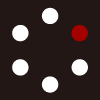
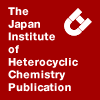
HETEROCYCLES
An International Journal for Reviews and Communications in Heterocyclic ChemistryWeb Edition ISSN: 1881-0942
Published online by The Japan Institute of Heterocyclic Chemistry
e-Journal
Full Text HTML
Received, 10th October, 2013, Accepted, 26th November, 2013, Published online, 12th December, 2013.
DOI: 10.3987/COM-13-12861
■ Ferrier Glycosylation Reaction Catalyzed by Bi(OTf)3-Montmorillonite K-10: Efficient Synthesis of 3,4-Unsaturated Sialic Acid Derivatives: Synthesis and Biological Evaluation as Inhibitors of Human Parainfluenza Virus Type 1
Mai Oba, Yayoi Ueno, Satoru Kitani, Takuya Hayakawa, Tadanobu Takahashi, Takashi Suzuki, Masayuki Sato, and Kiyoshi Ikeda*
Department of Pharmaceutical Sciences, Hiroshima International University, 5-1-1, Hirokoshingai, Kure, Hiroshima, 737-0112, Japan
Abstract
The reaction of the 4,5-oxazoline derivative of sialic acid with various alcohols was effectively promoted by a catalytic amount of montmorillonite K-10 clay-supported Bi(OTf)3 to produce a variety of 3,4-unsaturated sialic acids via the Ferrier glycosylation reaction in moderate yields. The deprotection of the isopropylidene group and hydrolysis of the ester group of 7a-g gave 4a-f, whose inhibitory activities against hPIV-1 sialidase were studied.INTRODUCTION
N-Acetylneuraminic acid (Neu5Ac, 1) and its various analogs are critical components of cell surface glycoconjugates involved in cellular recognition processes.1 A variety of compounds have been reported to inhibit the action of influenza virus sialidase and, of these, the most interesting and the most extensively studied are 2-deoxy-2,3-didehydro-N-acetylneuraminic acid (Neu5Ac2en, 2) and the corresponding analogs.2 Among them, 2,3-didehydro-2,4-dideoxy-4-guanidinyl-N-acetylneuraminic acid (Zanamivir, 3)3 in Figure 1 has been approved for human use as a specific sialidase inhibitor for anti-influenza drugs.
Human parainfluenza virus type 1 (hPIV-1) is a serious pathogen causing upper and lower respiratory disease in infants and young children;4 however, there are no known effective inhibitors of hPIV-1 infection. Lewis acid-catalyzed allylic rearrangement of acyloxy glycals is a well-known Ferrier glycosylation reaction5 and is widely employed to obtain 2,3-unsaturated glycosides, which are versatile chiral intermediates in the synthesis of several biologically active natural products.6 Owing to its great significance in the area of carbohydrate chemistry, there has been growing interest in the development of the Ferrier glycosylation reaction by using a variety of catalysts. Despite their usefulness, strong Lewis acid catalysts, such as BF3·OEt27 and SnCl4,8 are generally employed to obtain 2,3-unsaturated glycosides. Other reagents, such as the clay catalyst montmorillonite K-10,9 bismuth (III) trifluoromethanesulfonate (bismuth triflate) [Bi(OTf)3] ,10 Bi(OTf)3-SiO2,10 DDQ,11 N-iodosuccinimide,12 AuCl3,13 and Fe(OTf)314 and lanthanide triflates such as Yb(OTf)315 and Sc(OTf)316 are known to bring about the Ferrier glycosylation reaction under mild conditions. Bismuth triflate was also found to be a mild and effective promoter of glycosylation.17 Recently, glycal derivatives as glycosyl donors have been utilized in p-allylpalladium strategies for the stereoselective synthesis of O-glycosides.18
In order to design new hPIV-1 sialidase inhibitors, 3,4-unsaturated sialic acid derivatives are promising candidates as transition-state analogs for the enzyme reaction.19 Compound 4a (XR = OMe in Figure 1) was found to be a weak inhibitor against sialidase from influenza virus.20 In keeping with our interest in the development of new inhibitors against hPIV-1 sialidase,21-23 we reported our preliminary results24 on the interesting use of Bi(OTf)3-montmorillonite K-10 as an efficient catalyst for the synthesis of 3,4-unsaturated sialic acid derivative 4 via the Ferrier glycosylation reaction starting from sialic acid 4,5-oxazoline derivative 5. To the best of our knowledge, this is the first report on Bi(OTf)3-montmorillonite K-10-mediated Ferrier glycosylation reaction of sialic acid derivatives (Figure 2). We describe herein the synthesis of 3,4-unsaturated sialic acid derivatives promoted by Bi(OTf)3-montmorillonite K-10 and their evaluation of their inhibitory activities against hPIV-1 sialidase.
