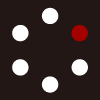
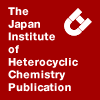
HETEROCYCLES
An International Journal for Reviews and Communications in Heterocyclic ChemistryWeb Edition ISSN: 1881-0942
Published online by The Japan Institute of Heterocyclic Chemistry
e-Journal
Full Text HTML
Received, 9th October, 2013, Accepted, 31st October, 2013, Published online, 22nd November, 2013.
DOI: 10.3987/COM-13-12859
■ Regioselectivity of the Palladium-Mediated Intramolecular Coupling Reaction of Highly Oxygenated Phenyl Benzoate Derivatives and Application to the Synthesis of Altertenuol
Takuya Matsukihira, Shumpei Saga, Yoshikazu Horino, and Hitoshi Abe*
Department of Environmental Applied Chemistry, Faculty of Engineering, Toyama University, Gofuku 3190, Toyama 930-8555, Japan
Abstract
The regioselectivity of the intramolecular biaryl coupling reaction of 3’,4’-dialkoxyphenyl 2,4-dimethoxybenzoates was investigated using a palladium reagent, and transition state models of the reaction are proposed. As an application of this study, a short-step synthesis of altertenuol is also performed.INTRODUCTION
A palladium-mediated intramolecular biaryl coupling reaction of phenyl benzoate derivatives is a useful transformation for the preparation of the 6H-dibenzo[b,d]pyran-6-one skeleton.1 There have been many studies of the total syntheses of natural products possessing this type of heterocyclic system, using the intramolecular biaryl coupling reaction.2 In some cases, regioselectivity is a crucial concern in the intramolecular biaryl coupling, thus we recently reported several types of reactions involving the issue of regioselectivity.3 In this report, as a series of our study, regioselectivity is examined in the Pd-mediated intramolecular biaryl coupling reaction of 3’,4’-dialkoxyphenyl 2,4-dimethoxybenzoates, and the synthesis of a natural polyphenolic compound, altertenuol,4 is also described.
RESULTS AND DISCUSSION
As the substrates for the investigation, we prepared three kinds of phenyl benzoate derivatives 1a-1c, which were easily obtained by a simple esterification between the corresponding phenols 2a-2c and benzoic acid 3. Initially, the regioselectivity was investigated using Ag2CO3 as the base (Table 1). For the ester 1a, when Ph3P was employed as the ligand, the ortho-product 5a mainly generated. However, a poor regioselectivity for para- and ortho-products, 4a and 5a, was observed under no ligand condition (entries 1 and 2). On the contrary, for the substrates 1b and 1c, a dramatic change was observed in the product ratio. Namely, when using Ph3P, the regioselectivity exhibited 1.4-0.7:1, although when using no ligand, the para-isomers 4b and 4c were predominantly generated (7.9-8.8:1, entries 3-6).
In contrast to the results above, employing K2CO3 as the base led to a different outcome for the product ratio. When several phosphine ligands5 were attempted with the ester 1a, the regioselectivity was almost identical to the result in entry 1 (entries 7-9). On the other hand, a slightly increased ratio was observed when using no phosphine ligand (entry 10). In the case of both 1b and 1c, a moderate selectivity was observed in the range of 1.2-3.6:1 under the condition that the phosphine ligand was employed (entries 11, 13, 14, and 15). When no ligand was used for 1b and 1c, the reaction proceeded with the product ratio of 4.4-4.5:1 (entries 12 and 16).
For the mechanistic aspect, we would be able to explain the regioselectivity by considering the transition state models.3i Based on Echavarren’s intermolecular-assisted mechanism,6 the transition state of substrate 1a can be illustrated as Figure 1. A1 and A2 describe the models in the case of using Ag2CO3 as the base. Strong affinity between the silver and the iodo atom causes a partial cationic character on the palladium. Therefore, the alkoxy oxygen can coordinate to the palladium atom (A2) leading to providing the ortho-products 5a-5c, whereas the steric hindrance, which was generated between the alkoxy group and the ligand on the palladium atom, is also significant (A2; L = phosphine). Consequently, the product ratios are controlled by the balance of both the coordinating power and the steric factor. However, when using no phosphine ligand, the tight solvation of the DMA takes place to make the surrounding of the palladium to be bulkier (A1 and A2; L = DMA). Thus, no phosphine ligand condition leads to the severe steric repulsion between the alkoxy group and DMA, as shown in A2, so that the ratio of the para-products 4a-4c is increased.
