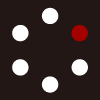
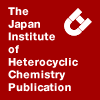
HETEROCYCLES
An International Journal for Reviews and Communications in Heterocyclic ChemistryWeb Edition ISSN: 1881-0942
Published online by The Japan Institute of Heterocyclic Chemistry
e-Journal
Full Text HTML
Received, 9th October, 2013, Accepted, 30th October, 2013, Published online, 31st October, 2013.
DOI: 10.3987/COM-13-12860
■ Synthetic Exploitation of Halogenated Alkenes Containing Electron-Withdrawing Group (EWG): Access to Valuable 2,4-Dinitrothiophenes via Ring-Closing and Ring-Opening/Ring-Closing Protocols
Amac Fatih Tuyun*
Department of Chemical Engineering, Engineering and Architecture Faculty, Beykent University, 34396, Turkey
Abstract
With the goal of their exploitation for the synthesis of highly functionalized 2,4-dinitrothiophenes, 2-(2,3-dichloro-1,3-dinitroallylidene)-1,3-dithiolane (2a), derived from the initial ring-closing of polyhalogenated nitrobutadienic building blocks with 1,2-ethanedithiol, were reacted with secondary amines. After protic work up, highly functionalized 2,4-dinitrothiophenes have been accomplished via ring-opening/ring-closing protocols.INTRODUCTION
Electron-deficient compounds, such as polyhalogenated butadienic building blocks, react promptly with neutral or anionic nucleophiles in mild or harsh conditions in versatile reactions ranging from nucleophilic vinylic substitution (SNVin)1 pathway via the addition-elimination route2 to [4+2] cycloaddition reactions.3 The multifaceted chemical behavior of polyhalogenated butadienic building blocks put in our hands a nice polyfunctionalized fragment, whose wide reactivity has been deeply studied that develops convenient synthetic accesses to versatile homo(hetero)cyclic and linear compounds.4 Approaches to the preparation of nitro substituted five and six membered heterocycles comprise: (i) ring construction5 i.e. 4-nitrothiophenes, pyrimidines, pyrazoles and (ii) functional group transformations6 by using polyhalogenated butadienic building blocks. Nitro- and dinitrothiophenes have previously been described in the literature7 and used as scaffolds8 for preparing a range of antitumor, antiproliferative, antifungal, and antibacterial compounds.9
Within the frame of a long-standing research project on the synthetic exploitability of the highly functionalized versatile polyhalogenated nitrobutadienic building blocks with different nucleophiles, in the last few years we have reported a number of successful applications of the highly functionalized polyhalogenated nitro-10 and dinitrobutadienes,11 respectively. Driven by the promising stepwise synthesis of the highly functionalized 2,4-dinitrothiophenes (4a-d) and with the above mentioned hints to biological activities in mind, we set out to develop structural conjunctions of the nitrothiophenes. The pivotal step to access 2,4-dinitrothiophenes is started by synthesizing the 1,1,3,4-tetrachloro-2,4- dinitrobuta-1,3-diene (1) easily accessible than related compounds in three steps starting from radical dimerization of trichloroethylene, subsequently dechlorination by zinc in ethanol; it was prepared for the first time in 1998 by means of direct nitration of this intermediate 1,1,3,4-tetrachlorobuta-1,3-diene in the presence of phosphoric acid, gave the desired product 1 as published by Potkin and Nechai et al.12 The regioselectivity is caused by the fact that the LUMO of nitrodienes is located preferentially at the dihalogenonitrovinyl moiety.13
RESULTS AND DISCUSSION
As expected, the preparation of heterocyclic compounds by applying dinitrobuta-1,3-diene (1) as the electrophilic substrate for dithiols is possible. Therefore, introduction of dithiols such as 1,2-ethanedithiol leads to the cyclic 1,1-disubstituted heterocycles, 2-(2,3-dichloro-1,3-dinitroallylidene)-1,3-dithiolane (2).11 During the studies of polyhalogenated butadienic building blocks toward the design of thiophenes, we have decided to use 2. As it clearly shows up from Scheme 1, the significance to the synthesis of 4a-d is provided after proper modifications of the functionalities of the resulting 1,3-dithiolane (2) in generally by introducing an amino group. It is interesting to note that the amine attack on 2 is regioselectively directed by second nitro group as an electron-withdrawing group to the C-3 atom. The replacement of the secondary amino group with a chloro atom in 2 has been accomplished by means of a procedure already applied10,11 by us with success to nitro- and dinitrobutadienes derived from ring-closing of 1 with bifunctional nucleophiles.
