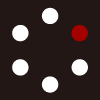
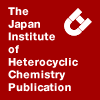
HETEROCYCLES
An International Journal for Reviews and Communications in Heterocyclic ChemistryWeb Edition ISSN: 1881-0942
Published online by The Japan Institute of Heterocyclic Chemistry
e-Journal
Full Text HTML
Received, 14th October, 2013, Accepted, 31st October, 2013, Published online, 14th November, 2013.
DOI: 10.3987/COM-13-12863
■ Radical Cyclization and 1,5-Hydrogen Transfer in Selected Aromatic Diazonium Salts
Benedetta Maggio, Gianfranco Fontana, Demetrio Raffa, Francesco Ferrante, and Giuseppe Daidone*
STEBICEF, University of Palermo, Via Archirafi 32, 90123 Palermo, Italy
Abstract
2-(Methyl-(3-methyl-1-phenyl-1H-pyrazol-5-yl)carbamoyl)thiophene- 3-diazonium hydrogen sulfate 20, 2-(methyl-(3-methylisoxazol-5-yl)carbamoyl)benzenediazonium hydrogen sulfate 21 and 2-(methyl(phenyl)carbamoyl)benzenediazonium hydrogen sulphate 22 were synthesized and reacted with a CuSO4/NaCl/ascorbic acid combination to give complex mixtures. The structures of the reaction products were elucidated, depending upon the pathways followed. Compound 20 almost exclusively afforded an Ar-5 cyclization product and trace amounts of the product derived from a competing Ar-6 Pschorr closure. In the case of compound 21, the Ar-6 cyclization was not observed, while the Ar-5 cyclization and 1,5-hydrogen radical transfer processes equally occurred. Finally, the Ar-6 cyclization was observed as the main process for compound 22, accompanied by the Ar-5 cyclization and, in a much lesser extent, by an 1,5-hydrogen radical transfer process.INTRODUCTION
Hanson and co-workers described the use of a combination of CuSO4 and NaCl in the presence of ascorbic acid to perform the Sandmeyer reaction of 4-chlorobenzenediazonium chloride in a homogeneous aqueous phase, leading to 1,4-dichlorobenzene.1 Ascorbic acid serves to reduce Cu(II) to Cu(I) which, in turn, reduces the diazonium ion to a diazenyl radical. The latter decomposes to dinitrogen and a 4-chlorophenyl radical. Complexes of Cu(II) ions with chloride ions then transfer a chlorine atom to the 4-chlorophenyl radical to afford the final product.
We found in previous studies that, under the foregoing conditions, diazonium salt 2 (Scheme 1) affords neither the product of the classical Sandmeyer reaction, chloro-derivative 3, nor the expected product of a competing Pschorr ring closure, tricyclic derivative 4. On the contrary, a mixture of epimeric products 7, presumably via radical intermediates 5 and 6, were obtained as the major reaction products.2 We also isolated the derivative 9, due to a 1,5-hydrogen atom transfer process, and trace amounts of the hydroxy spiro epimers 8.3
When we transformed, under the above conditions, the diazonium salt 10 (Scheme 2) derived from 4-amino-N-1,3-trimethyl-N-(3-methyl-1-phenyl-1H-pyrazol-5-yl)-1H-pyrazole-5-carboxamide,4 a different reaction pattern was observed. In fact, in this case we isolated the chlorinated spiro derivatives 14 and the product of the Pschorr ring closure 12 together with its structural isomer 16.
In continuing research on this type of reaction,2-5 and in order to establish the role of the aromatic moieties, linked by the amide bridge on the reaction pathway we became interested in investigating the transformation of diazonium salts 20–22 derived from the corresponding amines 17–19 (Figure 1).
RESULTS AND DISCUSSION
The amino-derivatives 17–19 were synthesized accordingly to Scheme 3.
Compound 17 was obtained by reduction of nitro-derivative 26 with Fe/5% AcOH; compound 26 is the product of the methylation of 25, which in turn arises from the coupling reaction between amine 24 and the acyl chloride 23.
A slightly modified procedure in which the final reduction step was performed with SnCl2, was employed for the synthesis of the amine 18. Finally, the known amine 19 was prepared from the nitro-derivative 35 accordingly to a reported procedure.6
The diazonium compounds 20–22 were prepared from the corresponding amines and subsequently subjected in situ to the reaction conditions suitable for the generation of the aryl radical (CuSO4, NaCl, ascorbic acid).1,2 After stirring 1 hour at rt, the products reported in Scheme 3 and Table 1 were isolated. Together with some data already reported by some of us,2,4 the following discussion also includes new computational data concerning compounds 2 and 10 for an useful comparison.
From the analysis of the structures of reaction products it is possible to gain information on the chemical behavior of the radical intermediates involved in the reaction pathways.