Contrary to the above, B1-B3 illustrate the transition state models under the condition using K2CO3 as the base (Figure 2). In these cases, the cationic property of the palladium atom may not be considered because of the hard character of the potassium ion. The coordinating effect of the alkoxy group to the palladium is not an important factor for the substrates 1b and 1c, thus the para-isomers 4b and 4c are slightly predominant (B1 and B2). Since the coordinating ability of the methylenedioxy group of the substrate 1a is stronger than 1b and 1c, the attractive interaction between the oxygen and the palladium can control and mainly produce the ortho-isomer 5a (B3).
Since the coupling product 4c was regioselectively obtained with the conditions of entry 6 in Table 1, we attempted a further transformation toward the natural product, alternenuol 6, which was found from Alternaria tenuis in 1957.7 The selective demethylation of 4c by the Node’s method8 was successful to afford 7, which was debenzylated by the conventional hydrogenolysis reaction leading to 6. As an alternative way, partial dealkylation with BBr3 proceeded effectively to produce 6 in a single step. The spectral data of synthetic 6 were in accordance with the reported data.4
CONCLUSION
The regioselectivity of the Pd-mediated biaryl coupling reaction of 3’,4’-dialkoxyphenyl 2,4-dimethoxybenzoates was intensively investigated. It was found that the combination of the ligand and the base influences the regioselectivity of the reaction. We also demonstrated the synthesis of altertenuol using the regioselective condition. This method would be utilized for further application to other syntheses of biaryl-type natural products.
EXPERIMENTAL
General Information
Melting points were measured using a Yanagimoto micro melting point hot-plate and are uncorrected. The IR spectra were recorded using a SHIMADZU FTIR-8400 spectrophotometer. The NMR spectra were obtained using a JEOL α-400 (400 MHz) instrument. The chemical shifts are given in δ ppm with TMS as an internal standard. The elemental analyses were performed using a Thermo Scientific FlashEA1112 analyzer. Silica gel column chromatography was carried out using a wakogel® C-200 or C-400HG (Wako).
3,4-Dimethoxyphenol (2b)9
A mixture of THF (2 mL), 31% H2O2 (0.13 mL), H3BO3 (189 mg, 3.05 mmol), and conc. H2SO4 (0.06 mL) was stirred for 30 min at rt. To the mixture was added a solution of 3,4-dimethoxybenzaldehyde (101 mg, 0.607 mmol) in THF (1 mL). The mixture was stirred for 19 h at rt, then a saturated aqueous NaHCO3 solution was added. The mixture was extracted with CHCl3 and the organic layer was washed with brine. After drying with MgSO4 and evaporation, a residue was subjected to silica gel column chromatography with hexane-AcOEt (4:1) to give 2b (73.1 mg, 78%) as colorless prisms, mp 83.9-85.2 °C (hexane-CHCl3) [lit.,9 mp 79 °C]. 1H-NMR (400 MHz, CDCl3) δ: 6.72 (1H, d, J = 8.8 Hz, ArH), 6.47 (1H, d, J = 2.7 Hz, ArH), 6.35 (1H, dd, J = 8.6, 2.7 Hz, ArH), 5.39 (1H, s, ArOH), 3.81 (3H, s, ArOMe), 3.79 (3H, s, ArOMe).
3,4-Dibenzyloxyphenol (2c)
A mixture of THF (2 mL), 31% H2O2 (0.07 mL), H3BO3 (100 mg, 1.62 mmol), and conc. H2SO4 (0.032 mL) was stirred for 30 min at rt. To the mixture was added a solution of 3,4-dibenzyloxybenzaldehyde (102 mg, 0.320 mmol) in THF (1.5 mL). The mixture was stirred for 19 h at rt, then a saturated aqueous NaHCO3 solution was added. The mixture was extracted with CHCl3 and the organic layer was washed with brine. After drying with MgSO4 and evaporation, a residue was subjected to silica gel column chromatography with hexane-AcOEt (7:3) to give 2c (86.8 mg, 88%) as a colorless solid, mp 117.4-118.8 °C (hexane-CHCl3). 1H-NMR (400 MHz, CDCl3) δ: 7.44-7.28 (10H, m, ArH), 6.79 (1H, d, J = 8.6 Hz, ArH), 6.49 (1H, d, J = 3.0 Hz, ArH), 6.29 (1H, dd, J = 8.7, 2.8 Hz, ArH), 5.09 (2H, s, ArOCH2), 5.07 (2H, s, ArOCH2), 4.83 (1H, s, ArOH).