RESULTS AND DISCUSSION
Neu5Ac 1 was transformed with inversion of the configuration at C-4 into the oxazoline derivative 525 by the treatment with trimethylsilyl trifluoromethanesulfonate (TMSOTf) in 76% yield in three steps (Chart 1).
We first investigated the Ferrier glycosylation reaction to construct methyl ketoside of 3,4-unsaturated sialic acid derivatives 7a. Thus, different reaction conditions, including promoters and solvents, were examined. These results are summarized in Table 1. The reaction between 5 and MeOH as a glycosyl acceptor using IR120 (H+) as Brønsted acid at room temperature gave the 3,4-unsaturated glycoside 7a in 31% yield as an anomeric mixture with the β-anomer as the major product (α/β = 6:94) (Table 1; entry 1). The α/β ratio of 7a26 was determined by 1H-NMR analysis. Using Lewis acids, TMSOTf, Hf(OTf)4, Zn(OTf)2, Yb(OTf)3, Sc(OTf)3, and InCl3 as promoters in MeCN, the reaction gave 7a in 56%, 42%, 45%, 45%, 51%, and 69% yield, respectively, as almost a β-anomer (Table 1; entries 2-7). A relatively large amount of α-glycosides 7a was obtained in MeCN in the presence of 0.8 equivalent of BF3·OEt2 in 51% yield with an α/β ratio of 41:59, as expected from the more significant solvent participation of MeCN than of CH2Cl2 (entries 8 and 9). The solvent effect of CPME27 was examined. When the reaction of 5 with MeOH was carried out using 0.8 equivalent of BF3·OEt2 in CPME at room temperature, however, no increment of α-selectivity was observed (α/β ratio of 17:83, entry 10). When both the 0.2 equivalent of Bi(OTf)3 and 30% w/w montmorillonite K-10 were used as activators, the reactions gave 7a in 50% and 63% yield, respectively (entries 11 and 12). Interestingly, the reaction of 5 with MeOH in the presence of 40% w/w Bi(OTf)3-montmorillonite K-10 loading of 20% w/w Bi(OTf)3 in MeCN showed remarkable improvement in the glycosylation yield (95%, α/β ratio of 7:93) (entry 13). This synergistic enhancement by the addition of montmorillonite K-10 to Bi(OTf)3 was tentatively understood by the formation of a montmorillonite K-10-ROH complex, which might activate 5 under the influence of Bi(OTf)3. This result shows that it is important to use the combination of Bi(OTf)3 and montmorillonite K-10 for higher yields in the Ferrier glycosylation reaction. The solvent effects were tested; however, the yields of 7a in CH2Cl2 and Et2O decreased to 73% and 47% yield, respectively (entries 14 and 15).
Next, the glycosyl donor of the propriety of 5 was evaluated by coupling with various alcohols. As summarized in Table 2, the reactions of 5 with ethyl, n-propyl, i-propyl, n-butyl, benzyl alcohols and 1-dodecanethiol were activated by 40% w/w Bi(OTf)3 (20%) -montmorillonite K-10 in MeCN at room temperature to give 7b-g in 77%, 44%, 32%, 50%, 41%, and 40% yield, respectively, as an anomeric mixture with the β-anomer as the major product.
The possible mechanism of the Ferrier glycosylation reaction involves the intermediacy of a cyclic allylic oxonium ion X with which the nucleophile undergoes α subsequent addition reaction (Chart 2). Molecular orbital calculations using the Spartan’04 Semi-Empirical Program PM3 of the cyclic allylic oxonium ion X in Chart 2 were carried out to study the origin of β orientation.
The stereostructure of the lowest energy conformation of X is shown in Figure 3. The pyran ring has an almost planar conformation. This conformation led us to suppose that nucleophilic attack would occur from the β face of the donor moiety X to result in the predominant formation of β-glycoside, since the α face is hindered by the acetyl group at C-7 (Figure 3).
De-O-acetylation of 4a and subsequent saponification of the resulting methyl ester gave sialidase inhibitor 7a in 92% yield in two steps (Table 3).
