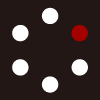
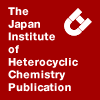
HETEROCYCLES
An International Journal for Reviews and Communications in Heterocyclic ChemistryWeb Edition ISSN: 1881-0942
Published online by The Japan Institute of Heterocyclic Chemistry
e-Journal
Full Text HTML
Received, 12th October, 2013, Accepted, 11th November, 2013, Published online, 26th November, 2013.
DOI: 10.3987/COM-13-S(S)118
■ Regio- and Enantioselective Nitrone Cycloaddition to Alkynones for the Synthesis of Δ4-Isoxazolines
Mukund P. Sibi,* Kelsey L. Dunkle, and Digamber Rane
Department of Chemistry and Biochemistry, North Dakota State University, Fargo, North Dakota 58108, U.S.A.
Abstract
We report herein a strategy for Lewis acid catalyzed enantioselective 1,3-dipolar cycloaddition of nitrones with alkynones to generate Δ4-isoxazolines. The corresponding cycloadducts were isolated as a single regioisomer with high yields and enantioselectivities.Construction of chiral heterocycles continues to be a challenge for organic chemists. Dipolar cycloaddition is one of the premiere methods for the construction of heterocycles.2 During the past few decades several methodologies have been developed for the construction of enantioenriched heterocycles via dipolar cycloadditions and these include Lewis acid or organocatalyzed reactions with olefinic dipolarophiles. Lewis acid catalyzed cycloaddition of dipoles such as azomethine imines,3 diazoacetates,4 and nitrile oxides5 etc to olefinic dipolarophiles have been thoroughly investigated. Nitrones are one such important class of dipoles. Dipolar cycloaddition of nitrones to olefinic and acetylenic dipolarophiles furnish isoxazolidines and isoxazolines respectively.6 These represent an important class of heterocycles possessing biological significance.7
Chiral Lewis acid catalyzed nitrone cycloadditions to electron deficient olefins have been studied in great detail and there are several significant reports on this topic.8 However, examples of enantioselective nitrone cycloadditions to acetylenic dienophiles are scarce and can be attributed to the geometry of the dipolarophile.9 As opposed to olefinic dipolarophiles which have sp2-sp2 bonds, acetylenic dipolarophiles due to their linear sp-sp bonds are challenging since the reaction center is away from the source of chirality control element. Hence a proper selection of chiral Lewis acid is essential to obtain high levels of enantioselectivity. Regiochemistry is another important issue in dipolar cycloaddition and it would be interesting to see if one can obtain high levels of regioselective product in the cycloaddition of nitrones to acetylenic dienophiles.10 While developing such a methodology care should be taken so as to encompass a broad substrate scope for it to be useful. With these goals in mind we set upon developing a general method for the construction of Δ4-isoxazolines, which led to the development of a Lewis acid catalyzed strategy for a highly regio- and enantioselective 1,3-dipolar cycloaddition of nitrones to acetylenic dipolarophiles.
