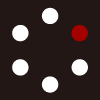
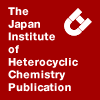
HETEROCYCLES
An International Journal for Reviews and Communications in Heterocyclic ChemistryWeb Edition ISSN: 1881-0942
Published online by The Japan Institute of Heterocyclic Chemistry
e-Journal
Full Text HTML
Received, 17th October, 2013, Accepted, 25th November, 2013, Published online, 10th December, 2013.
DOI: 10.3987/COM-13-12867
■ One-Pot Synthesis of 3,5-Diphenyl-1H-pyrazoles from Chalcones and Hydrazine under Mechanochemical Ball Milling
Ze Zhang,* Ya-Jun Tan, Chun-Shan Wang, and Hao-Hao Wu
School of Biological & Chemical Engineering, Anhyui Polytechnic University, No. 8, Beijing Road, Wuhu 241000, China
Abstract
A highly efficient and environmentally friendly method has been developed for facile synthesis of 3,5-diphenyl-1H-pyrazoles under mechanochemical ball-milling conditions. The advantages of short reaction time, high efficiency, no separation of in situ generated intermediate, using cheap sodium persulfate as the oxidant, together with very simple work-up procedure, make this one-pot and solvent-free protocol a green and powerful alternative to traditional methods for the synthesis of these kinds of compounds.INTRODUCTION
It is well known that pyrazole scaffold has been contributing more and more in biological,1 pharmacological,2 food,3 agrochemical fields4 and so on. Due to its scarcity in nature, various methods have been developed for the synthesis of pyrazoles, among which the most commonly used strategies are: (a) condensation of 1,3-diketones with hydrazines; (b) 1,3-dipolar cycloadditions of terminal alkynes onto diazo compounds generated from aldehyde and tosyl hydrazide; (c) reaction of α,β-unsaturated carbonyl compounds with hydrazines followed by further oxidation. But if a diversity-oriented synthesis of pyrazoles is desired, method (a) would be somewhat cumbersome as each 1,3-diketone must be purified and such obtained pyrazole is often a mixture of condensation products. As to method (b), the process involves diazo compounds, which are well known to be toxic and potentially explosive. Although various examples have been reported for the synthesis of pyrazoles from α,β-unsaturated carbonyl compounds by method (c), there are still drawbacks such as rigorous or hazardous conditions, intractable side reactions, use of organic solvent, long reaction time, tedious procedures and so on. To circumvent these problems, one of the best alternatives is to carry out this kind of reactions under solvent-free conditions. Among current solvent-free techniques, mechanical ball-milling has recently been contributing more and more for efficient promotion of various organic reactions in a greener way.5,6
Considering the requirement of green chemistry, we have also successfully applied the mechanochemistry technique in some organic transformation in recent years.7 Herein, we want to report a one-pot and solvent-free mechanochemical synthesis of various 3,5-diphenyl-1H-pyrazoles from chalcones and hydrazine, which can be used as a molecular scaffold for the synthesis of apoptosis-inducing agents8 or as inhibitors of monoamine oxidases.9
RESULTS AND DISCUSSION
We firstly chose chalcone 1a as the model substrate to optimize the best reaction condition. A series of experiments were carried out in solution and under ball milling by varying the oxidant, the solvent and the reaction temperature, and the results are summarized in Table 1.
Under the condition of ball milling at room temperature, the addition-cyclization of hydrazine with chalcone 1a to form intermediate pyrazoline 3 can be almost completely accomplished within 40 minutes. Then we explored several oxidants to convert the in situ generated pyrazoline 3 into pyrazole 2a, and found that potassium permanganate and iodine afforded rather low chemoselectivity, in which pyrazoline 3 was essentially consumed but the yield of the desired product pyrazole 2a was very poor (entries 1, 2). This may be ascribed to side reactions resulted from too strong oxidizing capability of the oxidants. As to Mn(OAc)3 and Cu(OAc)2, they cannot promote the conversion of pyrazoline 3 into pyrazole 2a efficiently (entries 3, 4). In contrast, Ce(NH4)2(NO3)6 and Na2S2O8 gave a relatively good result, producing pyrazole 2a in good to excellent yield (entries 5, 6). But with a view to green synthesis including the factors such as yield, atom economy and cost, Na2S2O8 is undoubtedly the best oxidant for this transformation.