BIOLOGICAL EVALUATIONS
The behavior of compounds 4a-f toward hPIV-1 sialidase was tested by a fluorometric assay using 4-methylumbelliferyl N-acetyl-α-neuraminic acid as per our previously reported method.22 As can be seen in Table 3, among synthesized compounds 4a-f, compounds 4d and 4f showed the most inhibitory activity against hPIV-1 sialidase (IC50 = 2.9 mM and 3.0 mM, respectively) (Table 3, entries 4 and 6). However, the degree of inhibition of 4f was much weaker than that of 1 (IC50 = 0.2 mM). It is suggested that the expression of inhibitory activity of sialic acid derivatives against hPIV-1 requires the structural feature of a 2,3-double bond of sialic acid.
The present findings should provide useful information for the development of anti-human parainfluenza virus compounds.
CONCLUSIONS
Our synthesis approach is the first example of the Ferrier glycosylation reaction for the construction of 3,4-unsaturated sialic acid derivatives. It is important to use the combination of Bi(OTf)3 and montmorillonite K-10 for higher yields in the Ferrier glycosylation reaction. We believe that this synthesis method provides a practical route to establish novel 3,4-unsaturated sialic acid analogs. The use of inexpensive and readily accessible Bi(OTf)3 with high yields makes it a useful and attractive alternative to the more expensive lanthanide triflate or stoichiometric conventional Lewis acid-promoted O-glycosidation procedures. We are currently applying this methodology to the development of new hPIV-1 inhibitors.
EXPERIMENTAL
All melting points are uncorrected. Optical rotations were measured with a JASCO P-1030s (Japan) digital polarimeter. FT-IR spectra were recorded on a SHIMADZU IRPrestige-21 (Japan) spectrometer. 1H NMR spectra were recorded with a JEOL ECA-500 (500 MHz) (Japan) instrument. 13C-NMR spectra were recorded with a JEOL ECA-500 (126 MHz) (Japan) instrument. Chemical shifts are expressed in ppm relative to Me4Si (δ = 0) in CDCl3 and in D2O referenced to HOD (4.85 ppm) as internal standards. MS and high-resolution (HR)-FAB-MS data were obtained using a JEOL JMS-700 (Japan) spectrometer in the positive mode with an NBA matrix. MS and high-resolution ESI (HR-ESI) were measured on a JEOL JMS-T100LC (Japan) mass spectrometer. Column chromatography was performed on Silica Gel 60 (70-230 mesh, Merck). All reactions were monitored using TLC (Silica Gel 60F254, E. Merck, Germany) by charring after spraying 5% H2SO4 in MeOH and then heating.
5-Acetamido-2-methoxy-6-(1,2,3-triacetoxypropyl)-5,6-dihydro-2H-pyran-2-carboxylic acid methyl ester (7a): The oxazoline derivative 5 (50 mg, 0.12 mmol) was dissolved in a mixture of 1.0 mL of MeCN and 0.1 mL of MeOH under argon, and Bi(OTf)3-montmorillonite K-10 (20 mg, 30% w/w) was added. The reaction mixture was allowed to stand at room temperature for 20 h. The reaction mixture was filtered over Celite, and several drops of NEt3 were added. The solvent was then evaporated and the residue obtained purified by chromatography over silica gel with CH2Cl2/MeOH (10:1). Yield of 7a: (51 mg) (95%); α-7a: 1H-NMR (CDCl3) δ: 1.99 (3H, s), 2.05, 2.12, 2.14 (each 3H, s), 3.34 (3H, s), 3.78 (3H, s), 4.24 (1H, dd, J = 12.4, 5.9 Hz), 4.26 (1H, ddd, J = 9.2, 9.8, 2.1 Hz), 4.48 (1H, dd, J = 2.4, 12.4 Hz), 4.52 (1H, m), 5.35 (1H, dd, J = 6.1, 2.1 Hz), 5.44 (1H, m), 5.59 (1H, d, J = 5.9 Hz), 5.78 (1H, dd, J = 10.1, 2.6 Hz), 6.07 (1H, dd, J = 1.9, 10.1 Hz). β-7a: 1H-NMR (CDCl3) δ: 1.99 (3H, s), 2.04, 2.10, 2.16 (each 3H, s), 3.29 (3H, s), 3.82 (3H, s), 4.05 (1H, dd, J = 10.2, 2.3 Hz), 4.24 (1H, dd, J = 12.5, 6.3 Hz) 4.63 (1H, dd, J = 2.3, 12.5 Hz), 4.64 (1H, d, J = 10.2 Hz), 5.35 (1H, m), 5.40 (1H, dd, J = 5.6, 2.3 Hz), 5.51 (1H, d, J = 9.6 Hz), 5.91 (2H, m). Positive ion MS (ESI) m/z: 468 (M+Na)+. HR-MS (ESI) Calcd for C19H27O11NNa (M+Na)+: 468.14818; Found: 468.14515.