The initially originated 2-aryl radicals 40–42, subsequently follow the transformations depicted in Scheme 4. The intramolecular cyclization, to give second generation tricyclic radicals, can follow two possible paths: a 5-exo-trig closure (path a) giving rise to the spiro systems 6, 43–45 (in the following discussion collectively referred to as I) and a 6-endo-trig closure (path b) giving rise to the tri-cyclic systems 46–48 (II). Another possibility is the occurrence of a 1,5-hydrogen shift (path c) from the N-methyl group to the aryl center to give the methylene radicals 49–51 (III).
It was found that all of the diazonium salts 2, 10, 20–22 follow the path a whereas the path b is only followed by 10, 20 and 22 (Schemes 1–4). Moreover, path a is the dominant one for all of the cases but the compound 22, that mostly follows the way b giving compound 36 as major product, through the radical 48. This result appears to be in accordance with the Baldwin's rules8 relying with the closure mode of simple aliphatic radicals, i.e. 5-exo-trig closure is dominant with respect to the 6-endo-trig, mainly on the ground of sterical reasons. When the cyclization process involves the radical's addition to an aromatic moiety, a competition between the two pathways does occur.9,10 In these cases, despite the 5-exo-trig – or “Ar-5” – cyclization is still the one kinetically preferred, 6-member rings containing final products of the type 6-endo-trig – or “Ar-6” – are obtained. This is likely due to the occurrence of alternative reaction pathways such as the oxidation of the radical to a cation, followed by a rearrangment of the latter. It is not surprising that in the case of the hetero-aromatic systems reported in this work the products derived from the spiro radicals are mainly obtained. This is due to the quite less aromatic character (and as a consequence the more localized nature of the π electron density in the C-4, C-5 formal double bond11) of the hetero-aromatic ring acceptor. On the other hand, the Cu/promoted Pschorr radical 6-endo-trig cyclisation to give both phenanthrene9 and phenanthridone12 systems are well documented.
It is worthy of further comment that we did not find products derived from a 6-exo (or “Ar-6”) mode of closure in the case of compound 22 (Scheme 1), as instead is the case for the cited compound 22 and for compounds 10 and 20 (Schemes 2 and 3) that gave the “Ar-6” products 12 and 28 in trace amounts, through the radicals 46. This is probably explainable with the oxidation by Cu2+ of the radical 45 to the corresponding spiro cation (Scheme 6), which can account for the formation of compound 36. The higher reactivity of the electron-rich pyrazolinyl radicals 43a,b and 6 makes the subsequent reaction with (CuCln)2-n complexes (see discussion below) faster than the oxidation.
In order to gain a better understanding of the radical's transformation processes described above, we evaluated the thermodinamic of the reaction courses depicted in the Scheme 4 by means of computational methods based on Density Functional theory. In particular the free energy variations (ΔG°) were evaluated by taking into consideration the conformations of the aryl radicals 40–42 with the higher degree of similarity between reactant and product (Scheme 5): C1 is the conformation related to both the radical closures, while C2 is the one related to the hydrogen shift process.
Table 2 reports the estimation of the ΔG° values referred to the conformations C1 and C2; the former is the one largely dominant in every case but for the radical 41b; in this case both the conformations can be considered equally populated, within the accuracy limit of the computational method employed. From the data reported in Table 2, it is evident as the conclusion that can be inferred from a thermodinamical control of the investigated reactions are in line with those deduced from the kinetic behaviour.
The evolution of the cyclized radical is related to the relative trend to give one of the four possible radical quenching processes: i) The ligand transfer reaction with (CuCln)2-n complexes to give chloro-substituted derivatives. ii) The direct cyclization to give an aromatic system (Pschorr reaction). iii) The radical coupling to give a dimer. iv). The oxidation by Cu2+, without Cl transfer, to give a cation. A “non-classical”2 Sandmayer reaction (i) is given by the radicals I arising from all of the compounds but 21 (Scheme 6). Further, while the compounds 2, 10 and 20 give predominantly the chloro spiro-derivatives 7, 14 and 27, compound 22 give the 6-Ar cyclization compound 36 as the main product together with the epimeric alcohols trans- and cis-37 and trace amount of the ketone 38 (Schemes 3 and 4).
This result reflects the relative electronic character, and as a consequence the nucleophilicity, of the radicals I. The fact that an alcohol is isolated in the case of 22, without any trace of chloro compounds, can be rationalised by the intervention of a fast oxidation step. The formation of the product 36 is easily explained by the known tendency of the five member cyclic cation to quickly rearrange to the six member one.9 Further, the reaction pathway proposed in Scheme 6 for the intermediate 45 is supported by the reported evidence of a higher Cl/H2O+ transfer ratio by any [Cu(II)Cln(H2O)m](2-n) species.13 In the case of compound 21, it can be argued that the reaction of the radical 44 (Schemes 4 and 3) with any Cu species is much slower than the dimerization, giving the product 33. The formation of compound 34 is easily explained by the sequence reported in Scheme 6.