Using the optimized Na2S2O8 as the oxidant, we also performed several control experiments for this reaction in various solvents. At room temperature in all these solution cases, a relatively long time of two hours was needed for complete conversion of starting materials chalcone 1a into pyrazoline 3, which was obviously slower than that under ball milling condition. When Na2S2O8 was afterward added and further stirring was kept for two hours, the in situ generated pyrazoline 3 still remained in some extent. It was noted that strong polarity of the solvent favored the formation of the product pyrazole 2a. For example, DMF and DMSO (entries 11-14) gave better conversion than that of CH2Cl2 and THF. Under heating, the first addition-cyclization step became significantly rapid, in which chalcone 1a was consumed within 30 minutes. When Na2S2O8 was added and heating was kept for another hour, both the conversion of pyrazoline 3 and the yield of pyrazole 2a decreased to a certain extent. The reason may be that Na2S2O8 would decompose when heated in solution, making the desired usage amount of the oxidant insufficient. On the other hand, we found that even in the absence of any oxidant, pyrazoline 3 can be slowly converted into pyrazole 2a just by heating in solution. For example, when a mixture of chalcone 1a and hydrazine was directly refluxed for 12 hours in EtOH, chalcone 1a was also completely consumed, forming 30% pyrazoline 3 and 56% pyrazole 2a (entry 17). In general, the solvent-free condition of ball milling at room temperature has obvious advantages including short reaction time, high chemoselectivity and yield.
Inspired by the results as shown in Table 1, we then employed Na2S2O8 as the oxidant and applied the ball milling technique to access a wide variety of 3,5-diphenyl-1H-pyrazoles from various chalcones in a one-pot pathway. Under this mechanically promoted condition, the reactions proceeded cleanly without intermediate work-up and left no, or only traces of byproducts. The detailed results are outlined in Table 2.
From Table 2 it can be seen that the first addition-cyclization step proceeded very smoothly within one hour in all cases, and substituents in chalcones 1 have no significant effect on this step. This may be explained that the α,β-unsaturated carbonyl moiety in chalcone exhibits very strong electrophilicity, and hydrazine has both very strong nucleorphilicity and basicity. These facts make the addition-cyclization of chalcone with hydrazine fairly easy whether the substituent is electron-donating or electron-withdrawing. But during the second step for oxidation of in situ generated intermediate pyrazoline into pyrazole, those cases bearing electron-donating groups exhibited obviously higher reaction activity, thus the reaction time for full conversion of intermediate pyrazoline into pyrazole was much shorter than those bearing electron-withdrawing groups. This point is easy to be understood that electron-donating groups cause electron density in pyrazoline ring very high, which favors the dehydrogenation in the presence of an oxidant.
In general, all these cases could afford good to excellent yields for the correponding 3,5-diphenyl-1H-pyrazoles, which were obtained just through immersing the resulted reaction mixtures into diluted HCl with the aid of ultrasonic irradiation and Büchner filtration. Furthermore, the reaction process involves no use of organic solvent, and only 10% excessive hydrazine was employed to achieve complete conversion of chalcone, which is much less than most traditional methods carried out in solution. All the products were well characterized by mp, IR, 1H NMR, 13C NMR and HRMS analysis. It is noteworthy that tautomerism was evident in 13C NMR experiments of the 3,5-substituted pyrazoles as reported,9,10,12 thus sometimes led to difficulty in assigning the corresponding 13C NMR signals.
In conclusion, we have successfully developed a facile, efficient and environmentally friendly method for synthesis of various 3,5-diphenyl-1H-pyrazoles using mechanochemical ball-milling technique. This one-pot protocol exhibits the advantages of short reaction time, free use of organic solvent, no separation of intermediates, low cost, simple work-up procedure, which make it a very efficient and green alternative to traditional methods for the synthesis of these kinds of compounds.