In particular, it should be noted that the ring-opening/ring-closing of 3 effectively occurs in the presence of sodium hydroxide, leading to the appealingly highly functionalized 2,4-dinitrothiophenes. Ring-opening of 1,3-dithiolane (3) is initiated upon addition of a base (40% aqueous sodium hydroxide in water) in dimethyl sulfoxide (DMSO) in cold condition within 1 to 3 h (Table 1). From a mechanistic point of view, initially, deprotonation of methylene group in active methylene carbon adjacent to the sulfur atom in 3a-d leads to ring-opening and generates the nucleophilic thiolate from 2-(2-organylamino-3-chloro-1,3-dinitroallylidene)-1,3-dithiolanes (3) that, finally, attacks the C-4 atom of the dinitrobutadienic system. Parallel of withdrawing of a chloride anion, the mechanism sequence completes in a ring-closing of these highly functionalized 2,4-dinitrothiophenes. A plausible mechanism is shown in Scheme 2.
To our delight, it is noteworthy to say that one-pot reaction is also performed starting from the polyhalogenated nitrobutadienic building block (2), i.e., with piperidine as the organic nucleophile, the compound 3a has been generated at 30 °C in DMSO. After 2 h, the mixture is cooled to 0 °C and treated with aqueous sodium hydroxide to initiate the ring-closing step in 67% yields. Treatment in basic medium (NaOH/DMSO/H2O) led to a carbanion on the dithiolane ring, followed by ring opening, formation of RS- sulfur anion and then, intramolecular cyclization into fully substituted 2,4-dinitrothiophenes. The synthesis can be also realized as a two-step one pot reaction.
CONCLUSIONS
Finally, thanks to the both nitro groups at highly functionalized nitrobutadienic building blocks in 1 for presenting us an opportunity to pave the way to functionalized 2,4-dinitrothiophenes. The results herein enlighten an original and attractive access to the 2,4-dinitrothiophenes, which originates from the ring-closing and ring-opening/ring-closing of functionalized dinitrobutadienic building block (1) whose key point is represented by two nitro groups serving activating functionality of chloro atoms on C-1 and C-3 to facilitate the synthesis of dinitrothiophenes, easily. Additionally, a straightforward one-pot reaction procedure for the preparation of highly functionalized 2,4-dinitrothiophenes was developed. Due to their high functionality, these 5-sulfanyl substituted 3-amino-2,4-dinitrothiophenes are valuable building block for the predictable synthesis of bioactive heterocycles with high synthetical potential.
EXPERIMENTAL
All reagents were commercially obtained commercial supplier and used without further purification unless otherwise noted. All solvents used in the reactions were distilled from appropriate drying agents prior to use. Petroleum ether had a boiling range 60-70 °C. Analytical thin layer chromatography (TLC) was purchased from Merck KGaA (silica gel 60 F254) based on Merck DC-plates (aluminum based). Visualization of the chromatogram was performed by UV light (254 nm). Column chromatographic separations were carried out using silica gel 60 (Merck, 63-200 μm particle sized, 60-230 mesh). Melting points were determined with an Electrothermal IA9000 series and were uncorrected. 1H NMR and 13C NMR spectra were recorded with Varian UNITY INOVA spectrometers with 500 MHz frequency for 1H and 125 MHz frequency for 13C NMR in ppm (δ) relative to tetramethylsilane (TMS) with the solvent resonance employed as the internal standard. 1H NMR spectra and 13C NMR spectra in CDCl3 refer to the solvent signal center at δ 7.26 and δ 77.0 ppm, respectively. Data are reported as follows: chemical shift, multiplicities are declared as follows: s (singlet), brs (broad singlet), d (doublet), dd (doublet of doublets), t (triplet), m (multiplet). Coupling constants J are given in Hz. IR spectra were recorded as ATR on Perkin Elmer Spectrum 100 UATR FTIR spectrometer. Microanalyses were carried out with a Carlo Erba Elemental Analyzer 1106. Mass spectra were obtained on a Thermo Finnigan LCQ Advantage MAX MS/MS spectrometer equipped with an ESI (Electrospray ionization) sources. Nitrogen was used as the nebulizing gas. In the MS1 experiment, helium was used as collision gas.