The reaction pathway c – 1,5-hydrogen atom shift – is mainly followed by the radical 41b arising from the precursors 21 and, in a much lower extent, by the radical 42 formed from 22 (Schemes 4 and 3). Both the radicals 50b and 51 given by 41b and 42 bring to the N-demethylated products 32 and 39 through one or both the mechanisms depicted in Scheme 7.
The ionic mechanism should be favored by the intrinsic stability of the immonium ion14 originated by the Cu(II) oxidation of the radicals 50b and 51. In the case of 21, this process completely outclasses the oxidation/rearrangement steps, that are instead dominant in the case of 22. This significant difference can be explained on the ground of the above cited conformational freedom of the radicals arising from 21 with respect to 22 (Schemes 3 and 4). The calculated data reported in tab. 2: ΔG° (C1 → C2) 2.7 KJ mol-1 for 41b vs. 17.4 KJ mol-1 for 42, support this statement.
CONCLUSIONS
The products distribution of the radicals mediated transformation of a set of aromatic diazonium compounds is fairly complex but explainable on the ground of the several reaction pathways accessible by the intermediates involved.
The initial aryl radical gives rise mainly to a 5-exo-trig (or Ar-5) cyclisation when the radical attacks a hetero-aromatic system (compounds 2, 10, 20, 21). On the other hand, when the attack involves a benzenic ring (compound 22), the intervention of a more complex oxidative pathway makes the Ar-6 cyclization mode the one dominant, yielding the phenanthridone-like compound 36 as the main reaction product. An 1,5-hydrogen shift side reaction occurs in some cases and is quite relevant in the case of compound 21. Both kinetic and thermodinamic based arguments can account for the experimental evidences collected. The final products structure depends also on the particular reaction course followed by the radical intermediates.
Despite its complexity, the reaction pattern described in the present study is on the whole internally coherent, rightly integrated within the body of literature evidences concerning the diazonium compound's radical chemistry and give a further insight into the class of the hetero-aromatic systems. A contribution to the general radical cyclisation rules can be added, i.e. radicals of the general pattern showed below follow the “Ar-5” closure path rather than the “Ar-6” one (Figure 2).
Finally, all of the spiro derivatives reported in the present study can be considered as potentially active biological agents because many compounds that possess a spirooxyindole moiety were shown to exhibit significant biological activity.15
EXPERIMENTAL
Generals
Reaction progress was monitored by TLC on silica gel plates (Merck 60, F254, 0.2 mm). Organic solutions were dried over Na2SO4. Evaporation refers to the removal of solvent on a rotary evaporator under reduced pressure. All melting points were determined on a Büchi 530 capillary melting point apparatus and are uncorrected. IR spectra were recorded with a Perkin Elmer Spectrum RXI FT-IR System spectrophotometer as solid in KBr disc or nujol. 1H NMR and 13C NMR spectra of all compounds but 27-cis/trans were obtained in CDCl3 or DMSO-d6 solutions on a Bruker AC 250 F or Bruker AC series 300 spectrometers. 1H NMR and 13C NMR spectra of compounds 27-cis/trans were obtained on a Bruker Avance II 400 spectrometer. In all cases tetramethylsilane was used as an internal standard; chemical shifts are expressed in δ values (ppm). Mass spectra at 70 eV were obtained using an 3400-CX Varian gas chromatograph fitted with a Saturn 3 mass spectrometer. For compound 33 the mass spectra was obtained by a Shimadzu LCMS2010 apparatus. Merck silica gel (Kiesegel 60/230-400 mesh) was used for flash chromatography (FC) columns. Yields refer to products after one crystallization. The name of the compounds were obtained using the ChemDraw Ultra 12.0 software.
Computational Details
All calculations have been performed in the framework of density functional theory by using the Gaussian 09 software.16 The Becke 3-parameters hybrid functional B3LYP,17 consisting of Becke exchange functional plus the Lee, Yang and Parr correlation functional has been used joined with the Dunning's correlation consistent polarized valence double zeta (cc-pVDZ). The geometry optimizations have been followed by the calculation of the harmonic frequencies, in order to verify the nature of minimum in the potential energy surface of the structure obtained and to calculate the zero-point vibrational contribution to the energy. The molecular Gibbs free energies of formation have been calculated by adding the estimated zero-point, thermal and entropic contributions (T = 298 K, p = 1 bar) to the SCF energy.