EXPERIMENTAL
NMR spectra were recorded on a Bruker AV300 or AV400 NMR spectrometer in CDCl3 or DMSO-d6. IR spectra were recorded on a Bruker VECTOR-22 instrument in KBr pellet. All melting points were determined on a XT-4 binocular microscope (Beijing Tech Instrument Co., China) and are not corrected. High resolution mass spectra (HR-MS) were recorded on a Micromass GCT-MS spectrometer with ESI mode. HPLC was conducted on an Agilent 1100 liquid chromatograph with a diode-array detector using a Zorbax Eclipse XDB-C18 (4.6 mm × 250 mm) with MeCN:H2O (4:1) as the eluent (2 mL/min), and was monitored at 254 nm. All ball-milling reactions were performed on mixer mill MM 400 (Retsch GmbH, Germany). TLC and column chromatography were performed on silica gel GF254, and silica gel H60, respectively. All other chemicals used in this study were commercially available.
Starting Materials. Chalcones were prepared according to reported method,11 and hydrazine (>99%) was purchased from Acros.
Typical Procedure for the Preparation of Products 2
Chalcone 1 (2.0 mmol), hydrazine (2.2 mmol) and a stainless ball of 7.0 mm in diameter, were introduced into a stainless jar (25 mL). The same mixture was also introduced into a second parallel jar. The two reaction vessels were sealed with screw caps, fixed on the vibration arms of a ball-milling apparatus (mixer mill MM400, Retsch GmbH, Haan, Germany) and were vibrated vigorously at a rate of 1800 rounds per minute (30 Hz) at room temperature for designated time (Time 1). Then the vessels were opened and Na2S2O8 (2.5 mmol) were added into the resulted mixtures, and the sealed vessels were continued to vibrate for designated time (Time 2). The reaction mixtures were immersed in 30 mL water with the aid of ultrasonic irradiation, and the desired solid products were collected by Büchner filtration, washed with EtOH/H2O (5 mL/5 mL) and dried in a desiccator.
3-Phenyl-5-phenyl-1H-pyrazole (2a). Mp 194–196 °C (lit.,12 197–199 °C); IR (KBr) ν 3241, 2834, 1462, 1274, 1182, 1075, 978, 750, 688 cm-1; 1H NMR (300 MHz, DMSO-d6) δ 10.40 (brs, 1H, NH), 7.84 (brs, 4H, ArH), 7.45 (brs, 4H, ArH), 7.34 (brs, 2H, ArH), 7.21 (s, 1H, CH) ; 13C NMR (75 MHz, DMSO-d6) δ 147.4, 131.3, 128.9, 128.0, 125.2, 99.8; HRMS (ESI) m/z calcd for C15H13N2 (M+H)+: 221.1079, found: 221.1068.
5-(4-Methoxyphenyl)-3-phenyl-1H-pyrazole (2b). Mp 164–166 °C (lit.,13 166–168 °C); IR (KBr) ν 3029, 2836, 1612, 1510, 1461, 1251, 1169, 1033, 976, 833, 765 cm-1; 1H NMR (300 MHz, CDCl3) δ 7.71 (d, J = 6.9 Hz, 2H, ArH), 7.62 (d, J = 8.4 Hz, 2H, ArH), 7.31–7.40 (m, 1H, ArH), 7.36 (d, J = 7.5 Hz, 2H, ArH), 6.90 (d, J = 8.4 Hz, 2H, ArH), 6.74 (s, 1H, CH), 3.82 (s, 3H, OCH3); 13C NMR (75 MHz, DMSO-d6) δ 159.0, 143.8, 143.4, 133.8, 128.7, 127.9, 127.6, 126.4, 125.0, 114.2, 98.8, 55.1; HRMS (ESI) m/z calcd for C16H15N2O (M+H)+: 251.1184, found: 251.1157.