We began optimizing reaction conditions for the cycloaddition of nitrone 2a with 1-methylimidazole tethered alkynone 1a. These results are summarized in Table 1. Evans and co-workers first demonstrated the use of 1-methylimidazole as a cheap and commercially available achiral template.11 Initial investigations were preformed using lanthanum Lewis acids such as Sc(III), Ce(IV), Gd(III) etc. and gave satisfactory yield of the desired cycloadduct as a single regioisomer. Excellent yield and good enantioselectivity was achieved for reactions catalyzed by Sc(OTf)3/4 (entry 1). Other chiral Lewis acid complexes prepared from 4 and Ce(IV), Gd(III), Dy(III) and Yb(III) were also examined but failed to give high levels of enantioselectivity. Additionally we also screened Sc(OTf)3/5 complex as the chiral Lewis acid which gave slightly inferior enantioselectivity compared to Sc(OTf)3/4 complex without any loss in reactivity (compare entries 1 and 2). Other alkaline earth metals such as Zn(II) and Mg(II) were also examined. The reaction of Mg(OTf)2/6 gave the desired cycloadduct 3a in high yield however with poor enantioselectivity (entry 3). Chiral Lewis acid complex of 6 with Zn(OTf)2 gave the cycloadduct 3a in 86% enantiomeric excess and 96% yield (entry 4). Zn(NTf2)2 whose counter ion is more dissociable and hence more acidic than Zn(OTf)2 gave the cycloadduct 3a with 53% yield and in 76% enantiomeric excess (entry 5). This preliminary screening of various chiral Lewis acid suggested that Zn(OTf)2/6 complex was slightly superior over other chiral alkaline earth metal and chiral lanthanum Lewis acids tested for asymmetric 1,3-dipolar cycloaddition of alkynone 1a with nitrone 2a and that the bulky amino indanol group containing bis(oxazoline) ligands were essential for imparting high levels of enantioselectivity. Thus Zn(OTf)2/6 complex was selected as the optimum chiral Lewis acid and further studies were conducted using this Lewis acid ligand combination. With the optimized chiral Lewis acid we decided to investigate the effect of lowering the catalyst loading on reaction yield and enantioselectivity. Lowering the catalyst loading to 10 mol% of Zn(OTf)2/6 gave the desired cycloadduct 3a in excellent yield, but with some erosion of enantioselectivity (entry 6). Additionally, the catalyst loading could be reduced to 5 mol% with the expense of further lowering the yield and further eroding the enantiomeric excess of the cycloadduct 3a (entry 7). Catalyst loading could be further reduced but was not pursued and a loading of 20 mol% of chiral Lewis acid complex of Zn(OTf)2/6 was deemed to be a prefect balance between reactivity and selectivity for the dipolar cycloaddition of alkynone 1a with nitrone 2a.
After optimizing the chiral Lewis acid and the catalyst loading we proceeded to explore the scope of dipolarophile by varying various β-substituent R on the alkynone. These results are summarized in Table 2. These alkynones can be rapidly synthesized using known and modified synthetic procedures.12 Furthermore, terminal substituent R was chosen so as to represent an array of structural motifs commonly encountered in organic chemistry and these include acyclic, cyclic, aromatic and heteroaromatic groups.
We have previously discussed that enantioselective dipolar cycloaddition reaction of nitrone 2a with alkynone 1a bearing a β-methyl group catalyzed by 20 mol% of Zn(OTf)2/6 complex gave the desired cycloadduct 3a in 96% yield and 86% enantiomeric excess (Table 2, entry 1). Replacing the β-substituent with a cyclic alkyl group had detrimental effect on both yield and enantioselectivity of the cycloadduct. Thus, alkynone 1b bearing cyclopentyl group gave the desired cycloadduct 3b in 82% yield and 47% enantiomeric excess (entry 2). However, the reaction was sluggish and required 7 days for completion. Typically, substitution of the β-position of an olefinic dienophile with aromatic ring generally leads to a tremendous decrease in reactivity relative to an alkyl substituent and is one of the most challenging group for substrate scope studies. In keeping with this fact, we found that nitrone cycloaddition to alkynones possessing a β-phenyl group required elongated periods of time for completion. Thus the reaction of alkynone 1c containing a phenyl group as the β-substituent with nitrone 2a required 7 days to yield cycloadduct 3c in 95% yield and 77% enantiomeric excess (entry 3). An aromatic substituent with an electron donating substituent did not have appreciable impact on reactivity or selectivity. Thus, in alkynone 1d whose β-substituent R is p-MeOPh yielded the cycloadduct 3d in 91% yield and 70% enantiomeric excess (entry 4). Finally, an alkynone containing a heteroaromatic group as a β-substituent was also evaluated. Thus, alkynone 1e whose group R was 3-thiophenyl reacted with nitrone 2a to furnish the cycloadduct 3e in 95% yield and in 72% enantiomeric excess (entry 5). From these studies we can conclude that the nature of the β-substituent has tremendous impact on the reactivity of the alkynone and that any change in the steric volume at the β-position of the alkynone lowers the reaction rate. Also based on the enantiopurity of the cycloadduct it could be inferred that aromatic and heteroaromatic β-substituents which present a flat steric volume are better as compared to cyclic substituents with a somewhat spherical steric volume.