5-Acetamido-2-ethoxy-6-(1,2,3-triacetoxypropyl)-5,6-dihydro-2H-pyran-2-carboxylic acid methyl ester (7b): The reaction was carried out using 5 (50 mg, 0.12 mmol) and Bi(OTf)3-montmorillonite K-10 (20 mg, 30% w/w) in a mixture of 1.0 mL of MeCN and 0.1 mL of EtOH in a manner similar to the preparation of 7a, to give 7b (20 mg, 77%). β-7b: 1H-NMR (CDCl3) δ: 1.21 (3H, t, J = 7.1 Hz), 1.98 (3H, s), 2.04, 2.10, 2.16 (each 3H, s), 3.41 (1H, m), 3.64 (1H, m), 3.81 (3H, s), 4.07 (1H, dd, J = 10.3, 2.9 Hz), 4.24 (1H, dd, J = 12.3, 6.3 Hz), 4.65 (1H, dd, J = 2.7, 12.3 Hz), 4.68 (1H, m), 5.29 (1H, m), 5.39 (1H, dd, J = 2.9, 4,5 Hz), 5.51 (1H, d, J = 9.7 Hz), 5.89 (1H, d, J = 10.3 Hz), 5.94 (1H, dd, J = 2.0, 10.3 Hz). 13C-NMR (CDCl3) δ:15.3, 20.7, 20.8, 21.1, 23.3, 43.0, 52.8, 60.4, 62.3, 68.3, 70.6, 71.3, 96.1, 126.2, 133.3, 167.8, 169.8, 170.1, 170.5, 170.6. Positive ion MS (ESI) m/z: 482 (M+Na)+. HR-MS (ESI) Calcd for C20H29O11NNa (M+Na)+: 482.16383; Found: 482.16209.
5-Acetamido-2-propoxy-6-(1,2,3-triacetoxypropyl)-5,6-dihydro-2H-pyran-2-carboxylic acid methyl ester (7c): The reaction was carried out using 5 (50 mg, 0.12 mmol) and Bi(OTf)3-montmorillonite K-10 (20 mg, 30% w/w) in a mixture of 1.0 mL of MeCN and 0.1 mL of n-PrOH in a manner similar to the preparation of 7a, to give 7c (43 mg, 77%). β-7c: 1H-NMR (CDCl3) δ: 0.91 (3H, t, J = 7.5 Hz), 1.57 (2H, m), 1.96 (3H, s), 2.02, 2.08, 2.14 (each 3H, s), 3.27 (1H, m), 3.52 (1H, m), 3.79 (3H, s), 4.06 (1H, dd, J = 10.3, 2.3 Hz), 4.24 (1H, dd, J = 12.6, 7.4 Hz), 4.65 (1H, m), 4.69 (1H, dd, J = 2.1, 12.6 Hz), 5.25 (1H, m), 5.38 (1H, dd, J = 2.3, 5.8 Hz), 5.45 (1H, d, J = 9.7 Hz), 5.86 (1H, dd, J = 10.0, 2.6 Hz), 5.94 (1H, d, J = 10.0 Hz). 13C-NMR (CDCl3) δ: 10.4, 20.7, 20.8, 21.0, 23.0, 23.3, 42.9, 52.8, 62.4, 66.3, 68.5,70.8, 71.8, 96.0, 126.2, 133.2, 167.7, 169.9, 170.2, 170.5, 170.6.Positive ion HR-FABMS (NBA) (m/z) Calcd for C21H32NO11 [M+H]+: 474.1975; Found 474.1960.
5-Acetamido-2-isopropoxy-6-(1,2,3-triacetoxypropyl)-5,6-dihydro-2H-pyran-2-carboxylic acid methyl ester (7d): The reaction was carried out using 5 (50 mg, 0.12 mmol) and Bi(OTf)3-montmorillonite K-10 (20 mg, 30% w/w) in a mixture of 1.0 mL of MeCN and 0.1 mL of i-PrOH in a manner similar to the preparation of 7a, to give 7d (19 mg, 32%). β-7d: 1H-NMR (CDCl3) δ: 1.10 (3H, d, J = 5.7 Hz), 1.23 (3H, d, J = 6.3 Hz), 1.97 (3H, s), 2.04, 2.05, 2.09 (each 3H, s), 3.80 (3H, s), 4.11-4.16 (2H, m), 4.27 (1H, dd, J = 12.6, 8.0 Hz), 4.72 (1H, m), 4.83 (1H, dd, J = 12.6, 2.0 Hz), 5.28 (1H, m), 5.41 (1H, dd, J = 3.0, 5.0 Hz), 5.45 (1H, d, J = 9.7 Hz), 5.84 (1H, d, J = 10.0 Hz), 6.04 (1H, dd, J = 2.3, 10.0 Hz). 13C-NMR (CDCl3) δ: 20.7, 21.1, 22.7, 23.3, 24.6, 42.9, 52.7, 62.7, 67.8, 69.4, 71.2, 72.5, 94.5, 126.7, 132.3, 168.0, 169.8, 170.1, 170.6, 170.9. Positive ion MS (ESI) m/z: 496 (M+Na)+. HR-MS (ESI) Calcd for C21H31O11NNa (M+Na)+: 496.17948; Found: 496.18086.