5-(4-Chlorophenyl)-3-phenyl-1H-pyrazole (2c). Mp 212–213 °C (lit.,9 217–219 °C); IR (KBr) ν 3217, 2920, 2850, 1588, 1491, 1448, 1182, 1097, 970, 758, 688 cm-1; 1H NMR (300 MHz, DMSO-d6) δ 13.44 (brs, 1H, NH), 7.87 (brs, 2H, ArH), 7.83 (brs, 2H, ArH), 7.52 (brs, 2H, ArH), 7.47 (brs, 2H, ArH), 7.36 (brs, 1H, ArH), 7.23 (s, 1H, CH), 7.13–7.16 (m, 1H, ArH), 6.90–6.96 (m, 2H, ArH), 3.94 (s, 3H, OCH3); 13C NMR (75 MHz, DMSO-d6) δ 147.2, 142.1, 132.2, 128.8 (3C overlapped), 128.1, 128.0, 126.8, 125.1, 99.9; HRMS (ESI) m/z calcd for C15H12ClN2 (M+H)+: 255.0689, found: 255.0673.
5-(3,4-Dichlorophenyl)-3-phenyl-1H-pyrazole (2d). Mp 194–195 °C; IR (KBr) ν 3045, 2913, 2845, 1603, 1432, 1038, 956, 865, 743, 691 cm-1; 1H NMR (300 MHz, DMSO-d6) 13.51 (brs, 1H, NH), 8.07 (s, 1H, ArH), 7.77–7.83 (m, 3H, ArH), 7.68 (s, 1H, ArH), 7.38–7.47 (m, 2H, ArH), 7.31 (s, 1H, ArH), 7.20 (s, 1H, ArH); 13C NMR (75 MHz, DMSO-d6) 149.0, 143.8, 134.3, 130.9, 129.7, 129.0, 128.6, 128.3, 127.6, 126.8, 126.6, 125.1, 100.3; HRMS (ESI) m/z calcd for C15H11Cl2N2 (M+H)+: 289.0299, found: 289.0292; Anal. Calcd for C15H10Cl2N2: C, 62.30; H, 3.49; N, 9.69. Found: C, 62.46; H, 3.53; N, 9.58.
5-(4-Bromophenyl)-3-phenyl-1H-pyrazole (2e). Mp 232–233 °C (lit.,14 219–220 °C); IR (KBr) ν 3042, 2910, 2844, 1601, 1232, 1057, 955, 793, 689 cm-1; 1H NMR (300 MHz, DMSO-d6) δ 13.45 (brs, 1H, NH), 7.82 (brs, 4H, ArH), 7.64 (brs, 2H, ArH), 7.48 (brs, 2H, ArH), 7.37 (brs, 1H, ArH), 7.24 (s, 1H, CH); 13C NMR (75 MHz, DMSO-d6) δ 150.3, 143.7, 131.6, 129.0, 128.8, 128.2, 128.0, 127.1, 125.1, 120.6, 99.9; HRMS (ESI) m/z calcd for C15H12BrN2 (M+H)+: 299.0184, found: 299.0175.
5-(4-Nitrophenyl)-3-phenyl-1H-pyrazole (2f). Mp 259–261 °C (lit.,8 281 °C); IR (KBr) ν 3348, 2916, 2848, 1604, 1516, 1346, 856, 768, 698 cm-1; 1H NMR (300 MHz, DMSO-d6) δ 13.72 (brs, 1H, NH), 8.29 (d, J = 8.4 Hz, 2H, ArH), 8.12 (d, J = 8.4 Hz, 2H, ArH), 7.81 (d, J = 7.5 Hz, 2H, ArH), 7.37–7.51 (m, 3H, ArH), 7.34 (s, 1H, CH); 13C NMR (75 MHz, DMSO-d6) δ 146.9, 145.2, 142.0, 129.5, 128.9, 126.3, 125.6, 124.6, 101.5; HRMS (ESI) m/z calcd for C15H12N3O2 (M+H)+: 266.0930, found: 266.0924.