3,4-Methylenedioxyphenyl 2-iodo-4,6-dimethoxybenzoate (1a)
Under an N2 atmosphere, a solution of 2-iodo-4,6-dimethoxybenzoic acid3d (104 mg, 0.337 mmol), sesamol (50.7 mg, 0.367 mmol), EDC (90.9 mg, 0.474 mmol), and DMAP (40.7mg, 0.333 mmol) in dry CH2Cl2 (3 mL) was stirred for 1 h at rt. The mixture was washed with water and brine, then the organic layer was dried over MgSO4. After evaporation, the resulting residue was subjected to silica gel column chromatography with chloroform to give 1 (90.2 mg, 63%). Colorless prisms, mp 110.2-110.9 °C (hexane-CH2Cl2). IR (KBr) cm-1: 1742, 1593, 1562, 1503, 1478, 1459, 1438, 1408, 1309, 1287, 1243, 1220, 1188, 1169, 1144, 1114, 1097, 1068, 1023, 938, 926, 881, 841, 828, 807, 791, 597. 1H-NMR (400 MHz, CDCl3) δ: 6.95 (1H, d, J = 2.2 Hz, ArH), 6.84-6.81 (2H, t, ArH), 6.75 (1H, dd, J = 8.4, 2.3 Hz, ArH), 6.48 (1H, d, J = 1.9 Hz, ArH) 6.00 (2H, s, ArOCH2OAr), 3.85 (3H, s, ArOMe), 3.82 (3H, s, ArOMe). 13C-NMR (100 MHz, CDCl3) δ: 166.3, 162.0, 158.2, 148.1, 145.7, 145.2, 122.4, 115.4, 114.2, 108.1, 103.9, 101.8, 99.1, 92.9, 56.2, 55.9. Anal. Calcd for C16H13IO6: C, 44.88; H, 3.06. Found: C, 44.74; H, 2.94.
3,4-Dimethoxyphenyl 2-iodo-4,6-dimethoxybenzoate (1b)
In the same procedure mentioned above using 2b (52.8 mg, 0.343 mmol), 1b (63.4 mg, 44%) was obtained. Colorless prisms, mp 80.2-80.5 °C (hexane-CH2Cl2). IR (KBr) cm-1: 1749, 1598, 1562, 1510, 1455, 1255, 1228, 1182, 1151, 1124, 1076, 1030. 1H-NMR (400 MHz, CDCl3) δ: 6.95 (1H, d, J = 2.2 Hz, ArH), 6.87 (2H, d, J = 1.5 Hz, ArH), 6.84 (1H, t, J = 1.5 Hz), 6.47 (1H, d, J = 2.2 Hz, ArH) 3.88 (3H, s, ArOMe), 3.87 (3H, s, ArOMe), 3.83 (3H, s, ArOMe), 3.80 (3H, s, ArOMe). 13C-NMR (100 MHz, CDCl3) δ: 166.3, 161.9, 158.1, 149.5, 147.2, 144.6, 122.5, 115.4, 113.0, 111.3, 105.9, 99.0, 92.9, 56.3, 56.2, 56.1, 55.8. Anal. Calcd for C17H17IO6: C, 45.96; H, 3.86. Found: C, 45.84; H, 3.72.
3,4-Dibenzyloxyphenyl 2-iodo-4,6-dimethoxybenzoate (1c)
In the same procedure mentioned above using 2c (4.04 g, 13.2 mmol), 1c (4.87 g, 63%) was obtained. Colorless solid, mp 101.4-102.0 °C (Et2O-CH2Cl2). IR (KBr) cm-1: 1751, 1603, 1560, 1510, 1454, 1435, 1421, 1400, 1387, 1331, 1315, 1277, 1250, 1236, 1215, 1184, 1153, 1119, 1074, 1030, 1001, 989, 922, 883, 818, 804, 787, 770, 750, 731, 702, 594. 1H-NMR (400 MHz, CDCl3) δ: 7.47-7.29 (10H, m, ArH), 6.97-6.93 (3H, m, ArH), 6.84 (1H, dd, J = 8.7, 2.6 Hz, ArH), 6.47 (1H, d, J = 2.2 Hz, ArH), 5.18 (2H, s, ArOCH2), 5.16 (2H, s, ArOCH2), 3.82 (3H, s, ArOMe), 3.81 (3H, s, ArOMe). 13C-NMR (100 MHz, CDCl3) δ: 166.1, 161.9, 158.2, 149.6, 147.0, 145.1, 137.3, 137.0, 128.6, 128.6, 128.0, 127.9, 127.5, 127.5, 122.5, 115.6, 115.4, 114.0, 109.1, 99.1, 93.0, 72.0, 71.5, 56.2, 55.8. Anal. Calcd for C29H25IO6: C, 58.40; H, 4.23. Found: C, 58.62; H, 4.15.