Ring-Closing Reaction of 1
At 0 °C, a solution of 1,1,3,4-tetrachloro-2,4-dinitrobuta-1,3-diene (1) and 1,2-ethanedithiol were stirred 8 h without a solvent. After this period, the addition of petroleum ether and stirring for additional 10 min, the precipitate was collected and washed successive with water, aqueous sodium hydrogen carbonate, again water, and then petroleum ether. After drying in vacuo, 2 was obtained in 80% (48%);11 mp 100.5-101.5 °C.
2-(2,3-Dichloro-1,3-dinitroallylidene)-1,3-dithiolane (2)11a
Synthesized as yellow crystal. Rf 0.48 (PET:CHCl3 = 1:1). IR (ATR) υ (cm-1): 2923, 2851 (CHaliphatic), 1439 (C=C), 1287, 1515 (NO2). UV-Vis (CHCl3): λmax 394 nm. 1H NMR (500 MHz, CDCl3) δ: 3.46-3.53 (m, 4H, (CH2)2). 13C NMR (125 MHz, CDCl3) δ: 37.91, 40.09 (CH2), 121.15, 129.74, 130.71, 168.14 (Cbutadien). MS (ESI+): m/z (%) 305 (100, [M+H]+). GC-MS (EI): m/z (%) 221 ([M-Cl-NO2]+, 38), 182 ([M-2Cl-NO2]+, 54), 140 ([M-2Cl-2NO2]+, 70), 128 ([M-2Cl-2NO2-Cl]+, 36). Anal. Calcd for C6H4Cl2N2O4S2 (303.143) (%): C, 23.77; H, 1.33; N, 9.24; S, 21.16. Found (%): C, 23.45; H, 1.23; N, 9.57; S, 21.53.
General Procedure of Proper Modifications of 2 with Amines (3a-d)
A suspension of dithiolane (2) in 25 mL CH2Cl2 was added to a solution of amine in 10 mL CH2Cl2 with five-fold excess at 0 °C. The mixture was stirred at room temperature for the required reaction time, according to TLC (typically 30 min). After stirring for reaction time, CHCl3 or CH2Cl2 was added to the reaction mixture. The organic layer was separated and washed with water (3 x 20 mL) and dried with CaCl2 or MgSO4. After evaporation of the solvent, the crude product was isolated as viscous oil after purification by column chromatography on silica gel.
2-(2-(Piperidin-1-yl)-3-dichloro-1,3-dinitroallylidene)-1,3-dithiolane (3a)
Following the general procedure as dark red oil, using 2 (0.74 g, 2.44 mmol) and piperidine (1.05 g, 12.8 mmol), 0.71 g, 83%. Rf 0.17 (PET:CHCl3 = 2:1). IR (ATR) υ (cm-1): 2930, 2850 (CHaliphatic), 1451 (C=C), 1219, 1518 (NO2). 1H NMR (500 MHz, CDCl3) δ: 1.33-1.46 (m, 2H, NCH2CH2CH2piperidine), 1.46-1.54 (m, 4H, NCH2CH2piperidine), 2.92 (brs, 4H, NCH2piperidine), 3.42-3.53 (m, 4H, (SCH2)2dithiolane). 13C NMR (125 MHz, CDCl3) δ: 23.19 (NCH2CH2CH2piperidine), 25.05 (NCH2CH2piperidine), 37.91, 40.09 ((SCH2)2dithiolane), 49.67 (NCH2piperidine), 110.20 (C1), 119.95 (C2), 139.03 (C3), 168.82 (C4). MS (ESI+): m/z (%) 305 (81, [M-NO2]+). Anal. Calcd for C11H14ClN3O4S2 (351.830) (%): C, 37.55; H, 4.01; N, 11.94; S, 18.23. Found (%): C, 37.75; H, 4.23; N, 11.56; S, 18.53.