5-(3-Nitrophenyl)-3-phenyl-1H-pyrazole (2g). Mp 199–201 °C (lit.,15 204–205 °C); IR (KBr) ν 3125, 2918, 2849, 1604, 1513, 1356, 1093, 930, 813, 791 cm-1; 1H NMR (300 MHz, DMSO-d6) δ 8.68 (s, 1H, ArH), 8.31 (d, J = 7.8 Hz, 1H, ArH), 8.18–8.21 (m, 1H, ArH), 7.79–7.87 (m, 2H, ArH), 7.74–7.77 (m, 1H, ArH), 7.37–7.52 (m, 4H, ArH), 4.58 (brs, 1H, NH); 13C NMR (75 MHz, DMSO-d6) δ 148.8, 147.6, 146.4, 134.5, 131.8, 130.9, 130.5, 129.4, 128.6, 125.6, 122.6, 119.7, 101.0; HRMS (ESI) m/z calcd for C15H12N3O2 (M+H)+: 266.0930, found: 266.0928.
3-(4-Methoxyphenyl)-5-phenyl-1H-pyrazole (2h). Mp 158–160 °C (lit.,16 160–162 °C); IR (KBr) ν 3128, 2921, 2832, 1603, 1504, 1383, 1297, 1034, 931, 828 cm-1; 1H NMR (300 MHz, DMSO-d6) δ 13.04 (brs, 1H, NH), 7.83 (d, J = 7.3 Hz, 2H, ArH), 7.76 (d, J = 8.8 Hz, 2H, ArH), 7.43 (t, J = 7.5 Hz, 2H, ArH), 7.32 (t, J = 7.4 Hz, 1H, ArH), 7.02 (d, J = 8.8 Hz, 2H, ArH), 6.99 (s, 1H, CH), 3.81 (s, 3H, OCH3); 13C NMR (75 MHz, DMSO-d6) δ 158.8, 128.3, 127.2, 126.2, 124.8, 114.0, 98.5, 54.9; HRMS (ESI) m/z calcd for C16H15N2O (M+H)+: 251.1184, found: 251.1169.
3,5-Bis(4-methoxyphenyl)-1H-pyrazole (2i). Mp 156–157 °C (lit.,17 153 °C); IR (KBr) ν 3417, 2964, 2937, 2840, 1610, 1496, 1440, 1303, 1246, 1110, 1022, 962, 835, 792, 642 cm-1; 1H NMR (300 MHz, DMSO-d6) δ 12.89 (brs, 1H, NH), 7.43 (d, J = 8.8 Hz, 4H, ArH), 7.01 (d, J = 8.8 Hz, 4H, ArH), 6.88 (s, 1H, CH), 3.81 (s, 6H, OCH3); 13C NMR (75 MHz, DMSO-d6) δ 158.7, 126.1, 113.9, 97.9, 54.9; HRMS (ESI) m/z calcd for C17H17N2O2 (M+H)+: 281.1290, found: 281.1283.
5-(4-Chlorophenyl)-3-(4-methoxyphenyl)-1H-pyrazole (2j). Mp 164–166 °C; IR (KBr) ν 3230, 2919, 2838, 1666, 1601, 1504, 1255, 1169, 1095, 968, 835, 789 cm-1; 1H NMR (300 MHz, DMSO-d6) 13.27 (brs, 1H, NH), 7.82 (brs, 2H, ArH), 7.71 (brs, 2H, ArH), 7.53 (brs, 2H, ArH), 7.09 (s, 1H, CH), 7.02 (brs, 2H, ArH), 3.77 (s, 3H, OCH3); 13C NMR (75 MHz, DMSO-d6) 159.5, 141.5, 141.2, 132.0, 130.5, 129.2, 128.5, 127.2, 126.9, 114.7, 99.5, 55.6; HRMS (ESI) m/z calcd for C16H14ClN2O (M+H)+: 285.0795, found: 285.0782; Anal. Calcd for C16H13ClN2O: C, 67.49; H, 4.60; N, 9.84. Found: C, 67.46; H, 4.65; N, 9.77.