N-(3-Methyl-1-phenyl-1H-pyrazol-5-yl)-3-nitrothiophene-2-carboxyamide (25)
To a solution of commercially available 3-methyl-1-phenyl-1H-pyrazol-5-amine (17.3 g, 0.1 mol) in dry CHCl3 (450 mL), 3-nitrothiophene-2-carbonyl chloride (19.2 g, 0.1 mol) was added; the resulting solution was refluxed for 5 h. After the first hour, triethylamine (14 mL) was added in four portions (7, 3.5, 2 x 1.75 mL respectively, with intervals of 1 h between additions). Then the reaction was quenched with ice cold water. The organic phase was dried onto Na2SO4 and solvent was evaporated. The residue was crystallized from EtOH to give compound 25 (50%); a yellow orange solid; mp 140–143 °C (EtOH); ΙR (Nujol) cm-1 3193 (NH), 1678 (CO); 1H NMR (250 MHz, CDCl3) δ 2.36 (3H, s, Me), 6.72 (1H, s, pyrazole H-4), 7.46-7.66 (7H, m, phenyl and thienyl protons), 10.75 (1H, br s, exchangable with D2O); 13C NMR (75 MHz, CDCl3) δ 13.49, 98.42, 124.95 (2C), 126.43, 128.31, 129.13, 129.29 (2C), 134.97, 136.93, 139.41, 142.85, 149.21, 154.09; MS m/z 328 (M+). Anal. Calcd for C15H12N4O3S: C, 54.87; H, 3.68; N, 17.06). Found: C, 55.01; H, 3.81; N, 17.26.
3-Amino-N-methyl-N-(3-methyl-1-phenyl-1H-pyrazol-5-yl)thiophene-2-carboxyamide (17)
In a three neck round bottomed flask, compound 25 (32.8 g, 0.1 mol) was solubilized in hot acetone (360 mL), then KOH (21.87 g, 0.39 mol) was added in one portion and a solution of methyl iodide (9.27 mL) in acetone (50 mL) was added dropwise. The reaction mixture was refluxed for 1 h. The resulting suspension was filtered off and the obtained solution evaporated. Finally, the solid residue was crystallized from EtOAc to give N-methyl-N-(1-phenyl-3-methyl-1H-pyrazol-5-yl)-3-nitrothiophene- 2-carboxyamide (26) (23%) as a yellow solid (mp 90–93 °C) and directly employed in the subsequent step after partial characterization by proton NMR. 1H NMR (250 MHz, CDCl3) δ 2.21 (3H, s, Me), 3.46 (3H, s, Me), 6.08 (1H, s, pyrazole H-4), 7.30-7.44 (7H, m, phenyl and thienyl protons).
Powdered Fe (80 g, 1.4 mol) was added to 100 mL of a 5% (v/v) aqueous acetic acid solution and stirred at 100 °C for 15 min. Then N-methyl-N-(1-phenyl-3-methyl-1H-pyrazol-5-yl)-3-nitrothiophene-2- carboxyamide (26) (44.5 g, 0.13 mol) was added within 1 h. The reaction mixture was stirred for 1 h more and then the pH was raised to 8 by adding aq. NaHCO3(sat.). The mixture was filtered, the solid material washed with water until neutrality, dried by standing overnight at rt and extracted with boiling CHCl3 (3 x 150 mL). The solution was dried onto sodium sulphate and evaporated. The solid residue was crystallized from EtOH to give compound 17 (80%); a pale yellow solid; mp 120–122 °C; ΙR (nujol) cm-1 3488 and 3371 (NH2), 1618 (CO); 1H NMR (250 MHz, CDCl3) δ 2.37 (3H, s, Me), 3.17 (3H, s, Me), 4.16 (2H, s br. exchangeable with D2O, NH2); 6.20 (1H, s, pyrazole H-4), 6.43-7.44 (7H, m, phenyl and thienyl protons); 13C NMR (75MHz, CDCl3) δ 14.22, 37.36, 100.95, 106.31, 119.12, 122.88 (2C), 127.38, 129.11, 131.01 (2C), 138.40, 141.16, 149.29, 155.96, 165.92; MS m/z 312 (M+). Anal. Calcd for C16H16N4OS: C, 61.52; H, 5.16; N, 17.93. Found: C, 61.72; H, 5.32; N, 18.17.
2-Amino-N-methyl-N-(3-methylisoxazol-5-yl)benzamide (18)
N-Methyl-(3-methylisoxazol-5-yl)-2-nitrobenzamide (6.70 g, 0.026 mol), previously prepared following the literature procedure,18 were added to a continuously stirred suspension of SnCl2 (14.60 g, 0.077 mol) in HClconc. (30 mL) while keeping the reaction temperature below 10 °C with an ice bath. The final suspension was further stirred for 24 h. Then the reaction was quenched with iced water (300 mL) and basified by adding 125 mL of a 40% (w/v) NaOH solution. Then the reaction mixture was extracted with EtOAc (3 x 250 mL), and the organic phase was washed with water, dried with Na2SO4 and evaporated. The solid residue was crystallized from EtOH to give compound 18 (65%), a colourless solid; mp 70–73 °C; IR (KBr) cm-1 3481 and 3369 (NH2), 1648 (CO); 1H NMR (250 MHz, CDCl3) δ 2.19 (s, 3H, Me), 3.45 (s, 3H, Me), 4.78 (br s, 2H, exchangeable with D2O, NH2), 5.77 (s, 1H, isoxazole 4-H), 6.65-7.27 (m, 4H, C6H4); 13C NMR (75 MHz, CDCl3) δ 11.91, 35.54, 93.89, 116.94, 117.07, 117.11, 128.88, 132.21, 147.10, 163.34, 164.73, 170.00; MS m/z 231 (M+). Anal. Calcd for C12H13N3O2: C, 62.33; H, 5.67; N, 18.17. Found: C, 62.51; H, 5.90; N, 18.40.