5-Acetamido-2-butoxy-6-(1,2,3-triacetoxypropyl)-5,6-dihydro-2H-pyran-2-carboxylic acid methyl ester (7e): The reaction was carried out using 5 (50 mg, 0.12 mmol) and Bi(OTf)3-montmorillonite K-10 (20 mg, 30% w/w) in a mixture of 1.0 mL of MeCN and 0.1 mL of n-BuOH in a manner similar to the preparation of 7a, to give 7e (30 mg, 50%). β-7e: 1H-NMR (CDCl3) δ: 0.91 (3H, t, J = 7.4 Hz), 1.36 (2H, dt, J = 15.5, 7.4 Hz), 1.54 (2H, m), 1.97 (3H, s), 2.03, 2.09, 2.15 (each 3H, s), 3.31 (1H, m), 3.56 (2H, m), 3.80 (3H, s), 4.07 (1H, dd, J = 10.3, 2.3 Hz), 4.25 (1H, dd, J = 12.3, 7.5 Hz), 4.67 (1H, m), 4.69 (1H, dd, J =2.5, 12.3 Hz), 5.26 (1H, m), 5.39 (1H, dd, J = 2.3, 2.3 Hz), 5.49 (1H, d, J = 9.7 Hz), 5.87 (1H, d, J = 10.0 Hz), 5.94 (1H, dd, J = 2.3, 10.0 Hz). 13C-NMR (CDCl3) δ: 13.8, 19.2, 20.71, 20.74, 21.0, 23.3, 31.8, 42.9, 52.7, 62.4, 64.6, 68.5, 70.8, 96.0, 126.2, 133.2, 167.7, 169.8, 170.1, 170.5, 170.6. Positive ion HR-FABMS (NBA) (m/z) Calcd for C22H34NO11 [M+H]+ : 488.2132, Found 488.2147.
5-Acetamido-2-benzyloxy-6-(1,2,3-triacetoxypropyl)-5,6-dihydro-2H-pyran-2-carboxylic acid methylester (7f): The reaction was carried out using 5 (50 mg, 0.12 mmol) and Bi(OTf)3-montmorillonite K-10 (20 mg, 30% w/w) in a mixture of 1.0 mL of MeCN and 0.1 mL of benzyl alcohol in a manner similar to the preparation of 7a, to give 7f (26 mg, 41%). β-7f: 1H-NMR (CDCl3) δ: 1.95 (3H, s), 1.97, 2.04, 2.17 (each 3H, s), 3.76 (3H, s), 4.15 (1H, dd, J = 10.3, 2.3 Hz), 4.25 (1H, dd, J = 12.8, 6.5 Hz), 4.48 (1H, d, J = 12.0 Hz), 4.64 (1H, d, J = 12.0 Hz), 4.67 (1H, m), 4.70 (1H, dd, J = 2.0, 12.8 Hz), 5.32 (1H, m), 5.41 (1H, dd, J = 5.3, 2.3 Hz), 5.71 (1H, br d), 5.91 (1H, d, J = 10.0 Hz), 6.02 (1H, dd, J = 2.6, 10.0 Hz), 7.35 (5H, m). 13C-NMR (CDCl3) δ: 20.7, 20.8, 23.2, 30.3, 38.6, 43.0, 50.8, 52.8, 62.3, 66.5, 68.3, 70.7, 71.4, 96.0, 125.8, 127.5, 127.8, 128.4, 133.4, 136.9, 167.6, 170.0, 170.1, 170.6, 170.7. Positive ion MS (ESI) m/z: 544 (M+Na)+. HR-MS (ESI) Calcd for C25H31O11NNa (M + Na)+: 544.17948; Found: 544.18228.