5-(4-Nitrophenyl)-3-(4-methoxyphenyl)-1H-pyrazole (2k). Mp 174–175 °C; IR (KBr) ν 3233, 2902, 2861, 1612, 1523, 1374, 1078, 930, 813, 749 cm-1; 1H NMR (300 MHz, DMSO-d6) 13.57 (brs, 1H, NH), 8.31 (d, J = 8.1 Hz, 2H, ArH), 8.13 (d, J = 8.1 Hz, 2H, ArH), 7.77 (d, J = 7.8 Hz, 2H, ArH), 7.32 (s, 1H, CH), 7.07 (d, J = 8.1 Hz, 2H, ArH), 3.82 (s, 3H, OCH3); 13C NMR (75 MHz, DMSO-d6) 159.7, 149.6, 146.8, 144.5, 140.6, 127.0, 126.2, 124.6, 122.0, 114.8, 100.6, 55.6; HRMS (ESI) m/z calcd for C16H14N3O3 (M+H)+: 296.1035, found: 296.1031; Anal. Calcd for C16H13N3O3: C, 65.08; H, 4.44; N, 14.23. Found: C, 65.15; H, 4.51; N, 14.19.
5-(3-Nitrophenyl)-3-(4-methoxyphenyl)-1H-pyrazole (2l). Mp 195–196 °C; IR (KBr) ν 3337, 2898, 2832, 1608, 1511, 1392, 1338, 1108, 938, 846, 754 cm-1; 1H NMR (300 MHz, DMSO-d6) 13.45 (brs, 1H, NH), 8.62 (s, 1H, ArH), 8.28 (d, J = 7.8 Hz, 1H, ArH), 8.15 (d, J = 8.1 Hz, 1H, ArH), 7.69–7.76 (m, 3H, ArH), 7.30 (s, 1H, CH), 7.04 (d, J = 8.7 Hz, 2H, ArH), 3.79 (s, 3H, OCH3); 13C NMR (75 MHz, DMSO-d6) 159.8, 148.8, 144.0, 142.5, 136.7, 131.7, 130.7, 127.0, 122.4, 122.1, 119.6, 114.9, 99.9, 55.6; HRMS (ESI) m/z calcd for C16H14N3O3 (M+H)+: 296.1035, found: 296.1027; Anal. Calcd for C16H13N3O3: C, 65.08; H, 4.44; N, 14.23. Found: C, 65.17; H, 4.52; N, 14.16.
5-(4-Bromophenyl)-3-(4-nitrophenyl)-1H-pyrazole (2m). Mp 243–244 °C; IR (KBr) ν 3277, 3001, 2938, 2844, 1597, 1515, 1487, 1351, 1237, 1055, 936, 742 cm-1; 1H NMR (300 MHz, DMSO-d6) 13.75 (brs, 1H, NH), 8.27–8.35 (m, 2H, ArH), 8.06–8.12 (m, 2H, ArH), 7.61–7.84 (m, 4H, ArH), 7.45 (s, 1H, CH); 13C NMR (75 MHz, DMSO-d6) 149.5, 146.4, 142.9, 139.8, 131.6, 128.1, 127.1, 125.8, 124.1, 121.5, 101.4; HRMS (ESI) m/z calcd for C15H11BrN3O2 (M+H) +: 344.0035, found: 344.0024; Anal. Calcd for C15H10BrN3O2: C, 52.35; H, 2.93; N, 12.21. Found: C, 52.39; H, 2.94; N, 12.24.