Typical Procedure for the Coupling Reaction (Table 1)
Under an N2 atmosphere, a mixture of 1a (100 mg, 0.234 mmol), Pd(OAc)2 (0.023 mmol), ligand (0.047 mmol), base (0.023 mmol), and DMA (7 mL) was heated under reflux for 1 h with stirring. Unsolved materials were filtered off, then water was added to the filtrate. After extraction with CH2Cl2, the organic layer was washed with brine and dried over MgSO4. Evaporation of the solvent gave a residue which was subjected to silica gel column chromatography with hexane-AcOEt-CH2Cl2 (1:1:3).
2,3-Methylenedioxy-7,9-dimethoxy-6H-benzo[c]chromen-6-one (4a)
Colorless needles, mp 240.5-241.0 °C (hexane-CH2Cl2). 1H-NMR (400 MHz, CDCl3) δ: 7.25 (1H, s, ArH), 6.83 (1H, d, J = 2.2 Hz, ArH), 6.75 (1H, s, ArH), 6.48 (1H, d, J = 2.2 Hz, ArH), 6.04 (2H, s, ArOCH2OAr), 3.98 (3H, s, ArOMe), 3.96 (3H, s, ArOMe). 13C-NMR (100 MHz, CDCl3) δ: 165.5, 164.2, 158.1, 150.0, 148.1, 145.0, 139.7, 111.1, 102.9, 102.2, 101.2, 98.6, 98.3, 96.8, 56.5, 55.8. IR (KBr) cm-1: 1719, 1603, 1574, 1504, 1472, 1454, 1433, 1381, 1344, 1254, 1211, 1180, 1146, 1092, 1063, 1030, 1005, 827. Anal. Calcd for C16H12O6: C, 64.00; H, 4.03. Found: C, 63.85; H, 3.84.
1,2-Methylenedioxy-7,9-dimethoxy-6H-benzo[c]chromen-6-one (5a)
Colorless needles, mp 254.8-256.5 °C (hexane-CH2Cl2). 1H-NMR (400 MHz, CDCl3) δ: 7.58 (1H, d, J = 2.2 Hz, ArH), 6.91 (1H, d, J = 8.6 Hz, ArH), 6.80 (1H, d, J = 8.8 Hz, ArH), 6.56 (1H, d, J = 2.4 Hz, ArH), 6.16 (2H, s, ArOCH2OAr), 4.00 (3H, s, ArOMe), 3.95 (3H, s, ArOMe). 13C-NMR (100 MHz, CDCl3) δ: 165.5, 163.8, 157.9, 146.8, 144.0, 143.7, 137.2, 109.8, 109.0, 105.3, 103.4, 102.3, 101.8, 99.4, 56.5, 55.8. IR (KBr) cm-1: 1722, 1641, 1612, 1597, 1574, 1504, 1489, 1456, 1445, 1418, 1346, 1265, 1246, 1205, 1165, 1080, 1057, 1043, 1007, 986, 920, 839, 916. Anal. Calcd for C16H12O6: C, 64.00; H, 4.03. Found: C, 63.92; H, 3.96.
1,2,7,9-Tetramethoxy-6H-benzo[c]chromen-6-one (5b)
Colorless solid, mp 202.2-203.5 °C (hexane-CH2Cl2). 1H-NMR (400 MHz, CDCl3) δ: 8.33 (1H, d, J = 2.2 Hz, ArH), 7.07 (2H, s, ArH), 6.61 (1H, d, J = 2.4 Hz, ArH), 3.99 (3H, s, ArOMe), 3.96 (3H, s, ArOMe), 3.92 (3H, s, ArOMe), 3.91 (3H, s, ArOMe). 13C-NMR (100 MHz, CDCl3) δ: 165.4, 163.7, 157.9, 149.5, 147.3, 146.4, 138.5, 114.7, 112.7, 112.4, 104.1, 102.3, 99.6, 60.4, 56.7, 56.5, 55.6. IR (KBr) cm-1: 1724, 1601, 1587, 1570, 1497, 1474, 1456, 1443, 1414, 1337, 1298, 1267, 1246, 1229, 1213, 1204, 1163, 1090, 1072, 1059, 1024, 962, 837, 804. Anal. Calcd for C17H16O6: C, 64.55; H, 5.10. Found: C, 64.45; H, 5.05.