5-Acetamido-2-dodecylsulfanyl-6-(1,2,3-triacetoxypropyl)-5,6-dihydro-2H-pyran-2-carboxylic acid methyl ester (7g): The reaction was carried out using 5 (50 mg, 0.12 mmol), 1-dodecanethiol (98 mg, 0.48 mmol) and BF3·OEt2 (34 mg, 0.24 mmol) in 1.0 mL of CH2Cl2 in a manner similar to the preparation of 7a, to give 7g (30 mg, 40%). α-7g: 1H-NMR (CDCl3) δ: 0.88 (3H, t, J = 6.9 Hz), 1.30 (18H, m), 1.48 (2H, q, J = 7.3 Hz), 1.98 (3H, s), 2.05, 2.10, 2.15 (each 3H, s), 2.57 (2H, m), 3.82 (3H, s), 4.28 (1H, dd, J = 12.3, 7.2 Hz), 4.36 (1H, dd, J = 10.0, 2.4 Hz), 4.64 (1H, m), 4.68 (1H, dd, J = 2.3, 12.3 Hz), 5.21 (1H, ddd, J = 4.4, 7.2, 2.3 Hz), 5.40 (1H, dd, J = 2.4, 4.4 Hz), 5.48 (1H, br d), 5.75 (1H, d, J = 10.3, Hz), 6.13 (1H, dd, J = 2.6, 10.3 Hz). 13C-NMR (CDCl3) δ: 14.1, 20.7, 21.0, 22.6, 23.3, 24.6, 28.3, 29.0, 29.3, 29.4, 29.5, 29.6, 31.8, 34.0, 42.8, 52.8, 62.5, 68.5, 70.7, 85.4, 126.4, 130.5, 167.3, 169.7, 170.2, 170.5. Positive ion MS (ESI) m/z: 638 (M+Na)+. HR-MS (ESI) Calcd for C30H49O10NSNa (M+Na)+: 638.29749; Found: 638.29845.
5-Acetamido-2-methoxy-6-(1,2,3-trihydroxypropyl)-5,6-dihydro-2H-pyran-2-carboxylic acid (4a): To a solution of compound 7a (65 mg, 0.15 mmol) in dry MeOH (1.0 mL) was added NaOMe (2 drops, 25% w/w MeOH solution) and the mixture was stirred for 1 h at 0 °C under Ar, and then Amberlite IRC-50 (0.5 g) resin was added to remove sodium ions, after which the sample was filtered and concentrated to dryness. The residue was dissolved in MeOH (1.0 mL) and to the mixture was added 5 drops of 1.0 M NaOH aqueous solution at room temperature: the mixture was then stirred overnight, made neutral with Amberlite IR-120 (H+ form) (0.2 g) resin, filtered, and the filtrate was concentrated to dryness. The residue was purified by silica gel column chromatography using CHCl3-MeOH-H2O (6:6:1) to give 4a (27 mg, 60%). FT-IR (neat) 1734, 1616, 1373, 1080 cm-1; 1H-NMR (D2O) δ: 1.84 (3H, s), 3.12 (3H, s), 3.47-3.51 (2H, m), 3.64 (1H, dd, J = 2.9, 12.0 Hz), 3.74-3.78 (1H, m), 3.83 (1H, d, J = 10.4 Hz), 4.58 (1H, d, J = 10.4 Hz), 5.77 (2H, s). MS (ESI) m/z: 328 (M+Na)+. HR-MS (ESI) Calcd for C12H19O8NNa (M+Na)+: 328.10084; Found: 328.09663.
5-Acetamido-2-ethoxy-6-(1,2,3-trihydroxypropyl)-5,6-dihydro-2H-pyran-2-carboxylic acid (4b): The reaction was carried out using 7b (13 mg, 0.03 mmol) in a manner similar to the preparation of 4a, to give 4b (5 mg, 52%), FT-IR (neat) 1734, 1363, 1217, 648 cm-1; 1H-NMR (D2O) δ: 1.01 (3H, t, J = 6.9 Hz), 1.85 (3H, s), 3.22 (1H, q, J = 9.2 Hz), 3.45-3.56 (3H, m), 3.64 (1H, dd, J = 2.9, 12.1 Hz), 3.71-3.72 (1H, m), 3.85 (1H, d, J = 10.3 Hz), 4.56 (1H, d, J = 10.3 Hz), 5.77 (2H, s). MS (ESI) m/z: 342 (M+Na)+. HR-MS (ESI) Calcd for C13H21O8NNa (M+Na)+: 342.11649; Found: 342.11428.