5-(4-Chlorophenyl)-3-(4-nitrophenyl)-1H-pyrazole (2n). Mp 234–236 °C; IR (KBr) ν 3431, 2916, 2850, 1602, 1516, 1491, 1344, 976, 852 cm-1; 1H NMR (300 MHz, CDCl3) 8.31 (d, J = 9.0 Hz, 2H, ArH), 7.96 (d, J = 8.7 Hz, 2H, ArH), 7.60 (d, J = 8.4 Hz, 2H, ArH), 7.46 (d, J = 8.7 Hz, 2H, ArH), 6.94 (s, 1H, CH); 13C NMR (75 MHz, DMSO-d6) 146.5, 144.3, 143.8, 143.6, 129.6, 129.1, 128.5, 126.9, 125.8, 121.4, 101.5; HRMS (ESI) m/z calcd for C15H11ClN3O2 (M+H)+: 300.0540, found: 300.0531; Anal. Calcd for C15H10ClN3O2: C, 60.11; H, 3.36; N, 14.02. Found: C, 60.20; H, 3.31; N, 13.93.
3,5-Bis(4-nitrophenyl)-1H-pyrazole (2o). Mp 257–258 °C (lit.,18 266 °C); IR (KBr) ν 3273, 2925, 2843, 1597, 1516, 1478, 1355, 1237, 1074, 915, 707 cm-1; 1H NMR (300 MHz, DMSO-d6) δ 14.07 (brs, 1H, NH), 8.30–8.39 (m, 4H, ArH), 8.07–8.16 (m, 4H, ArH), 7.69 (s, 1H, CH); 13C NMR (75 MHz, DMSO-d6) δ 147.0, 139.1, 129.5, 124.6, 101.5; HRMS (ESI) m/z calcd for C15H11N4O4 (M+H)+: 311.0780, found: 311.0773.
ACKNOWLEDGEMENTS
We are grateful for the financial support from National Natural Science Foundation of China (21242013, 20902002) and Anhui Provincial Natural Science Foundation (No. 10040606Q04).
References
1. B. Cottineau, P. Toto, C. Marot, C. Marot, A. Pipaud, and J. Chenault, Bioorg. Med. Chem. Lett., 2002, 12, 2105; CrossRef R. R. Ranatunge, D. S. Garvey, D. R. Janero, L. G. Letts, A. M. Martino, M. G. Murty, S. K. Richardson, D. V. Young, and I. S. Zemetseva, Bioorg. Med. Chem., 2004, 12, 1357; CrossRef F. Manna, F. Chimenti, R. Fioravanti, A. Bolasco, D. Secci, P. Chimenti, C. Ferlini, and G. Scambia, Bioorg. Med. Chem. Lett., 2005, 15, 4632; CrossRef H. Khalilullah, S. Khan, M. J. Ahsan, and B. Ahmed, Bioorg. Med. Chem. Lett., 2011, 21, 7251. CrossRef
2. J. Elguero, 'Comprehensive Heterocyclic Chemistry,' ed. by A. R. Katritzky, Pergamon, Oxford, 1996; J.-Y. Zhang, B.-F. Shen, and A.-N. Lin, Trends Pharmacol. Sci., 2007, 28, 286; CrossRef H. Biere, I. Bottcher, and J. F. Kapp, Arch. Pharm., 1983, 316, 588; CrossRef F. Wu, L. Hao, and Z.-P. Zhan, Heterocycles, 2013, 87, 657. CrossRef
3. C. B. Vicentini, D. Mares, A. Tartari, M. Manfrini, and G. Forlani, J. Agric. Food Chem., 2004, 52, 1898; CrossRef L. Miao, C.-L. Liu, J.-C. Yang, J.-B. Zhang, Z.-N. Li, Z. Hong, and Z.-M. Li, J. Agric. Food Chem., 2010, 58, 2664. CrossRef
4. L. M. Cole, R. A. Nicholson, and J. E. Casida, Pestic. Biochem. Physiol., 1993, 46, 47. CrossRef