2-(2-(Morpholin-4-yl)-3-dichloro-1,3-dinitroallylidene)-1,3-dithiolane (3b)
Following the general procedure as red oil, using 2 (0.89 g, 2.94 mmol) and morpholine (1.347 g, 15.46 mmol), 0.82 g, 79%. Rf 0.14 (PET:CHCl3 = 1:1). IR (ATR) υ (cm-1): 2961, 2917, 2850 (CHaliphatic), 1454 (C=C), 1223, 1515 (NO2). 1H NMR (500 MHz, CDCl3) δ: 2.95-3.02 (m, 4H, OCH2morpholine), 3.45-3.54 (m, 4H, (SCH2)2dithiolane), 3.64 (t, 3J = 4.88 Hz, 4H, NCH2morpholine). 13C NMR (125 MHz, CDCl3) δ: 37.82, 40.33 ((SCH2)2dithiolane), 49.67 (NCH2morpholine), 49.76 (NCH2), 67.23 (OCH2), 113.78 (C1), 131.02 (C2), 139.31 (C3), 170.63 (C4). MS (ESI+): m/z (%) 355 (75, [M+2H]+). Anal. Calcd for C10H12ClN3O5S2 (353.802) (%): C, 33.95; H, 3.42; N, 11.88; S, 18.13. Found (%): C, 33.77; H, 3.33; N, 11.62; S, 18.45.
2-(2-(Thiomorpholin-4-yl)-3-dichloro-1,3-dinitroallylidene)-1,3-dithiolane (3c)
Following the general procedure as red oil, using 2 (0.62 g, 2.04 mmol) and thiomorpholine (1.110 g, 10.73 mmol), 0.62 mg, 75%. Rf 0.13 (PET:CHCl3 = 1:1). IR (ATR) υ (cm-1): 2910, 2835 (CHaliphatic), 1454 (C=C), 1221, 1515 (NO2). 1H NMR (500 MHz, CDCl3) δ: 2.59 (t, 3J = 4.88 Hz, 4H, SCH2thiomorpholine), 3.20 (s, 4H, NCH2thiomorpholine), 3.42-3.56 (m, 4H, (SCH2)2dithiolane). 13C NMR (125 MHz, CDCl3) δ: 28.14 (SCH2thiomorpholine), 37.87, 40.26 ((SCH2)2dithiolane), 52.25 (NCH2thiomorpholine), 114.10 (C1), 130.52 (C2), 140.03 (C3), 170.49 (C4). MS (ESI+): m/z (%) 335 (16, [M-NO2]+). Anal. Calcd for C10H12ClN3O4S3 (369.868) (%): C, 32.47; H, 3.27; N, 11.36; S, 26.01. Found (%): C, 32.56; H, 3.13; N, 11.12; S, 25.85.