After exploring the substituent scope on the alkynones we set out to expand the scope of this methodology by studying the scope of nitrones. These results are summarized in Table 3. A variety of nitrones were prepared by condensation of the corresponding N-substituted hydroxylamine with aromatic aldehydes using known protocols.
The reactivity of 1a made it an ideal candidate as a dipolarophile for this study. Cycloaddition of nitrone 2a with alkynone 1a was discussed previously (entry 1). N-Benzyl nitrone 2b derived from anisaldehyde gave cycloadduct 3f in 88% yield and 73% enantiomeric excess (entry 2).11 Nitrones derived from aromatic aldehydes possessing strong electron withdrawing groups at the para position also reacted with alkynone 1a to yield the desired cycloadduct in good yield but with lower enantioselectivity. Thus nitrone 2c derived from p-nitrobenzaldehyde furnished cycloadduct 3g in 84% yield and 51% enantiomeric excess (entry 3). Surprisingly, nitrone 1d derived from p-chlorobenzaldehyde was found to be one of the least reactive dipole and required 7 days to yield cycloadduct 3h in excellent yield however in modest enantiomeric excess of 56% (entry 4). Nitrones derived from ortho- or meta- substituted benzaldehydes are often not ideal for substrate scope studies since the ortho- or meta- substituents inhibit the approach of the dipole towards the reaction center by steric repulsion. We observed that nitrone 2e derived from m-bromobenzaldehyde reacted slowly as compared to nitrone 2a providing the cycloadduct with slightly lower yield and enantioselectivity (compare entries 1 and 5). Impact of the substitution on nitrogen atom of the nitrone was also investigated. Thus nitrone 2f derived from N-methylhydroxylamine reacted comparably to N-benzyl substituted nitrone furnishing the desired cycloadduct 3j in good yield and modest selectivity (compare entries 5 and 6). Surprisingly N-methylnitrone 2g derived from anisaldehyde was less reactive than the corresponding N-benzylnitrone, however it furnished the desired cycloadduct 3k with similar yield and enantiomeric excess (compare entries 2 and 7). Finally N-PMB substituted nitrones was also examined. Nitrone 2h gave the cycloadduct 3l in 98% yield and 76% enantioselectivity (entry 8). The absolute stereochemistry of the product was ascertained by single crystal X-ray analysis of cycloadduct 3k and was determined to be (R).
In conclusion, we have developed an efficient strategy for the construction of optically active Δ4-isoxazolines via dipolar cycloaddition of nitrones with electron deficient alkynes. Cycloadducts derived from a wide variety of nitrones and terminally substituted alkynones were prepared in high yields with modest to good enantioselectivity and as a single regioisomer. This represents one of the few strategies for enantioselective nitrone cycloaddition to acetylenic dienophiles to yield enantioenriched Δ4-isoxazolines. Further work is in progress in our laboratory to exploit the synthetic utility of these chiral cycloadducts.
Supporting Information
Experimental procedures, characterization data for starting materials and final cycloadducts are available. This material is available via the internet at http://www.heterocycles.jp. Deposition number CCDC-964327 for compound No. 3k. Free copies of the data can be obtained via http://www.ccdc.cam.ac.uk/conts/retrieving.html (or from the Cambridge Crystallographic Data Centre, 12 Union Road, Cambridge, CB2 1EZ, UK; Fax: +44 1223 336033; e-mail: deposit@ccdc.cam.ac.uk).
ACKNOWLEDGEMENTS
We thank the National Science Foundation (NSF- CHM-0709061) for financial support. This article is dedicated to Prof. Victor Snieckus on the occasion of his 77th birthday. Congratulations Victor. You are a great scientist, mentor and friend.
