppm. MS (EI, m/z): 393 (M+1), 392 (M+). Anal. Calcd for C18H21N2O6P (392.35): C, 55.10; H, 5.39; N, 7.14%. Found: C, 54.95; H, 5.14; N, 7.42%.
Diethyl {7,7-dimethyl-3-[(2-hydroxy-5-methylphenyl)carbonyl]-5-oxo-5,6,7,8-tetrahydro-4H- chromen-4-yl}phosphonate (17): Pale yellow crystals from EtOH in 48% yield; mp 303–305 °C. IR (KBr), (νmax, cm-1): 3444 (br, OH), 2957, 2867 (C–Haliph), 1681 (C=O), 1655 (C=O), 1622 (C=C), 1203 (P=O), 1003 (P–O–C). 1H-NMR (300 MHz, DMSO–d6): 0.83 (s, 3H, CH3), 1.00 (s, 3H, CH3), 1.33 (t, 6H, J = 7.5 Hz, OCH2CH3), 2.04 (s, 2H, CH2), 2.28 (s, 3H, Ar–CH3), 3.62 (q, 4H, J = 7.5 Hz, OCH2CH3), 4.28 (s, 2H, CH2), 4.51 (d, 1H, J = 18 Hz, CH–P), 7.46–7.54 (m, 2H, Ar–H), 7.71 (s, 1H, Ar–H), 8.26 (brs, 1H, H–2ˊpyran), 9.38 (s, 1H, OH exchangeable with D2O). Anal. Calcd for C23H29O7P (448.46): C, 61.60; H, 6.52%. Found: C, 61.24; H, 6.51%.
Diethyl {5-[(2-hydroxy-5-methylphenyl)carbonyl]-1-phenyl-4H-3-oxo-pyrano[2,3-c]pyrazol-4-yl}- phosphonate (18): Beige crystals from EtOH in 54% yield; mp 252–254 °C. IR (KBr), (νmax, cm-1): 3392 (br, OH, NH), 3064 (C–Harom), 2980, 2980 (C–Haliph), 1713 (C=Oamide), 1641 (C=O), 1617 (C=C), 1212 (P=O), 1084 (P–O–C). 1H-NMR (300 MHz, DMSO–d6): 1.00–1.21 (m, 6H, OCH2CH3), 2.16 (s, 3H, Ar–CH3), 4.10–4.21 (m, 4H, OCH2CH3), 4.92 (d, 1H, J = 18 Hz, CH–P), 6.60–7.70 (m, 8H, Ar–H and Ph–H), 8.40 (brs, 1H, H–6ˊpyran), 9.52 (brs, 2H, OH and NH exchangeable with D2O). 31P-NMR (242 MHz, DMSO–d6): 21.6 ppm. MS (EI, m/z): 484 (M+). Anal. Calcd for C24H25N2O7P (484.45): C, 59.50; H, 5.20; N, 5.78%. Found: C, 59.21; H, 4.96; N, 5.38%.