5-Acetamido-2-propoxy-6-(1,2,3-trihydroxypropyl)-5,6-dihydro-2H-pyran-2-carboxylic acid (4c): The reaction was carried out using 7c (19 mg, 0.04 mmol) in a manner similar to the preparation of 4a, to give 4c (5 mg, 38%). FT-IR (neat) 1734, 1364, 1217, 632 cm-1; 1H-NMR (D2O) δ: 0.71 (3H, t, J = 7.5 Hz), 1.39 (2H, q, J = 6.9 Hz), 1.83 (3H, s), 3.10-3.15 (1H, m), 3.41-3.51 (3H, m), 3.62-3.67 (1H, m), 3.68-3.76 (1H, m), 3.80 (1H, t, J = 10.4 Hz), 4.54 (1H, d, J = 10.4 Hz), 5.73-5.77 (2H, m). MS (ESI) m/z: 356 (M+Na)+. HR-MS (ESI) Calcd for C14H23O8NNa (M+Na)+: 356.13214; Found: 356.13227.
5-Acetamido-2-isopropoxy-6-(1,2,3-trihydroxypropyl)-5,6-dihydro-2H-pyran-2-carboxylic acid (4d): The reaction was carried out using 7d (43 mg, 0.09 mmol) in a manner similar to the preparation of 4a, to give 4d (10 mg, 32%). FT-IR (neat) 2922, 1737, 1624, 1259, 1072 cm-1; 1H-NMR (D2O) δ: 0.98-1.05 (6H, m), 1.85 (3H, s), 3.17 (1H, t, J = 8.0 Hz), 3.43-3.50 (2H, m), 3.65-3.93 (3H, m), 5.78 (1H, dd, J = 2.3, 9.8 Hz), 5.86 (1H, dt, J = 2.0, 12.4 Hz). MS (ESI) m/z: 356 (M+Na)+. HR-MS (ESI) Calcd for C14H23O8NNa (M+Na)+: 356.13214; Found: 356.13399.
5-Acetamido-2-butoxy-6-(1,2,3-trihydroxypropyl)-5,6-dihydro-2H-pyran-2-carboxylic acid (4e): The reaction was carried out using 7e (28 mg, 0.06 mmol) in a manner similar to the preparation of 4a, to give 4e (14 mg, 65%). FT-IR (neat) 3304, 2924, 1730, 1627, 1541, 1074 cm-1; 1H-NMR (D2O) δ: 0.66-0.70 (3H, m), 1.12-1.32 (2H, m), 1.28-1.38 (2H, m), 1.82 (3H, s), 3.16-3.20 (1H, m), 3.37-3.43 (2H, m), 3.48-3.56 (1H, m), 3.61-3.82 (2H, m), 3.89 (1H, dd, J = 1.1, 10.4 Hz), 4.53 (1H, t, J = 9.8 Hz), 5.75-5.80 (2H, m). MS (ESI) m/z: 370 (M+Na)+. HR-MS (ESI) Calcd for C15H25O8NNa (M+Na)+: 370.14779; Found: 370.14971.
5-Acetamido-2-benzyloxy-6-(1,2,3-trihydroxypropyl)-5,6-dihydro-2H-pyran-2-carboxylic acid (4f): The reaction was carried out using 7f (43 mg, 0.08 mmol) in a manner similar to the preparation of 4a, to give 4f (9 mg, 28%). FT-IR (neat) 3317, 2924, 1734, 1627, 1541, 1074, 1035 cm-1; 1H-NMR (D2O) δ: 1.83 (3H, s), 3.42-3.49 (2H, m), 3.65-3.69 (1H, m), 3.81 (1H, dd, J = 1.7, 9.8 Hz), 3.89 (1H, d, J = 10.0 Hz), 4.42 (2H, s), 4.53-4.56 (1H, m), 5.78-5.85 (1H, m), 5.93 (1H, dd, J = 1.7, 8.0 Hz), 7.17-7.25 (5H, m). MS (ESI) m/z: 404 (M+Na)+. HR-MS (ESI) Calcd for C18H23O8NNa (M+Na)+: 404.13214; Found: 404.13361.
ACKNOWLEDGEMENTS
This work was financially supported in part by a Grant-in-Aid for Scientific Research No. 24590155 from the Ministry of Education, Science, Sports and Culture of Japan.