2-(2-[4-(4-Fluorophenyl)piperazin-1-yl]-3-dichloro-1,3-dinitroallylidene)-1,3-dithiolane (3d)
Following the general procedure as dark red oil, using 2 (0.73 g, 2.41 mmol) and (4-fluorophenyl)piperazine (2.28 g, 12.68 mmol), 0.77 g, 69%. Rf 0.18 (PET:CHCl3 = 3:1). IR (ATR) υ (cm-1): 3053 (CHaromatic), 2956, 2827 (CHaliphatic), 1450 (C=C), 1267, 1518 (NO2). 1H NMR (500 MHz, CDCl3) δ: 3.04 (t, 3J = 4.88 Hz, 4H, NCH2piperazine), 3.14 (brs, 4H, NCH2piperazine), 3.46-3.52 (m, 4H, (SCH2)2dithiolane), 6.77-6.94 (m, 4H, CHaromatic). 13C NMR (125 MHz, CDCl3) δ: 36.64, 39.08 ((SCH2)2dithiolane), 48.21, 49.61 (NCH2piperazine), 114.45, 117.30 (CHaromatic), 146.90, 155.40, 157.31 (Caromatic), 112.30 (C1), 129.90 (C2), 138.01 (C3), 169.42 (C4). MS (ESI+): m/z (%) 400 (100, [M-NO2]+). Anal. Calcd for C16H16ClFN4O4S2 (446.904) (%): C, 43.00; H, 3.61; N, 12.54; S, 14.35. Found (%): C, 43.37; H, 3.27; N, 12.29; S, 14.77.
General Procedure of 2,4-Dinitrothiophenes (4a-d)
Within 10 min at 0 °C, 0.50 g of an aqueous solution of sodium hydroxide in water (40%, 5.0 mmol) was added to solution of 1.00 mmol amino substituted dithiolane (3a-d) dissolved in 10 mL DMSO. After 1 h at 0 °C, the mixture was stirred for additional 2 h at room temperature. Then, cold water (30 mL) was added at 5 °C. The mixture was acidified to pH 1 upon dropwise addition of 5% hydrochloric acid. The product precipitated as oil, and the aqueous fraction was decanted. The oily product was dissolved in CHCl3 (50 mL), the solution was washed with aqueous HCl (10%, 3 x 30 mL) and water (2 x 30 mL), and was then dried over Na2SO4. After evaporation of the solvent, the product was isolated as viscous oil after purification by column chromatography on silica gel.
3-(Piperidin-1-yl)-2,4-dinitro-5-vinylsulfanylthiophene (4a)
Following the general procedure as orange oil, yield: 87%. Rf 0.54 (PET:CHCl3 = 2:1). IR (ATR) υ (cm-1): 3100 (CHaromatic), 2960, 2935, 2847 (CHaliphatic), 1308, 1537 (NO2), 1482, 1413, 1377. 1H NMR (500 MHz, CDCl3) δ: 1.47-1.54 (m, 2H, NCH2CH2CH2piperidine), 1.56 (m, 4H, NCH2CH2piperidine), 3.04 (t, 3J = 5.37 Hz, 4H, NCH2piperidine), 5.69 (d, 2J = 9.27 Hz, 1H, =CH2cis), 5.75 (d, 2J = 16.11 Hz, 1H, =CH2trans), 6.45 (dd, 3J = 9.27, 16.59 Hz, 1H, =CH-S). 13C NMR (125 MHz, CDCl3) δ: 23.01 (NCH2CH2CH2piperidine), 25.50 (NCH2CH2piperidine), 50.37 (NCH2piperidine), 123.99 (=CH2), 126.36 (=CH-S), 117.27, 137.72, 140.05, 141.46 (Cthiophene). MS (ESI+): m/z (%) 315 (36, [M]+), 313 (40, [M-2H]+), 318 (52, [M+2H]+). Anal. Calcd for C11H13N3O4S2 (315.369) (%): C, 41.89; H, 4.15; N, 13.32; S, 20.33. Found (%): C, 41.56; H, 4.33; N, 13.49; S, 20.67.