References
1. Present Address: Department of Medicinal Chemistry, University of Kansas, Lawrence, Kansas 66047, USA.
2. For a comprehensive review of 1,3-dipolar cycloadditions, see: Synthetic Application of 1,3-Dipolar Cycloaddition Chemistry towards Heterocycles and Natural Products; ed. by A. Padwa and W. H. Pearson; John Wiley and Sons: Hoboken, NJ, 2003. For reviews on asymmetric 1,3-dipolar cycloadditions, see: (a) K. V. Gothelf and K. A. Jørgensen, Chem. Rev., 1998, 98, 863; CrossRef (b) H. Pellissier, Tetrahedron, 2007, 63, 3235; CrossRef For reviews on nitrone cycloadditions, see: K. Braun-Rück, T. H. E. Freysoldt, and F. Weirschem, Chem. Soc. Rev., 2005, 34, 507q; CrossRef (c) M. Frederickson, Tetrahedron, 1997, 53, 403; CrossRef (d) W. Zhang, Chem. Lett., 2013, 42, 676; CrossRef (e) S. Kanemasa, Heterocycles, 2010, 82, 87; CrossRef (f) T. Hashimoto and K. Maruoka, in Handbook of Cyclization Reactions, ed. by S. Ma; 2010, 1, 87-168; (g) H. Suga, Topics in Heterocyclic Chemistry, 2009, 19, 119.
3. For selected recent examples of enantioselective cycloadditions of azomethine imines, see: (a) W. Chen, Y.-Z. Duan, Y. Wu, S.-Y. Yang, and Y.-C. Chen, Angew. Chem. Int. Ed., 2007, 46, 7667; CrossRef (b) H. Suga, A. Funyu, and A. Kakehi, Org. Lett., 2007, 9, 97; CrossRef (c) S. Milosevic and A. Togni, J. Org. Chem., 2013, 78, 9638; CrossRef (d) H. Suga, T. Arikawa, K. Itoh, Y. Okumura, A. Kakehi, and M. Shiro, Heterocycles, 2010, 81, 1669. CrossRef
4. For enantioselective cycloadditions of diazoalkanes and diazoacetate, see: (a) S. Kanemasa and T. Kanai, J. Am. Chem. Soc., 2000, 122, 10710; CrossRef (b) T. Kano, T. Hashimoto, and K. Maruoka, J. Am. Chem. Soc., 2006, 128, 2174; CrossRef (c) M. P. Sibi, L. M. Stanley, and T. Soeta, Org. Lett., 2007, 9, 1553; CrossRef (d) G. A. Molander and L. N. Cavalcanti, Org. Lett., 2013, 15, 3166; CrossRef (e) N. Jiang and C.-J. Li, Chem. Commun., 2004, 394. CrossRef
5. For enantioselective cycloaddition of nitrile oxides, see: (a) M. P. Sibi, K. Itoh, and C. P. Jasperse, J. Am. Chem. Soc., 2004, 126, 5366; CrossRef (b) H. Suga, Y. Adachi, K. Fujimoto, Y. Furihata, T. Tsuchida, A. Kakehi, and T. Baba, J. Org. Chem., 2009, 74, 1099; CrossRef (c) M. Tsuji, Y. Ukaji, and K. Inomata, Chem. Lett., 2002, 1112; CrossRef (d) For enantioselective cycloaddition of nitrile imines, see: M. P. Sibi, L. M. Stanley, and C. P. Jasperse, J. Am. Chem. Soc., 2005, 127, 8276. CrossRef