Preparation and transformation of 2-(methyl-(3-methyl-1-phenyl-1H-pyrazol-5-yl)carbamoyl)thiophene-3-diazonium hydrogen sulfate (20)
The solution of compounds 20 was prepared from the corresponding amines 17 (2 g, 6.41 mmol) by treating with 2.5 M H2SO4 (12.8 mL) and 2.5 M NaNO2 (2.65 mL) while keeping temperature at 0–5 °C. The transformation reaction was carried out following the procedure of Hanson1 which has been modified by us.2 After 15 min the resulting solution of 20 was diluted with 305 mL of a cold (0–5 °C) solution of CuSO4∙5H2O (0.3 M) and sodium chloride (0.75 M). Ascorbic acid was then added (0.28 g, 1.59 mmol) and stirring was continued for 1 h at room temperature. The suspension was filtered off and the solid residue was dried between porous plates and subsequently chromatographed following the FC procedure:19 external diameter column 5 cm, EtOAc/light petroleum (bp 40–70 °C) (3:7 v/v) as eluent, silica gel (170 g), fractions each 50 mL. Fractions 16-38 were evaporated, and the residue was crystallized to give compounds 27 (trans/cis 1.2:1 mixture).
The partial separation of the epimers was obtained operating a very fast FC with a Biotage® apparatus, using Et2O/light petroleum (bp 40–70 °C) as eluent. Compound cis-27 was obtained pure, whereas trans-27 was contaminated with about 15% of cis isomer, as judged by 1H NMR. cis-4-Chloro-5,5’-dimethyl-2-phenyl-2,4-dihydrospiro[pyrazole-3,4-thieno[2,3-c]pyrrol]-6(5’H)-one (cis-27):20 16%; a pale yellow solid; mp 178–180 °C (EtOH); IR (KBr) cm-1 1697 (CO); 1H NMR (400 MHz, CDCl3) δ 2.25 (s, 3H, Me), 2.67 (s, 3H, Me), 5.25 (s, 1H, pyrazoline H-4), 6.79 (d, J = 7.0 Hz, 2H), 6.90 (t, J = 5.9 Hz, 1H), 7.09-7.15 (m, 3H), 7.76 (d, J = 4.5 Hz, 1H); 13C NMR (100 MHz, CDCl3) δ 13.39, 27.27, 67.35, 86.58, 115.68 (2C), 119.52, 122.28, 129.05 (2C), 136.44, 136.73, 143.06, 146.97, 153.95, 162.57. Anal. Calcd for C16H14ClN3OS: C, 57.91; H, 4.25; N, 12.66. Found: C, 57.75; H, 4.50; N, 12.50.
trans-4-Chloro-5,5’-dimethyl-2-phenyl-2,4-dihydrospiro[pyrazole-3,4-thieno[2,3-c]pyrrol]-6(5’H)- one (trans-27):20,21 IR (Nujol) cm-1 1702 (CO); 1H NMR (400 MHz, CDCl3) δ 2.26 (s, 3H, Me), 2.80 (s, 3H, Me), 4.94 (s, 1H, pyrazoline H-4), 6.72 (d, J = 7.1 Hz, 2H), 6.89 (t, J = 6.0 Hz, 1H), 7.07-7.17 (m, 3H), 7.66 (d, J = 4.3 Hz, 1H); 13C NMR (100 MHz, CDCl3) δ 13.43, 25.69, 66.76, 87.86, 116.24 (2C), 122.29, 122.46, 128.95 (2C), 134.57, 136.44, 142.39, 146.85, 151.17, 162.30. Anal. Calcd for C16H14ClN3OS: C, 57.91; H, 4.25; N, 12.66. Found: C, 57.85; H, 4.54; N, 12.52.
Fractions 66-94 of the first cromatographic procedure afforded a residue which was crystallized from EtOH to give 1,4-dimethyl-3-phenyl-3H-pyrazolo[3,4-b]thieno[3,2-d]pyridine-5(4H)-one (28) (2.1%); a colourless solid, mp 195–196 °C; IR(KBr) cm-1 1636 (CO); 1H NMR (250 MHz, (CDCl3) δ 2.63 (3H, s, Me), 3.29 (3H, s, Me), 7.44-7.84 (7H, m, C6H5 and thiophene protons); 13C NMR (75MHz, CDCl3) δ 13.74, 31.76, 102.74, 121.52, 125.14, 127.54 (2C), 129.28 (2C), 129.47, 134.89, 138.33, 139.67, 142.16, 143.19, 158.55; ΜS m/z 295 (M+). Anal. Calcd for C16H13N3OS: C, 65.06; H, 4.44; N, 14.23. Found: C, 65.16; H, 4.62; N, 14.37.