3-(Morpholin-4-yl)-2,4-dinitro-5-vinylsulfanylthiophene (4b)
Following the general procedure as orange oil, yield: 89%. Rf 0.43 (PET:CHCl3 = 2:1). IR (ATR) υ (cm-1): 3103 (CHaromatic), 2918, 2849 (CHaliphatic), 1270, 1526 (NO2), 1497, 1355, 1294. 1H NMR (500 MHz, CDCl3) δ: 3.32-3.36 (m, 4H, NCH2morpholine), 3.41-3.48 (m, 4H, OCH2morpholine), 5.82 (d, 2J = 8.79 Hz, 1H, =CH2cis), 5.90 (d, 2J = 16.11 Hz, 1H, =CH2trans), 6.53 (dd, 3J = 8.79, 16.11 Hz, 1H, =CH-S). 13C NMR (125 MHz, CDCl3) δ: 43.82 (NCH2morpholine), 62.78 (OCH2morpholine), 123.28 (=CH2), 127.54 (=CH-S), 110.00, 138.72, 140.12, 145.26 (Cthiophene). MS (ESI+): m/z (%) 341 (81, [M+Na]+). Anal. Calcd for C10H11N3O5S2 (317.341) (%): C, 37.85; H, 3.49; N, 13.24; S, 20.21. Found (%): C, 37.89; H, 3.55; N, 13.59; S, 20.31.
3-(Thiomorpholin-4-yl)-2,4-dinitro-5-vinylsulfanylthiophene (4c)
Following the general procedure as yellow oil, yield: 91%. Rf 0.23 (PET:CHCl3 = 1:1). IR (ATR) υ (cm-1): 3113 (CHaromatic), 2918, 2849 (CHaliphatic), 1284, 1541 (NO2), 1465, 1381, 1284. 1H NMR (500 MHz, CDCl3) δ: 2.76 (t, 3J = 5.37 Hz, 4H, SCH2thiomorpholine), 3.15 (t, 3J = 5.37 Hz, 4H, NCH2thiomorpholine), 5.74 (d, 2J = 9.27 Hz, 1H, =CH2cis), 5.83 (d, 2J = 16.59 Hz, 1H, =CH2trans), 6.56 (dd, 3J = 9.27, 16.59 Hz, 1H, =CH-S). 13C NMR (125 MHz, CDCl3) δ: 28.68 (SCH2thiomorpholine), 53.87 (NCH2thiomorpholine), 124.63 (=CH2), 126.39 (=CH-S), 104.54, 135.02, 141.01, 144.88 (Cthiophene). MS (ESI+): m/z (%) 289 (100, [M-NO2]+). Anal. Calcd for C10H11N3O4S3 (333.407) (%): C, 36.02; H, 3.33; N, 12.60; S, 28.85. Found (%): C, 36.32; H, 3.41; N, 12.78; S, 29.07.
3-[4-(4-Fluorophenyl)piperazin-1-yl]-2,4-dinitro-5-vinylsulfanylthiophene (4d)
Following the general procedure as dark red oil, yield: 77%. Rf 0.18 (PET:CHCl3 = 1:1). IR (ATR) υ (cm-1): 3048 (CHaromatic), 2956, 2830 (CHaliphatic), 1228, 1521 (NO2), 1449, 1320. 1H NMR (500 MHz, CDCl3) δ: 3.11-3.15 (m, 4H, NCH2piperazine), 3.27-3.33 (m, 4H, NCH2piperazine), 5.74 (d, 2J = 8.79 Hz, 1H, =CH2cis), 5.80 (d, 2J = 16.59 Hz, 1H, =CH2trans), 6.47 (dd, 3J = 8.79, 16.11 Hz, 1H, =CH-S), 6.80-6.94 (m, 4H, CHaromatic). 13C NMR (125 MHz, CDCl3) δ: 45.25, 49.17 (NCH2piperazine), 125.19 (=CH2), 125.83 (=CH-S), 115.05, 117.77 (CHaromatic), 146.48, 154.92, 156.03 (Caromatic), 101.71, 134.20, 142.09, 145.21 (Cthiophene). MS (ESI+): m/z (%) 435 (94, [M+Na]+). Anal. Calcd for C16H15FN4O4S2 (410.443) (%): C, 46.82; H, 3.68; N, 13.65; S, 15.62. Found (%): C, 46.44; H, 3.79; N, 13.92; S, 15.43.