Diethyl {6-[(2-hydroxy-5-methylphenyl)carbonyl]-1,5-dihydro-2H-2,4-dioxo-pyrano[2,3-d]- pyrimidin-5-yl}phosphonate (19): Brown crystals from DMF in 100% yield; mp 193–195 °C. IR (KBr), (νmax, cm-1): 3414 (br, OH), 3182 (br, NH), 3020 (C–Harom), 2986, 2911 (C–Haliph), 1713 (2 C=Oamide), 1628 (C=O), 1212 (P=O), 1011 (P–O–C). 1H-NMR (300 MHz, DMSO–d6): 1.07 (t, 3H, J = 6.6 Hz, OCH2CH3), 1.20 (t, 3H, J = 6.6 Hz, OCH2CH3), 2.23 (s, 3H, Ar–CH3), 3.42 (q, 2H, J = 6.6 Hz, OCH2CH3), 3.86 (q, 2H, J = 6.6 Hz, OCH2CH3), 5.05 (d, 1H, J = 15.6 Hz, CH–P), 6.93–7.27 (m, 2H, Ar–H), 7.79 (s, 1H, Ar–H), 8.60 (brs, 1H, H–7ˊpyran), 9.23 (brs, 1H, OH exchangeable with D2O), 10.67, 11.20 (brs, 2H, NH exchangeable with D2O). 13C-NMR (150 MHz, CDCl3): 15.0 (OCH2CH3), 21.3 (CH3), 43.0 (d, J = 133.5 Hz, CH–P), 60.8 (OCH2CH3), 95.1 (C–4ˊa), 115.3 (C–3), 117.4 (C–6ˊ), 121.7 (C–1), 132.0 (C–6), 135.9 (C–4), 150.9 (C–7ˊ), 155.0 (C–2), 160.5 (C–8ˊa), 162.2 (2 C=Oamide), 186.5 (C=O). MS (EI, m/z): 437 (M+1), 436 (M+). Anal. Calcd for C19H21N2O8P (436.36): C, 52.30; H, 4.85; N, 6.42%. Found: C, 51.98; H, 4.63; N, 6.02%.
References
1. P. Kafarski and B. Lejczak, Phosphorus, Sulfur and Silicon Relat. Elem., 1991, 63, 193. CrossRef
2. A. Peyman, K. H. Budt, J. Spanig, B. Stowasser, and D. Rupert, Tetrahedron Lett., 1992, 33, 4549. CrossRef
3. R. Markwell, In Aminophosphonic and Aminophosphinic Acids Derivatives as Inhibitors of Human Collagenase, ed by V. P. Kukhar and H. R. Hudson, Aminophosphonic and Aminophosphinic Acids, Wiley, Chichester, 2000, pp. 579−621.
4. J. Z. Liu, B. A. Song, H. T. Fan, P. S. Bhadury, W. T. Wan, S. Yan, W. Xu, J. Wu, L. H. Jin, X. Wei, D. Y. Hu, and S. Zeng, Eur. J. Med. Chem., 2010, 45, 5108. CrossRef
5. Y. H. Reddy, B. S. Kumar, G. C. K. Reddy, E. Dadapeer, and K. S. Reddy, Der Chemica Sinica, 2012, 4, 81.
6. Y. Zhang, S. Bai, B. Song, P. S. Bhadury, D. Hu, S. Yang, X. Zhang, H. Fan, and P. Lu, J. Chromatoghraphy B, 2010, 878, 1285. CrossRef
7. E. N. Jascobsen and P. A. Bartlett, J. Am. Chem. Soc., 1981, 103, 654. CrossRef
8. V. P. Kukhar and V. P. Solodenko, Phosphorus, Sulfur and Silicon Relat. Elem., 1994, 92, 239. CrossRef
9. D. Y. Hu, D. D. Wan, S. Yang, B. A. Song, P. S. Bhadury, L. H. Jin, K. Yan, F. Liu, Z. Chen, and W. Xue, J. Agric. Food Chem., 2008, 56, 998. CrossRef
10. S. Micheal, C. Jay, Z. Ding, S. Aaron, D. C. Atasi, D. Siddhartha, and E. P. Stenen, Org. Lett., 2010, 12, 4596. CrossRef
11. Z. Miao, J. Zhang, Z. Cui, B. Wang, and R. Chen, Helv. Chim. Acta, 2007, 90, 1932. CrossRef
12. M. M. Kabachnik, T. N. Ternovskaya, E. V. Zobnina, and I. P. Beletskaya, Russ. J. Org. Chem., 2002, 38, 484. CrossRef
