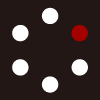
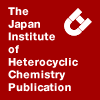
HETEROCYCLES
An International Journal for Reviews and Communications in Heterocyclic ChemistryWeb Edition ISSN: 1881-0942
Published online by The Japan Institute of Heterocyclic Chemistry
e-Journal
Full Text HTML
Received, 16th October, 2013, Accepted, 11th November, 2013, Published online, 20th November, 2013.
DOI: 10.3987/COM-13-S(S)119
■ A Formal PCB-Free Synthesis of (-)-GSK1360707 via a Double Alkylation Reaction
Vassil I. Elitzin, Matthew J. Sharp, and Elie A. Tabet*
Product Development, GlaxoSmithKline, Research Triangle Park, North Carolina 27709, U.S.A.
Abstract
The formal synthesis of the triple reuptake inhibitor (-)-GSK1360707 is described. The synthesis features an intramolecular cyclization and a double alkylation sequence to produce the 3-azabicyclo[4.1.0]heptane skeleton. An advantage of this route is the absence of PCB generation which plagued an earlier route.The 3-azabicyclo[4.1.0]heptane skeleton is an important ring system in medicinal chemistry. Compounds containing this ring system have been shown to be human orexin receptor antagonists,1 triple reuptake inhibitors,2 and might serve as a building block in the synthesis of glycomimetics, peptidomimetics and secondary-structure inducing elements.3 Typical methods for introduction of the cyclopropane ring involve sulfur ylide chemistry,3 Simmons-Smith reaction,1b rhodium(II) acetate carbene chemistry1b and enyne cycloisomerization.4 GSK1360707 is a potent serotonin, noradrenaline, and dopamine reuptake (triple reuptake) inhibitor that was under development at GlaxoSmithKline for the treatment of major depressive disorder (MDD).2 Previously, we disclosed a new synthetic route to GSK1360707 based on double alkylation methodology to form the cyclopropane ring (Scheme 1).5 Despite its many advantages, the overall process suffers from one major drawback. It produces PCB 77 (3,3’,4,4’-tetrachloro-1,1’-
biphenyl, an EPA regulated substance) as a minor product of the Suzuki coupling step between vinyl triflate 1 and 3,4-dichlorophenylboronic acid 2. This impurity is formed via dimerization of the 3,4-dichlorophenylboronic acid.6
Due to the potential PCB issue associated with homocoupling of the dichlorophenylboronic acid (Scheme 1),5 we decided to explore other routes toward GSK1360707 that avoid cross coupling of a dichlorophenyl moiety.7 With this in mind, we envisioned that the commercially available 3,4-dichlorocinnamic acid is an attractive starting material containing the key C-C bond. Michael addition between ethyl cyanoacetate 7 and 3,4-dichlorocinnamic ester 6 could provide, after some manipulations, access to ester 5 (Scheme 2). A double alkylation reaction similarly to our previous route would deliver cyclopropane 4. In this paper, we describe an alternative synthesis of 3-azabicyclo[4.1.0]hept-4-ene-1-carboxylate 4, which was previously converted to (-)-GSK1360707 in 4 steps.5
The synthesis began with the formation of enoate 6 via the esterification of carboxylic acid 8 using EtI, and K2CO3 in DMF (Scheme 3). Michael addition of ethyl cyanoacetate 7 to enoate 6 was performed using Cs2CO3/NMP to give diester 9. While sodium alkoxides were reported in the literature for similar Michael additions,8 we found that Cs2CO3/NMP produced more robust conditions on scale. After hydrogenation of the nitrile to the corresponding amine using PtO2,8 treatment with K2CO3 in EtOH/H2O gave the lactam as a 1:1.4 cis/trans mixture by HPLC. Equilibration to the trans isomer 10 was accomplished using KOH in EtOH. While it was expected that both isomers could be progressed, the equilibration was performed to simplify the analysis of this and subsequent intermediates. Attempts to perform the cyclization and isomerization in one step were not efficient due to competing ester hydrolysis. Next, the nitrogen was protected with Boc2O to give lactam carbamate 11. The Boc protecting group served two purposes – it was needed to form the enecarbamate moiety in compound 59 and to later intercept intermediate 4 from our original synthesis of GSK1360707 (Scheme 1).5 Thus, reduction of the lactam carbamate 11 was carried out with Super Hydride (LiBHEt3) followed by dehydration of the resultant hemiaminal to give enecarbamate 5.9
Installation of the cyclopropane moiety was next investigated. Our original conditions to form the cyclopropane included an intermolecular alkylation with CH2ICl followed by an intramolecular displacement of the remaining chloride.5 This double alkylation was performed in one pot using LiOtBu in NMP. Unfortunately, these conditions failed to cleanly produce the desired cyclopropane 4 from enecarbamate 5. The use of NaH/THF was also investigated without any success. As a result, a two step strategy was pursued. First alkylation of enecarbamate 5 was accomplished with LiHMDS and CH2ICl to give chloride 12. Additives such as LiCl, TMEDA and DMPU gave lower yields in the alkylation reaction. No attempt was made to determine the relative stereochemistry of 12. However, the NMR and mass spectra were consistent with the proposed structure. Finally, chloride 12 was treated with LiHMDS and DMPU at room temperature to give cyclopropane 4,10,11 which was spectroscopically identical to that previously reported.5 It is also worth mentioning that in the absence of the olefin in 1212 we were unable to close the cyclopropane ring using LiHMDS/THF/DMPU even at elevated temperatures. A simple benzylic activation was not enough to induce the intramolecular alkylation.
In conclusion, we have shown that cyclopropane 4 can be synthesized via an alternative, PCB free route. Thus a formal synthesis of (-)-GSK1360707 was accomplished. The key steps in this synthesis are a lactam carbamate reduction and an intramolecular cyclopropanation. The intramolecular cyclopropanation has proven to be versatile as it is applicable to different substrates (3 and 5).