Synthesis of 2,4-Dinitrothiophenes via One-pot Reaction (4a)
A suspension of dithiolane (2) in 25 mL DMSO was added to a solution of piperidine in 10 mL DMSO with five-fold excess of 2 at 30 °C. The mixture was stirred at that temperature for the required reaction time, according to TLC (1 h). After stirring for 1 h, the mixture is cooled, over a period of 10 min at 0 °C, treated with aqueous sodium hydroxide in water (40%) with five-fold excess of 2 to initiate the ring-opening/ring-closing. The mixture was acidified to pH 1 upon dropwise addition of 5% hydrochloric acid. CHCl3 (40 mL) was added to the reaction mixture. The organic layer was separated and washed with water (3 x 20 mL) and dried with CaCl2 or MgSO4. After evaporation of the solvent, the crude product was isolated viscous oil after purification by column chromatography on silica gel in 67% yields.
ACKNOWLEDGEMENTS
The author thanks the Board of Trustees of Beykent University for supplying the equipment and materials.
References
1. (a) L. Leroyer, V. Maraval, and R. Chauvin, Chem. Rev., 2012, 112, 1310; CrossRef (b) Z. Fang, H. Yuan, Y. Liu, Z. Tong, H. Li, J. Yang, B.-D. Barry, J. Liu, P. Liao, J. Zhang, Q. Liu, and X. Bi, Chem. Commun., 2012, 48, 8802; CrossRef (c) A. Rahimi, M. Gjikaj, and A. Schmidt, Synlett, 2009, 2583; CrossRef (d) A. Schmidt, A. Rahimi, and M. Gjikaj, Synthesis, 2009, 2371; CrossRef (e) C. Ibis and H. Yildirim, Phosphorus, Sulfur Silicon Relat. Elem., 2009, 184, 369; CrossRef (f) C. Ibis and N. Y. Onul, Rev. Roum. Chim., 2007, 52, 953; (g) C. Ibis and N. Onul, Phosphorus, Sulfur Silicon Relat. Elem., 2006, 181, 2411. CrossRef
2. (a) Z. Wrobel and A. Kwast, Synthesis, 2007, 259; CrossRef (b) S. Chiba, K. Ando, and K. Narasaka, Synlett, 2009, 2549. CrossRef
3. (a) M. Nawaz, M. Sher, and P. Langer, Synlett, 2010, 2383; CrossRef (b) V. A. Zapol’skii, R. Fischer, J. C. Namyslo, and D. E. Kaufmann, Bioorg. Med. Chem., 2009, 17, 4206; CrossRef (c) V. A. Zapol’skii, J. C. Namyslo, M. Gjikaj, and D. E. Kaufmann, Z. Naturforsch., 2010, 65b, 843; (d) V. A. Zapol’skii, J. C. Namyslo, B. Blaschkowski, and D. E. Kaufmann, Synlett, 2006, 3464; CrossRef (e) E. Block, F. Tries, C. He, C. Guo, M. Thiruvazhi, and P. J. Toscano, Org. Lett., 2003, 5, 1325. CrossRef
4. (a) V. A. Zapol’skii, J. C. Namyslo, M. Gjikaj, and D. E. Kaufmann, ARKIVOC, 2007, i, 76; CrossRef (b) N. Onul, W. M. D. Brimo, and C. Ibis, Phosphorus, Sulfur Silicon Relat. Elem., 2011, 186, 2180; CrossRef (c) C. Ibis and H. Yildirim, Phosphorus, Sulfur Silicon Relat. Elem., 2011, 186, 2236. CrossRef