6. For use of Δ4-isoxazoline as building blocks, see: (a) I. Adachi, R. Miyazaki, and H. Kanõ, Chem. Pharm. Bull., 1974, 22, 70; CrossRef (b) A. Padwa and G. S. K. Wong, J. Org. Chem., 1986, 51, 3125; CrossRef (c) B. Zhao, Y. Yu, and S. Eguchi, Tetrahedron, 1996, 52, 12049; CrossRef (d) M. Closa, P. March, M. Figueredo, and J. Font, Tetrahedron: Asymmetry, 1997, 8, 1031; CrossRef (e) W. R. Bowmann, R. V. Davis, A. M. Z. Slawin, G. S. Sohal, R. B. Titman, and D. J. Wilkins, J. Chem. Soc., Perkin Trans. 1, 1997, 155; CrossRef (f) T. Ishikawa, T. Kudoh, J. Yoshida, A. Yasuhara, S. Manabe, and S. Saito, Org. Lett., 2002, 4, 1907; CrossRef (g) Y. Tomioka, C. Nagahiro, Y. Nomura, and H. Maruoka, J. Heterocycl. Chem., 2003, 40, 121; CrossRef (h) W. Friebolin and W. Eberbach, Helv. Chim. Acta, 2001, 84, 3822; CrossRef (i) E. Lopez-Calle, M. Keller, and W. Eberbach, Eur. J. Org. Chem., 2003, 1438; CrossRef (j) A. Y. Bitar and A. J. Frontier, Org. Lett., 2009, 11, 49; CrossRef (k) S. H. Kim and S. Chang, Org. Lett., 2010, 12, 1868; CrossRef (l) H. Valizadeh and L. Dinparast, Synth. Commun., 2011, 41, 291; CrossRef (m) S. M. M. Lopes, C. M. Nunes, and T. M. V. D. Pinho e Melo, Tetrahedron, 2010, 66, 6078; CrossRef For reviews on application of Δ4-isoxazolines, see: (n) J. P. Freeman, Chem. Rev., 1983, 83, 241; CrossRef (o) T. M. V. D. Pinho e Melo, Eur. J. Org. Chem., 2010, 3363. CrossRef
7. For application of Δ4-isoxazolines in medicinal chemistry, see: (a) A. G. Habeeb, P. N. Rao, and E. E. Knaus, J. Med. Chem., 2001, 44, 2921; CrossRef (b) R. D. Cramer, R. J. Jilek, S. Guessregen, S. J. Clark, B. Wendt, and R. D. Clark, J. Med. Chem., 2004, 47, 6777; CrossRef (c) M. E. Fraley, R. M. Garbaccio, and G. D. Hartmann, Patent WO 2006023440, 2006.
8. For selective examples of enantioselective nitrone cycloadditions to olefins using chiral Lewis acids, see: (a) M. P. Sibi, Z. Ma, and C. P. Jasperse, J. Am. Chem. Soc., 2004, 126, 718; CrossRef (b) H. Suga, T. Nakajima, K. Itoh, and A. Kakehi, Org. Lett., 2005, 7, 1431; CrossRef (c) G. Desimoni, G. Faita, M. Mella, and M. Boiocchi, Eur. J. Org. Chem., 2005, 1020; CrossRef (d) K. B. Simonsen, P. Bayón, R. G. Hazell, K. V. Gothelf, and K. A. Jørgensen, J. Am. Chem. Soc., 1999, 121, 3845; CrossRef (e) A. Badoiu and E. P. Kuendig, Org. Biomol. Chem. 2012, 10, 114; CrossRef (f) D. Carmona, M. P. Lamata, F. Viguri, R. Rodriguez, L. A. Oro, F. J. Lahoz, A. I. Balana, T. Tejero, and P. Merino, J. Am. Chem. Soc., 2005, 127, 13386; CrossRef (g) T. Kano, T. Hashimoto, and K. Maruoka, J. Am. Chem. Soc., 2005, 127, 11926; CrossRef (h) L. Weselinski, E. Kalinowska, and J. Jurczak, Tetrahedron: Asymmetry, 2012, 23, 264; CrossRef (i) K-C. Lim, Y-T. Hong, and S. Kim, Adv. Synth. Catal., 2008, 350, 380; CrossRef (j) X. Ding, K. Taniguchi, Y. Hamamoto, K. Sada, S. Fujinami, Y. Ukaji, and K. Inomata, Bull. Chem. Soc. Jpn., 2006, 79, 1069; CrossRef (k) D. Carmona, M. P. Lamata, F. Viguri, R. Rodriguez, L. A. Oro, F. J. Lahoz, A. I. Balana, T. Tejero, and P. Merino, J. Am. Chem. Soc., 2005, 127, 13386. CrossRef