2,3-Dibenzyloxy-7,9-dimethoxy-6H-benzo[c]chromen-6-one (4c)
Colorless solid, mp 166.5-167.3 °C (hexane-CH2Cl2). 1H-NMR (400 MHz, CDCl3) δ: 7.49-7.30 (11H, m, ArH), 6.81 (1H, s, ArH), 6.72 (1H, d, J = 2.2 Hz, ArH), 6.42 (1H, d, J = 2.2 Hz, ArH), 5.20 (2H, s, ArOCH2), 5.18 (2H, s, ArOCH2), 3.95 (3H, s, ArOMe), 3.92 (3H, s, ArOMe). 13C-NMR (100 MHz, CDCl3) δ: 165.4, 164.2, 158.2, 152.0, 147.4, 145.4, 139.4, 137.2, 136.2, 128.8, 128.7, 128.3, 128.1, 127.6, 127.3, 110.2, 109.9, 103.0, 102.5, 98.1, 96.6, 72.8, 71.0, 56.4, 55.7. IR (KBr) cm-1: 1738, 1614, 1593, 1568, 1518, 1499, 1448, 1431, 1391, 1333, 1244, 1211, 1196, 1159, 1126, 1061, 1020, 1003, 924, 839, 824, 733, 694. Anal. Calcd for C29H24O6: C, 74.35; H, 5.16. Found: C, 74.47; H, 5.22.
1,2-Dibenzyloxy-7,9-dimethoxy-6H-benzo[c]chromen-6-one (5c)
Colorless solid, mp 184.4-186.1 °C (hexane-CH2Cl2). 1H-NMR (400 MHz, CDCl3) δ: 8.29 (1H, d, J = 2.4 Hz, ArH), 7.47-7.31 (10H, m, ArH), 7.14 (1H, d, J = 9.0 Hz, ArH), 7.06 (1H, d, J = 9.0 Hz, ArH), 6.55 (1H, d, J = 2.2 Hz, ArH), 5.16 (2H, s, ArOCH2), 5.08 (2H, s, ArOCH2), 3.96 (3H, s, ArOMe), 3.43 (3H, s, ArOMe). 13C-NMR (100 MHz, CDCl3) δ: 165.4, 163.5, 157.9, 148.7, 146.8, 138.7, 137.0, 136.7, 128.7, 128.6, 128.5, 128.4, 128.3, 127.8, 117.0, 113.2, 112.6, 104.1, 102.2, 100.2, 75.2, 72.1, 56.4, 55.3. IR (KBr) cm-1: 1742, 1601, 1572, 1501, 1474, 1456, 1443, 1427, 1371, 1337, 1290, 1261, 1252, 1215, 1196, 1167, 1049, 1022, 959, 800, 752, 731, 696. Anal. Calcd for C29H24O6: C, 74.35; H, 5.16. Found: C, 74.26; H, 5.13.
Altertenuol (2,3,7-Trihydroxy-9-methoxy-6H-benzo[c]chromen-6-one) (6)
Under N2 atmosphere, to a solution of 4c (50.1 mg, 0.107 mmol) in CH2Cl2 (3.5 mL) was added BBr3 (1M CH2Cl2 solution, 0.320 mL, 0.320 mmol) at 0 °C. The mixture was stirred for 1 h at rt, and quenched with water. After extraction with AcOEt, the organic layer was washed with brine and dried over MgSO4 to give a residue which was subjected to silica gel column chromatography with AcOEt. A colorless solid of 1 (27.9 mg, 95%) was obtained, mp 283.9-285.8 °C (acetone) [lit.,7 mp 284-285 °C]. 1H-NMR (400 MHz, CD3OD) δ: 7.38 (1H, s, ArH), 6.94 (1H, d, J = 2.4 Hz, ArH), 6.74 (1H, s, ArH), 6.50 (1H, d, J = 2.2 Hz, ArH), 3.93 (3H, s, ArOMe). 13C-NMR (100 MHz, DMSO-d6) δ: 166.8, 165.1, 163.8, 149.3, 144.4, 143.8, 137.6, 109.1, 109.0, 103.5, 100.0, 98.6. 98.0, 56.1. IR (KBr) cm-1: 3535, 3285, 3105, 1647, 1607, 1568, 1528, 1510, 1456, 1431, 1279, 1227, 1202, 1165. Anal. Calcd for C14H10O6: C, 61.32; H, 3.68. Found: C, 61.26; H, 3.69.