Preparation and trasformation of 2-(methyl-(3-methyl-isoxazol-5yl)carbamoyl)benzenediazonium hydrogen sulfate (21)
The solution of compounds 21 was prepared from the corresponding amine 18 (3 g, 12.98 mmol) by treating with 2.5 M H2SO4 (26 mL) and 2.5 M NaNO2 (5.37 mL) while keeping temperature at 0–5 °C. The transformation reaction was carried out following the procedure of Hanson1 which has been modified by us.2 After 15 min the resulting solution of 21 was diluted with 620 mL of a cold (0–5 °C) solution of CuSO4∙5H2O (0.3 M) and sodium chloride (0.75 M). Ascorbic acid was then added (0.57 g, 3.24 mmol) and stirring was continued for 1 h at room temperature. The mixture was extract with EtOAc (3 x 250 mL) and the organic phase was washed with water, dried with Na2SO4 and evaporated. The residue was washed with EtOAc (2.5 mL) and the suspension filtered off affording a quasi-pure solid product which was crystallized from EtOH to give 4’-(2,3’-dimethyl-3-oxo-4’H-spiro[isoindoline-1,5’-isoxazol]- 4’-yl)-2,3’-dimethyl-4’H-spiro[isoindoline-1,5’-isoxazol]-3-one (33) (6.4%); a colourless solid; mp > 270 °C; IR (KBr) cm-1 1724, 1711; 1H NMR (250 MHz, CDCl3) δ 2.34 (s, 6H, 2x Me), 2.81 (s, 6H, 2xMe), 3.83 (s, 2H, isoxazolines 4-H), 7.37-7.52 (m, 8H, 2xC6H4); 13C NMR (75MHz, CDCl3) δ 14.54, 24.37, 52.75, 101.11, 123.47, 124.53, 130.98, 131.93, 132.25, 138.04, 155.88, 164.86; MS m/z 431 ( M+1). Anal. Calcd for C24H22N4O4: C, 66.97; H, 5.15; N, 13.02. Found: C, 66.75; H, 5.20; N, 13.29.
The mother liquors of the above suspension were evaporated and the residue chromatographed following the FC procedure:19 external diameter column 5 cm, EtOAc/light petroleum (bp 40–70 °C) ( 3:7 v/v) as eluent, silica gel (192 g), fractions each 50 mL. Fractions 15-23 were evaporated, and the residue was crystallized from EtOAc/light petroleum to give a compound which was identical in all respects (MS, 1H-NMR, mixed mp, TLC) with an authentic specimen of N-methylphthalimide (34) (14.4%). Fractions 31-36 left a residue which was crystallized from EtOH to give N-(3-methyl-isoxazol-5-yl)benzamide (32)22 (13.3%); mp 144–146 °C; IR (KBr) cm-1 3344 (NH), 1677 (CO); 1H NMR (250 MHz, CDCl3) δ 2.28 (s, 3H, CH3), 6.39 (s, 1H, pyrazole H-4), 7.47-7.92 (m, 5H, C6H5), 9.08 (br s, 1H, exchangeable with D2O, NH); MS m/z 203 (M+1).
Preparation and trasformation of 2-(methyl(phenyl)carbamoyl)benzenediazonium hydrogen sulphate (22)
The solution of compounds 22 was prepared from the corresponding amine 19 (3.39 g, 15 mmol) by treating with 2.5 M H2SO4 (30 mL) and 2.5 M NaNO2 (6.21 mL) while keeping temperature at 0–5 °C. The transformation reaction was carried out following the procedure of Hanson1 which has been modified by us.2 After 15 min the resulting solution of 22 was diluted with 714 mL of a cold (0–5 °C) solution of CuSO4∙5H2O (0.3 M) and sodium chloride (0.75 M). Ascorbic acid was then added (0.66 g, 3.75 mmol) and stirring was continued for 1 h at room temperature. The mixture was extract with EtOAc (3 x 250 mL) and the organic phase was washed with water, dried with Na2SO4 and evaporated. The residue was chromatographed following the FC procedure18: external diameter column 5 cm, EtOAc/light petroleum (bp 40–70 °C) (4:6 v/v) (3 L) and 6:4 v/v (3 L) as eluents, silica gel (230 g), fractions each 50 mL.
Fractions 15-23 were evaporated, and the residue was crystallized from cyclohexane to give 5-methylphenanthridine-6(5H)-one (36)6 (25%); mp 104–105 °C (benzene/light petroleum (bp 40–70 °C)); 1H NMR (250 MHz, DMSO-d6) δ 3.72 (s, 3H, Me), 7.32-8.52 (m, 8H, 2 x C6H4); MS m/z 209 (M+).