13. R. Hirschmann, A. B. Smith, C. M. Taylor, P. A. Benkovic, S. D. Taylor, K. M. Yager, P. A. Sprengler, and S. J. Benkovic, Science, 1994, 265, 234. CrossRef
14. S. G. Lee, J. K. Lee, C. E. Song, and D. C. Kim, Bull. Korean Chem. Soc., 2002, 23, 667. CrossRef
15. S. Bhagat and A. K. Chakraborti, J. Org. Chem., 2007, 72, 1263. CrossRef
16. I. Yavari, A. Mirzaei, and G. Khalili, Helv. Chim. Acta, 2010, 93, 654. CrossRef
17. I. Yavari, A. Mirzaei, and L. Moradi, Synth. Commun., 2010, 40, 2407. CrossRef
18. R. Lavilla, A. Spada, and J. Bosch, Org. Lett., 2000, 2, 1533. CrossRef
19. D. Albouy, M. Lasptras, G. Etemad-Moghadam, and M. Koenig, Tetrahedron Lett., 1999, 40, 2311. CrossRef
20. A. Belyaev, X. Zhang, K. Augustyns, A. Lambeir, I. De Meester, I. Vedernikova, S. L. Scharpe, and A. Haemers, J. Med. Chem., 1999, 42, 1041. CrossRef
21. T. E. Ali, Eur. J. Med. Chem., 2009, 44, 4539. CrossRef
22. T. E. Ali, ARKIVOC, 2008, ii, 71. CrossRef
23. T. E. Ali, Phosphorus, Sulfur and Silicon Relat. Elem., 2010, 185, 88. CrossRef
24. S. A. Abdel-Aziz, T. E. Ali, K. M. El-Mahdy, and S. M. Abdel-Karim, Eur. J. Chem., 2011, 2, 25. CrossRef
25. T. E. Ali, Heteroat. Chem., 2013, 24, 426. CrossRef
26. S. Y. Lang, D. Z. Wang, and L. H. Cao, Phosphorus, Sulfur and Silicon Relat. Elem., 2009, 184, 2560. CrossRef
27. N. S. Zefirov and E. D. Matveeva, ARKIVOC, 2008, i, 1. CrossRef
28. G. X. Sabitha, Aldrichimica Acta, 1996, 29, 15.
29. C. K. Y. Ghosh, Heterocycles, 2004, 63, 2875. CrossRef
30. A. S. Plaskon, O. O. Grygorenko, and S. V. Ryabukhin, Tetrahedron, 2012, 68, 2743. CrossRef
31. X. H. Liu, Y. M. Jia, B. A. Song, Z. X. Pang, and S. Yang, Bioorg. Med. Chem. Lett., 2013, 23, 720. CrossRef
32. M. Xie, R. K. Ujjinamatada, M. Sadowska, R. G. Lapidus, M. J. Edelman, and R. S. Hosmane, Bioorg. Med. Chem. Lett., 2010, 20, 4386. CrossRef
33. A. Moure, M. Orzlez, M. Sancho, and A. Messeguer, Bioorg. Med. Chem. Lett., 2012, 22, 7097. CrossRef
34. A. U. Rahman, M. I. Choudhary, and W. J. Thomsen, Bioassay Techniques for Drug Development, Harwood Academic Publishers, Netherlands, 2001. CrossRef
35. K. M. Khan, Z. S. Saify, A. K. Zeesha, M. Ahmed, M. Saeed, M. Schick, H. J. Bkohlbau, and W. Voelter, Arzneim-Forsch/Drug Res., 2000, 50, 915.
36. D. Mishra, S. Patnaik, C. C. Rath, S. K. Dash, R. K. Mishra, and U. Patnaik, Indian J. Pharm. Sci., 2002, 64, 256.
37. K. Kato, S. Terao, N. Shimamoto, and M. Hirata, J. Med. Chem., 1988, 31, 793. CrossRef
38. P. Siddhuraju and K. Becker, Food Chem., 2007, 101, 10. CrossRef
39. A. Nohara, T. Umetani, and Y. Sanno, Tetrahedron, 1974, 30, 3553. CrossRef