2,3-Dibenzyloxy-7-hydroxy-9-methoxy-6H-benzo[c]chromen-6-one (7)4a
Under N2 atmosphere, to a solution of 4c (102 mg, 0.218 mmol) in a mixed solvent of CH2Cl2 (4.7 mL) and MeCN (1.4 mL) was added AlCl3 (69.6 mg, 0.522 mmol) and NaI (83.3 mg, 0.556 mmol) at 0 °C. The mixture was stirred for 4.5 h at rt, and quenched with water. After extraction with CH2Cl2, the organic layer was washed with brine and dried over MgSO4 to give a residue which was subjected to silica gel column chromatography with hexane-AcOEt (4:3). Colorless solid of 7 (69.9 mg, 71%) was obtained, mp 211.3-212.5 °C (AcOEt) [lit.,4a mp 199-200 °C]. 1H-NMR (400 MHz, CDCl3) δ: 11.5 (1H, s, ArOH), 7.49-7.32 (11H, m, ArH), 6.88 (1H, s, ArH), 6.75 (1H, d, J = 2.2 Hz, ArH), 6.50 (1H, d, J = 2.2 Hz, ArH), 5.22 (4H, s, ArOCH2), 3.91 (3H, s, ArOMe).
Conversion of 7 into 6
Under H2 atmosphere, a mixture of 7 (53.6 mg, 0.118 mmol), 10% Pd/C (12.1 mg), and THF (4 mL) was vigorously stirred for 19 h at rt. After filtration and washing the filter paper with CHCl3-MeOH (10:1), the filtrate was concentrated. An obtained residue was purified by silica gel column chromatography with AcOEt to give 6 (31.6 mg, 97%).
ACKNOWLEDGMENT
A part of this work was financially supported by JSPS KAKENHI Grant No. 22590003 and the Tamura Science and Technology Foundation.
References
1. (a) D. E. Ames and A. Opalko, Tetrahedron, 1984, 40, 1919; CrossRef For reviews, see: (b) G. Bringmann, M. Breuning, and S. Tasler, Synthesis, 1999, 525; CrossRef (c) J. Hassan, M. Sévignon, C. Gozzi, E. Schulz, and M. Lemaire, Chem. Rev., 2002, 102, 1359; CrossRef (d) G. Bringmann, A. J. P. Mortimer, P. A. Keller, M. J. Gresser, J. Garner, and M. Breuning, Angew. Chem. Int. Ed., 2005, 44, 5384; CrossRef (e) D. Alberico, M. E. Scott, and M. Lautens, Chem. Rev., 2007, 107, 174; CrossRef (f) G. Bringmann, T. Gulder, T. A. M. Gulder, and M. Breuning, Chem. Rev., 2011, 111, 563; CrossRef (g) J. Yamaguchi, A. D. Yamaguchi, and K. Itami, Angew. Chem. Int. Ed., 2012, 51, 8960. CrossRef
2. (a) T. Matsumoto, T. Hosoya, and K. Suzuki, J. Am. Chem. Soc., 1992, 114, 3568; CrossRef (b) T. Hosoya, E. Takashiro, T. Matsumoto, and K. Suzuki, J. Am. Chem. Soc., 1994, 116, 1004; CrossRef (c) T. Harayama, H. Yasuda, T. Akiyama, Y. Takeuchi, and H. Abe, Chem. Pharm. Bull., 2000, 48, 861; CrossRef (d) G. Bringmann and D. Menche, Acc. Chem. Res., 2001, 34, 615; CrossRef (e) G. Bringmann, M. Breuning, R.-M. Pfeifer, W. A. Schenk, K. Kamikawa, and M. Uemura, J. Organomet. Chem., 2002, 661, 31; CrossRef (f) G. Bringmann, S. Tasler, R.