Fractions 24–33 left a residue which was crystallized from EtOAc to give N-phenylbenzamide (39) (3.4%); mp 156–58 °C (lit.,24 160–161 °C from EtOH); 1H NMR (300 MHz, DMSO-d6) δ 7.07-7.97 (m, 10H, 2 x C6H5), 10.23 (s, 1H, exchangeable with D2O, NH); MS m/z 197 (M+).
Further eluition gave fractions 46–58 from which a residue was obtained. Crystallization of the residue gave 2-methylspiro[cycloexa[2,5]diene-1,1’-isoindoline]-3’,4-dione (38) (3%); a colouless solid, mp 213–215 °C (EtOAc/light petroleum bp 40–70 °C); IR (KBr) cm-1 1708, 1664 (CO). 1H NMR (300 MHz, CDCl3) δ 2.88 (s, 3H, Me), 6.51 (d, J = 8.1 Hz, 2H, cycloexadiene protons), 6.75 (d, J = 8.2 Hz, 2H, cycloexadiene protons), 7.38 (d, J = 5.7 Hz, 1H, phenyl proton), 7.63 (m, 2H, phenyl protons), 7.82 (d, J = 7.8, 1H, phenyl proton); 13C NMR (300 MHz, CDCl3) δ 25.69, 64.29, 122.87, 123.57, 129.63, 131.16 (2C), 131.80, 132.50, 141.37, 147.64 (2C), 166.91, 184.17. Anal. Calcd for C14H11NO2: C, 74.65; H, 4.92; N, 6.22). Found: C, 74.42; H, 5.06; N, 6.30.
Continuing eluition, fractions 70-81 and 82-114 were collected. Evaporation left residues wich were crystallized from EtOAc to give epimers trans-37 and cis-37 respectively.
trans-4-Hydroxy-2’-methylspiro[cycloexa[2,5]diene-1,1’-isoindolin]-3’-one (trans-37):20 (3%); a colourless solid, mp 120–122 °C (EtOAc); IR (KBr) cm-1 1665 (CO); 1H NMR (300 MHz, DMSO-d6) δ 2.74 (s, 3H, Me), 4.55 (m, 1H, cycloexadiene proton), 5.35 (m, 3H, one exchangeable with D2O, OH and cycloexadiene protons), 6.28 (dd, J = 8.5, 1.9 Hz, 2H, cycloexadiene protons), 7.42-7.71 (ABCD m, 4H, C6H4); 13C NMR (75 MHz, DMSO-d6) δ 24.67, 59.78, 62.69, 122.58, 123.27, 126.93 (2C), 128.63, 131.06, 131.83, 134.81 (2C), 147.42, 166.44. Anal. Calcd for C14H13NO2: C, 73.99; H, 5.77; N, 6.16. Found: C, 74.12; H, 5.96; N, 6.30.
cis-4-Hydroxy-2’-methylspiro[cycloexa[2,5]diene-1,1’-isoindolin]-3’-one (cis-37):21 (6%); a colourless solid, mp 189–191 °C (EtOAc); IR (KBr) cm-11682 (CO); 1H NMR (300 MHz, DMSO-d6) δ 2.82 (s, 3H, Me), 4.69 (br s, 1H, cycloexadiene proton), 5.38 (d, J = 9.9 Hz, 2H, cycloexadiene protons), 5.42 (d, J = 6.6 Hz, 1H, exchangeable with D2O, OH), 6.23 (d, J = 9.9 Hz, 2H, cycloexadiene protons), 7.26 (d, X part of an ABCX pattern, J = 9.0 Hz, 1H, phenyl proton), 7.53-7.75 (ABC part m, 3H, phenyl protons); 13C NMR (75 MHz, DMSO-d6) δ 24.71, 59.68, 62.41, 122.29, 122.65, 125.85 (2C), 128.30, 130.69, 131.58, 133.74 (2C), 147.43, 166.18. Anal. Calcd. for C14H13NO2: C, 73.99; H, 5.77; N, 6.16. Found: C, 74.01; H, 5.86; N, 6.35.
ACKNOWLEDGEMENTS
Financial support from “Fondo di Finanziamento della Ricerca di Ateneo (ex 60%)” and PON a3_00053-PLASS are gratefully acknowledged. 1H (400 MHz) and 13C (100 MHz) NMR data were provided by Centro Grandi Apparecchiature – UNINetLab – Università di Palermo funded by P.O.R. Sicilia 2000-2006, Misura 3.15 Quota Regionale.