ACKNOWLEDGEMENTS
We are grateful to David Black, Kimberly Harvey and John Grimes for analytical support.
References
1. R. Di Fabio WO 2012/089607 A1; R. Di Fabio WO 2012/089606 A1; D. Amantini and R. Di Fabio WO 2010/122151 A1.
2. F. Micheli, P. Cavanni, D. Andreotti, R. Arban, R. Benedetti, B. Bertani, M. Bettati, L. Bettelini, G. Bonanomi, S. Braggio, R. Carletti, A. Checchia, M. Corsi, E. Fazzolari, S. Fontana, C. Marchioro, E. Merlo-Pich, M. Negri, B. Oliosi, E. Ratti, K. D. Read, M. Roscic, I. Sartori, S. Spada, G. Tedesco, L. Tarsi, S. Terreni, F. Visentini, A. Zocchi, L. Zonzini, and R. Di Fabio, J. Med. Chem., 2010, 53, 4989. CrossRef
3. C. Napolitano, M. Borriello, F. Cardullo, D. Donati, A. Paio, and S. Manfredini, Tetrahedron, 2010, 66, 5492. CrossRef
4. A. Fürstner, H. Szillat, and F. Stelzer, J. Am. Chem. Soc., 2000, 122, 6785; CrossRef A. Fürstner, F. Stelzer, and H. Szillat, J. Am. Chem. Soc., 2001, 123, 11863. CrossRef
5. V. I. Elitzin, K. A. Harvey, H. Kim, M. Salmons, M. J. Sharp, E. A. Tabet, and M. A. Toczko, Org. Process Res. Dev., 2010, 14, 912. CrossRef
6. C. Adamo, C. Amatore, I. Ciofini, A. Jutand, and H. Lakmini, J. Am. Chem. Soc., 2006, 128, 6829; CrossRef Y. Yamamoto, R. Suzuki, K. Hattori, and H. Nishiyama, Synlett, 2006, 1027; CrossRef M. Moreno-Manas, M. Perez, and R. Pleixats, J. Org. Chem., 1996, 61, 2346; CrossRef S. B. Kedia and M. B. Mitchell, Org. Process Res. Dev., 2009, 13, 420. CrossRef
7. Along with Prof. Fürstner, we have developed an enyne cycloisomerization route to GSK1360707. However, both routes involve cross coupling with 3,4-dichloroiodobenzene. N. M. Deschamps, V. I. Elitzin, B. Liu, M. B. Mitchell, M. J. Sharp, and E. A. Tabet, J. Org. Chem., 2011, 76, 712; CrossRef H. Teller and A. Fürstner, Chem. Eur. J., 2011, 17, 7764. CrossRef
8. W. H. Moos, R. D. Gless, and H. Rapoport, J. Org. Chem., 1981, 46, 5064; CrossRef K. Sugi, N. Itaya, T. Katsura, M. Igi, S. Yamazaki, T. Ishibashi, T. Yamaoka, Y. Kawada, Y. Tagami, M. Otsuki, and T. Ohshima, Chem. Pharm. Bull., 2000, 48, 529; CrossRef J. M. Cox, S. D. Edmondson, and A. Mastracchio WO/2007/070434.
9. J. Yu, V. Truc, P. Riebel, E. Hierl, and B. Mudryk, Tetrahedron Lett., 2005, 46, 4011 and references therein. CrossRef
10. For similar intermolecular alkylation see M. Essamkaoui, J. Mayrargue, J.-M. Vierfond, A. Reynet, H. Moskowitz, and C. Thal, Synth. Commun., 1992, 22, 2723; CrossRef For similar intramolecular alkylation see B. Rieger, G. Jany, R. Fawzi, and M. Steimann, Organometallics, 1994, 13, 647. CrossRef
11. (±)-3-(1,1-Dimethylethyl)-1-ethyl 6-(3,4-dichlorophenyl)-3-azabicyclo[4.1.0]hept-4-ene-1,3-dicarboxylate (4): 1-(1,1-Dimethylethyl) 3-ethyl 3-(chloromethyl)-4-(3,4-dichlorophenyl)-3,4-dihydro-1,3(2H)-pyridinedicarboxylate 12 (0.18 g, 0.401 mmoles) was dissolved in THF (1.4 mL, 1.24 g, 17.3 mmoles). DMPU (0.24 mL, 0.25 g, 1.98 mmoles) and LiHMDS (0.88 mL, 0.88 mmoles, 1M in THF) were added and the resulting reaction was stirred at ~22 oC for 5 h. The reaction was cooled to ~0 oC and quenched with 1M HCl (3 mL). EtOAc (3 mL) was added and the reaction was warmed to ~22 oC. The layers were separated. The organic layer was dried over Na2SO4, filtered and concentrated under vacuum. The material was purified by chromatography (heptane and then gradient of 2.5 to 12.5% EtOAc/heptane) to give 0.15 g of solid 4 (91% yield). 1H NMR (400 MHz, CDCl3) δ 7.32 (d, J = 4 Hz, 1H), 7.31 (d, J = 8 Hz, 1H), 7.06 (dd, J = 8, 4 Hz, 1H), 6.72-6.49 (br d, 1H), 5.2-5.05 (br d, 1H), 4.4-4.1 (br dd, 1H), 3.85-3.66 (m, 3H), 2.26 (d, J = 4 Hz, 1H), 1.53 (d, J = 4 Hz, 1H), 1.47 (s, 9H), 0.95-0.8 (br s, 3H).
12. The olefin was removed by treating 12 with Et3SiH, TFA and PhMe followed by re-protection of the nitrogen with Boc2O.