-M. Pfeifer, and M. Breuning, J. Organomet. Chem., 2002, 661, 49; CrossRef (g) G. A. Molander, K. M. George, and L. G. Monovich, J. Org. Chem., 2003, 68, 9533; CrossRef (h) H. Abe, S. Takeda, T. Fujita, K. Nishioka, Y. Takeuchi, and T. Harayama, Tetrahedron Lett., 2004, 45, 2327; CrossRef (i) H. Abe, K. Nishioka, S. Takeda, M. Arai, Y. Takeuchi, and T. Harayama, Tetrahedron Lett., 2005, 46, 3197; CrossRef (j) S. Takeda, H. Abe, Y. Takeuchi, and T. Harayama, Tetrahedron, 2007, 63, 396; CrossRef (k) H. Abe, Y. Sahara, Y. Matsuzaki, Y. Takeuchi, and H. Abe, Tetrahedron Lett., 2008, 49, 605; CrossRef (l) H. Abe and T. Harayama, Heterocycles, 2008, 75, 1305; CrossRef (m) K. Shioe, Y. Takeuchi, T. Harayama, and H. Abe, Chem. Pharm. Bull., 2010, 58, 435; CrossRef (n) K. Shioe, Y. Sahara, Y. Horino, T. Harayama, Y. Takeuchi, and H. Abe, Tetrahedron, 2011, 67, 1960. CrossRef
3. (a) T. Harayama, Y. Kawata, C. Nagura, T. Sato, T. Miyagoe, H. Abe, and Y. Takeuchi, Tetrahedron Lett., 2005, 46, 6091; CrossRef (b) T. Harayama, A. Hori, H. Abe, and Y. Takeuchi, Heterocycles, 2006, 67, 385; CrossRef (c) H. Nishioka, C. Nagura, H. Abe, Y. Takeuchi, and T. Harayama, Heterocycles, 2006, 70, 549; CrossRef (d) H. Abe, T. Fukumoto, Y. Takeuchi, and T. Harayama, Heterocycles, 2007, 74, 265; CrossRef (e) T. Harayama, M. Asai, T. Miyagoe, H. Abe, Y. Takeuchi, A. Yamaguchi, and S. Fujii, Heterocycles, 2010, 81, 1881; CrossRef (f) T. Harayama, C. Nagura, T. Miyagoe, Y. Kawata, H. Abe, and Y. Takeuchi, Heterocycles, 2010, 81, 2609; CrossRef (g) H. Abe, T. Fukumoto, Y. Horino, T. Harayama, and Y. Takeuchi, Heterocycles, 2010, 82, 851; CrossRef (h) H. Abe, T. Matsukihira, T. Fukumoto, Y. Horino, Y. Takeuchi, and T. Harayama, Heterocycles, 2012, 84, 323; CrossRef (i) K. Maeda, T. Matsukihira, S. Saga, Y. Takeuchi, T. Harayama, Y. Horino, and H. Abe, Heterocycles, 2014, 88, 621. CrossRef
4. (a) K. Kuramochi, K. Fukudome, I. Kuriyama, T. Takeuchi, Y. Sato, S. Kamisuki, K. Tsubaki, S. Sugawara, H. Yoshida, and Y. Mizushina, Bioorg. Med. Chem., 2009, 17, 7227; CrossRef (b) G. Nemecek, J. Cudaj, and J. Podkech, Eur. J. Org. Chem., 2012, 3863. CrossRef
5. For Xphos, see: K. Billingsley and S. L. Buchwald, J. Am. Chem. Soc., 2007, 129, 3358. CrossRef
6. (a) D. Garcia-Cuadrado, A. A. C. Braga, F. Maseras, and A. M. Echavarren, J. Am. Chem. Soc., 2006, 128, 1066; CrossRef (b) D. Garcia-Cuadrado, P. de Mendoza, A. A. C. Braga, F. Maseras, and A. M. Echavarren, J. Am. Chem. Soc., 2007, 129, 6880. CrossRef
7. (a) T. Rosett, R. H. Sankhala, C. E. Stickings, M. E. U. Taylor, and R. Thomas, Biochem. J., 1957, 67, 390; (b) R. Thomas, Biochem. J., 1961, 80, 234.
8. M. Node, T. Kajimoto, K. Nishide, E. Fujita, and K. Fuji, Bull. Inst. Chem. Res., Kyoto Univ., 1992, 70, 308.
9. A. Roy, K. R. Reddy, P. K. Mohanta, H. Ila, and H. Junjappa, Synth. Commun., 1999, 29, 3781. CrossRef