References
1. ‘Biology of the Sialic Acids,’ ed. by A. Rosenberg, Plenum Press: New York, 1995. CrossRef
2. K. Ikeda, M. Sato, and Y. Torisawa, Curr. Med. Chem., 2004, 3, 339.
3. M. von Itzstein, W.–Y. Wu, G. B. Kok, M. S. Pegg, J. C. Dyason, B. Jin, T. V. Phan, M. L. Smythe, H. F. White, S. W. Oliver, P. M. Colman, J. N. Varghese, D. M. Ryan, J. M. Woods, R. C. Bethell, V. J. Hotham, J. M. Cameron, and C. R. Penn, Nature, 1993, 363, 418. CrossRef
4. B. R. Murphy, Parainfluenza viruses, ‘In Infectious Diseases,’ ed. by S. L. Gorbach, J. G. Bartlett, and N. R. Blacklow, W. B. Saunders Company, Philadelphia, 1998, pp. 2125-2131.
5. R. J. Ferrier and N. J. Prasad, J. Chem. Soc. C, 1969, 570. CrossRef
6. R. J. Ferrier, Adv. Carbohydr. Chem. Biochem., 1969, 24, 199. CrossRef
7. E. Wieczorek and J. Thiem, Carbohydr. Res., 1998, 307, 263. CrossRef
8. G. Grynkiewicz, W. Priebe, and A. Zamojski, Carbohydr. Res., 1979, 68, 33. CrossRef
9. K. Toshima, T. Ishizuka, G. Matsuo, and M. Nakata, Synlett, 1995, 306. CrossRef
10. J. L. Babu, A. Khare, and Y. D. Vankar, Molecules, 2005, 10, 884. CrossRef
11. K. Toshima, T. Ishizuka, G. Matsuo, and M. Nakata, Chem. Lett., 1993, 22, 2013. CrossRef
12. J. C. Lopez, A. M. Gomez, S. Valverdi, and B. Fraser-Reid, J. Org. Chem., 1995, 60, 3851. CrossRef
13. B. S. Rengarajan and R. K. Srinvivasa, Tetrahedron, 2009, 65, 8139. CrossRef
14. C. Periran and W. Shaoshan, Tetrahedron, 2012, 68, 5336.
15. M. Takhi, A. A.-H. Abdel-Rahman, and R. R. Schmidt, Synlett, 2001, 427. CrossRef
16. J. S. Yadav, B. V. S. Reddy, C. V. S. R. Murthy, and K. G. Mahesh, Synlett, 2000, 1450. CrossRef
17. K. Ikeda, Y. Torisawa, T. Nishi, J.-I. Minamikawa, K. Tanaka, and M. Sato, Bioorg. Med. Chem., 2003, 11, 3073. CrossRef
18. T. Yamanoi, R. Inoue, S. Matsuda, K. Katsuraya, and K. Hamasaki, Tetrahedron: Asymmetry, 2006, 17, 2914. CrossRef
19. B. P. Schuff, G. J. Mercer, and H. M. Nguyen, Org. Lett., 2007, 9, 3173. CrossRef
20. J. Maudrin, B. Barrere, B. Chantegrel, C. Deshayes, G. Quash, and A. Doutheau, Bull. Soc. Chim. Fr., 1994, 131, 400.
21. K. Ikeda, K. Sano, M. Ito, M. Saito, K. Hidari, T. Suzuki, Y. Suzuki, and K. Tanaka, Carbohydr. Res., 2001, 330, 31. CrossRef
22. T. Suzuki, K. Ikeda, N. Koyama, C. Hosokawa, T. Kogure, T. Takahashi, K. Hidari, D. Miyamoto, K. Tanaka, and Y. Suzuki, Glycoconjugate J., 2001, 18, 331. CrossRef
23. K. Sato, K. Ikeda, T. Suzuki, S. Aoyama, N. Maki, Y. Suzuki, and M. Sato, Tetrahedron, 2007, 63, 7571. CrossRef
24. K. Ikeda, Y. Ueno, S. Kitani, R. Nishino, and M. Sato, Synlett, 2008, 1027. CrossRef
25. M. Chandler, M. J. Bamford, R. Conroy, B. Lamont, B. Patel, V. K. Patel, I. P. Steeples, R. Storer, N. G. Weir, M. Wright, and C. Williamson, J. Chem. Soc., Perkin Trans. 1, 1995, 1173. CrossRef
26. The α-methyl ketoside of 5-acetamido-3,4,5-trideoxy-D-manno-non-3-en-2-ulosonic acid 4a, and its β-epimer were synthesized from 3-acetamido-4,5,6,7-tetra-O-acetyl-2,3-dideoxy-D-manno-heptose and methyl 2-methoxy-2-dimethylphosphono acetate in 29% yield over five steps.20.
27. H. Tokimoto, Y. Fujimoto, K. Fukase, and S. Kusumoto, Tetrahedron: Asymmetry, 2005, 16, 441. CrossRef