5. (a) V. A. Zapol’skii, J. C. Namyslo, A. de Meijere, and D. E. Kaufmann, Beilstein J. Org. Chem., 2012, 8, 621; CrossRef (b) E. Nutz, V. A. Zapol’skii, and D. E. Kaufmann, Synthesis, 2009, 2719; CrossRef (c) V. A. Zapol’skii, J. C. Namyslo, C. Altug, M. Gjikaj, and D. E. Kaufmann, Synthesis, 2008, 304; CrossRef (d) V. A. Zapol’skii, E. Nutz, J. C. Namyslo, A. E. W. Adam, and D. E. Kaufmann, Synthesis, 2006, 2927. CrossRef
6. V. A. Zapol’skii, J. C. Namyslo, M. Gjikaj, and D. E. Kaufmann, Synlett, 2007, 1507. CrossRef
7. (a) V. A. Zapol’skii, J. C. Namyslo, A. E. W. Adam, and D. E. Kaufmann, Heterocycles, 2004, 63, 1281; CrossRef (b) E.-J. Vogt, V. A. Zapol’skii, E. Nutz, and D. E. Kaufmann, Z. Naturforsch., 2012, 67b, 285; (c) S. Lethu and J. Dubois, Eur. J. Org. Chem., 2011, 20-21, 3920; CrossRef (d) D. Thomae, J. C. R. Dominguez, G. Kirsch, and P. Seck, Tetrahedron, 2008, 64, 3232; CrossRef (e) H. S. P. Rao and K. Vasantham, J. Org. Chem., 2009, 74, 6847; CrossRef (f) L. Wen and S. C. Rasmussen, J. Chem. Crystallogr., 2007, 37, 387; CrossRef (g) A. R. Katritzky, A. V. Vakulenko, J. Sivapackiam, B. Draghici, and R. Damavarapu, Synthesis, 2008, 699. CrossRef
8. (a) L. Bianchi, C. Dell’Erba, M. Maccagno, S. Morganti, M. Novi, G. Petrillo, E. Rizzato, F. Sancassan, E. Severi, D. Spinelli, and C. Tavani, Tetrahedron, 2004, 60, 4967; CrossRef (b) C. Dell’Erba, A. Gabellini, A. Mugnoli, M. Novi, G. Petrillo, and C. Tavani, Tetrahedron, 2001, 57, 9025; CrossRef (c) C. Dell’Erba, A. Gabellini, M. Novi, G. Petrillo, C. Tavani, B. Cosimelli, and D. Spinelli, Tetrahedron, 2001, 57, 8159. CrossRef
9. (a) J. O. Morley and T. P. Matthews, Bioorg. Med. Chem., 2006, 14, 8099; CrossRef (b) J. O. Morley and T. P. Matthews, Org. Biomol. Chem., 2006, 4, 359; CrossRef (c) D. Briel, A. Rybak, C. Kronbach, and K. Unverferth, Eur. J. Med. Chem., 2010, 45, 69; CrossRef (d) P. Mishra, H. K. Maurya, B. Kumar, V. K. Tandon, and V. J. Ram, Tetrahedron Lett., 2012, 53, 1056; CrossRef (e) A. Fontana, M. Viale, S. Guernelli, C. Casbarri, E. Rizzato, M. Maccagno, G. Petrillo, C. Aiello, S. Ferrini, and D. Spinelli, Org. Biomol. Chem., 2010, 8, 5674. CrossRef
10. (a) C. Ibis, A. F. Tuyun, and G. Aydinli, Asian J. Chem., 2010, 22, 1360; (b) C. Ibis, A. F. Tuyun, and G. Aydinli, Phosphorus, Sulfur Silicon Relat. Elem., 2010, 185, 2014. CrossRef
11. (a) C. Ibis and A. F. Tuyun, Curr. Org. Synth., 2011, 8, 861; (b) C. Ibis and A. F. Tuyun, Phosphorus, Sulfur Silicon Relat. Elem., 2012, 187, 1161. CrossRef
12. N. I. Nechai, V. I. Potkin, P. V. Kurman, and R. V. Kaberdin, Dokl. Akad. Nauk. Belarusi, 1998, 42, 75.
13. (a) V. I. Potkin, N. I. Nechai, and R. V. Kaberdin, Russ. J. Org. Chem., 1996, 32, 657; (b) R. V. Kaberdin, V. I. Potkin, and V. A. Zapol’skii, Russ. Chem. Rev., 1997, 66, 827. CrossRef