9. For other examples for enantioselective selective synthesis of Δ4- isoxazolines from alkynes, see: (a) A. Sakakura, M. Hori, M. Fushimi, and K. Ishihara, J. Am. Chem. Soc., 2010, 132, 15550; CrossRef (b) W. Wei, M. Kobayashi, Y. Ukaji, and K. Inomata, Heterocycles, 2009, 78, 717; CrossRef (c) J. Aleman, A. Fraile, L. Marzo, J. L. Garcia Ruano, C. Izquierdo, and S. Diaz-Tendero, Adv. Synth. Catal., 2012, 354, 1665; CrossRef (d) X. Cai, C. Wang, and J. Sun, Adv. Synth. Catal., 2012, 354, 359. CrossRef
10. For studies on regiochemistry in dipolar cycloadditions, see: (a) J. Sims and K. N. Houk, J. Am. Chem. Soc., 1973, 95, 5798; CrossRef (b) K. N. Houk, J. Sims, R. E. Duke Jr., R. W. Strozier, and J. K. George, J. Am. Chem. Soc., 1973, 95, 7287; CrossRef (c) K. N. Houk, J. Sims, C. R. Watts, and L. J. Luskus, J. Am. Chem. Soc., 1973, 95, 7301; CrossRef (d) M. D. Gordon, P. V. Alston, and A. R. Rossi, J. Am. Chem. Soc., 1978, 100, 5701; CrossRef (e) A. Liguori, R. Ottana, G. Romeo, G. Sindona, and N. Uccella, Tetrahedron, 1988, 44, 1247; CrossRef (f) M. Burdisso, R. Gandolfi, and P. Grünanger, Tetrahedron, 1989, 45, 5579; CrossRef (g) H. G. Aurich, M. Franzke, H. P. Kesselheim, and M. Rohr, Tetrahedron, 1992, 48, 669; CrossRef (h) D. H. Ess and K. N. Houk, J. Am. Chem. Soc., 2008, 130, 10187; CrossRef (i) Y. Hu and K. N. Houk, Tetrahedron, 2000, 56, 8239; CrossRef (j) S. Fukuda, A. Kamimura, S. Kanemasa, and K. Hori, Tetrahedron, 2000, 56, 1637. CrossRef
11. For an example of application of 1-methyl imidazole as template, see: (a) D. A. Evans, K. R. Fandrick, and H. Song, J. Am. Chem. Soc., 2005, 127, 8942; CrossRef (b) M. Yoshida, H. Ohmiya, and M. Sawamura, J. Am. Chem. Soc., 2012, 134, 11896; CrossRef c) B. M. Trost and T. M. Lam, J. Am. Chem. Soc., 2012, 134, 11319; CrossRef (d) A. J. Boersma, B. L. Feringa, and G. Roelfes, Org. Lett., 2007, 9, 3647; CrossRef (e) E. L. Tyson, E. P. Farney, and T. P. Yoon, Org. Lett., 2012, 14, 1110; CrossRef (f) X. Xu, W-H. Hu, and M. P. Doyle, Angew. Chem. Int. Ed., 2011, 50, 6392; CrossRef (g) X-Y. Guan, L-P. Yang, and W. Hu, Angew. Chem. Int. Ed., 2010, 49, 2190; CrossRef (h) R. P. Megens and G. Roelfes, Chem. Commun., 2012, 48, 6366. CrossRef
12. See supporting information and reference mentioned within.