References
1. P. Hanson, J. R. Jones, B. C. Gilbert, and A. W. Timms, J. Chem. Soc., Perkin Trans. 2, 1991, 1009. CrossRef
2. G. Daidone, B. Maggio, D. Raffa, S. Plescia, F. Benetollo, and G. Bombieri, J. Chem. Soc., Perkin Trans. 1, 1998, 2891. CrossRef
3. B. Maggio, D. Raffa, M. V. Raimondi, and G. Daidone, Molecules, submitted.
4. B. Maggio, G. Daidone, D. Raffa, S. Plescia, G. Bombieri, and F. Meneghetti, Helv. Chim. Acta, 2005, 88, 2272. CrossRef
5. B. Maggio, D. Raffa, M. V. Raimondi, S. Cascioferro, S. Plescia, M. A. Sabatino, G. Bombieri, F. Meneghetti, and G. Daidone, ARKIVOC, 2008, xvi, 130. CrossRef
6. R. A. Heacock and D. H. Hey, J. Chem. Soc., 1952, 1508. CrossRef
7. Formed as mixtures of epimers.
8. J. E. Baldwin, J. Chem. Soc. Chem. Comm., 1976, 734. CrossRef
9. P. Hanson, P. W. Lövenich, S. C. Rowell, P. H. Walton, and A. W. Timms, J. Chem. Soc., Perkin Trans. 2, 1999, 49. CrossRef
10. J. C. Chottard and M. Julia, Tetrahedron, 1972, 28, 5615. CrossRef
11. A. R. Katritzky and J. M. Lagowski, ‘Comprehensive Heterocyclic Chemistry’, vol. 5, ed. by K. T. Potts, Pergamon Press, 1984, part 4A, pp. 1–38.
12. D. H. Hey and T. M. Moynehan, J. Chem. Soc., 1959, 1563. CrossRef
13. P. Hanson, R. C. Hammond, B. C. Gilbert, and A. W. Timms, J. Chem. Soc., Perkin Trans. 2, 1995, 2195. CrossRef
14. A. H. Lewin, A. H. Dinwoodie, and T. Cohen, Tetrahedron, 1966, 22, 1527. CrossRef
15. D. Chunhui, L. Bio, and R. J. S. Corey, Beilstein J. Org. Chem., 2012, 8, 986 and references cited therein. CrossRef
16. Gaussian 09, Revision C.01, M. J. Frisch, G. W. Trucks, H. B. Schlegel, G. E. Scuseria, M. A. Robb, J. R. Cheeseman, G. Scalmani, V. Barone, B. Mennucci, G. A. Petersson, H. Nakatsuji, M. Caricato, X. Li, H. P. Hratchian, A. F. Izmaylov, J. Bloino, G. Zheng, J. L. Sonnenberg, M. Hada, M. Ehara, K. Toyota, R. Fukuda, J. Hasegawa, M. Ishida, T. Nakajima, Y. Honda, O. Kitao, H. Nakai, T. Vreven, J. A. Montgomery, Jr., J. E. Peralta, F. Ogliaro, M. Bearpark, J. J. Heyd, E. Brothers, K. N. Kudin, V. N. Staroverov, R. Kobayashi, J. Normand, K. Raghavachari, A. Rendell, J. C. Burant, S. S. Iyengar, J. Tomasi, M. Cossi, N. Rega, J. M. Millam, M. Klene, J. E. Knox, J. B. Cross, V. Bakken, C. Adamo, J. Jaramillo, R. Gomperts, R. E. Stratmann, O. Yazyev, A. J. Austin, R. Cammi, C. Pomelli, J. W. Ochterski, R. L. Martin, K. Morokuma, V. G. Zakrzewski, G. A. Voth, P. Salvador, J. J. Dannenberg, S. Dapprich, A. D. Daniels, Ö. Farkas, J. B. Foresman, J. V. Ortiz, J. Cioslowski, and D. J. Fox, Gaussian, Inc., Wallingford CT, 2009.
17. (a) A. D. Becke, J. Chem. Phys., 1993, 98, 1372; CrossRef (b) C. Lee, W. Yang, and R. G. Parr, Phys. Rev. B, 1988, 37, 785; CrossRef (c) P. J. Stephens, J. F. Devlin, C. F. Chabalowsky, and M. J. Frisch, J. Phys. Chem., 1994, 98, 11623. CrossRef
18. M. L. Baajardi, G. Daidone, D. Raffa, and S. Plescia, Heterocycles, 1986, 24, 1367. CrossRef
19. W. C. Still, M. Kahn, and A. Mitra, J. Org. Chem., 1978, 43, 2923. CrossRef
20. The relative stereochemistry was deduced on the ground of the supposed deshielding effect due to the magnetic anisotropy of the aromatic ring on the CHOH and CHCl protons of cis-37 and cis-27 respectively, compared with the trans isomers. This hypothesis was supported by the values of 1H shielding tensor computed for the four compounds at the optimized geometry (available in the supporting material) by using the B3LYP/aug-cc-pvdz level: observed vs calculated proton Δδ: 0.12 vs. 0.13 ppm for 37 and 0.50 vs. 0.31 for 27.
21. NMR values were obtained by extrapolation of the mixture spectra.
22. S. I. Sviridov, A. A. Vasil’ev, and S. V. Shorshnev, Tetrahedron, 2007, 63, 12195. CrossRef
23. C. N. Webb, Org. Synth., 1941, 1, 82.