5. For some reviews, see: E. Boldyreva, Chem. Soc. Rev., 2013, 42, 7719; CrossRef S.-E. Zhu, F. Li, and G.-W. Wang, Chem. Soc. Rev., 2013, 42, 7535; CrossRef G.-W. Wang, Chem. Soc. Rev., 2013, 42, 7668; CrossRef J. Huot, D. B. Ravnsbæk, J. Zhang, F. Cuevas, M. Latroche, and T. R. Jensen, Prog. Mater. Sci., 2013, 58, 30; CrossRef R. B. N. Baig and R. S.Varma, Chem. Soc. Rev., 2012, 41, 1559; CrossRef S. L. James, C. J. Adams, C. Bolm, D. Braga, P. Collier, T. Friščić, F. Grepioni, K. D. M. Harris, G. Hyett, W. Jones, A. Krebs, J. Mack, L. Maini, A. G. Orpen, I. P. Parkin, W. C. Shearouse, J. W. Steed, and D. C. Waddell, Chem. Soc. Rev., 2012, 41, 413; CrossRef A. Stolle, T. Szuppa, S. E. S. Leonhardt, and B. Ondruschka, Chem. Soc. Rev., 2011, 40, 2317; CrossRef G. Kaupp, CrystEngComm, 2009, 11, 388; CrossRef B. Rodríguez, A. Bruckmann, T. Rantanen, and C. Bolm, Adv. Synth. Catal., 2007, 349, 2213. CrossRef
6. For most recently selected examples, see: G.-P. Fan, Z. Liu, and G.-W. Wang, Green Chem., 2013, 15, 1659; CrossRef C. B. Aakeröy and A. S. Sinha, RSC Adv., 2013, 3, 8168; CrossRef M. Jorres, S. Mersmann, G. Raabe, and C. Bolm, Green Chem., 2013, 15, 612; CrossRef J.-B. Yu, Z.-H. Li, K.-Y. Jia, Z.-J. Jiang, M.-L. Liu, and W.-K. Su, Tetrahedron Lett., 2013, 54, 2006; CrossRef N. Mukherjee, S. Ahammed, S. Bhadra, and B. C. Ranu, Green Chem., 2013, 15, 389; CrossRef I. Y. Jeon, H. J. Choi, S. M. Jung, J. M. Seo, M. J. Kim, L.-M. Dai, and J. B. Baek, J. Am. Chem. Soc., 2013, 135, 1386; CrossRef J.-B. Yu, Z.-H. Li, and W.-K. Su, Synth. Commun., 2013, 43, 361; CrossRef M. Pascu, A. Ruggi, R. Scopelliti, and K. Severin, Chem. Commun., 2013, 49, 45; CrossRef Z. Liu, G.-P. Fan, and G.-W. Wang, Chem. Commun., 2012, 48, 11665; CrossRef G.-W. Wang and J. Gao, Green Chem., 2012, 14, 1125; CrossRef R. Sharma, A. K. Pandey, and P. M. S. Chauhan, Synlett, 2012, 23, 2209. CrossRef
7. Z. Zhang, H.-H. Wu, and Y.-J. Tan, RSC Adv., 2013, 3, 16940; CrossRef Z. Zhang, Z.-W. Peng, M.-F. Hao, and J.-G. Gao, Synlett, 2010, 21, 2895; CrossRef Z. Zhang, J. Gao, J.-J. Xia, and G.-W. Wang, Org. Biomol. Chem., 2005, 3, 1617; CrossRef Z. Zhang, Y.-W. Dong, G.-W. Wang, and K. Komatsu, Synlett, 2004, 61; CrossRef Z. Zhang, Y.-W. Dong, G.-W. Wang, and K. Komatsu, Chem. Lett., 2004, 33, 168; CrossRef Z. Zhang, G.-W. Wang, C.-B. Miao, Y.-W. Dong, and Y.-B. Shen, Chem. Commun., 2004, 1832. CrossRef
8. A. Y. Shawa, H.-H. Liau, P.-J. Lu, C.-N. Yang, C.-H. Lee, J.-Y. Chen, Z.-G. Xu, and G. Flynn, Bioorg. Med. Chem., 2010, 18, 3270. CrossRef
9. F. Chimenti, R. Fioravanti, A. Bolasco, F. Manna, P. Chimenti, D. Secci, O. Befani, P. Turini, F. Ortuso, and S. Alcaro, J. Med. Chem., 2007, 50, 425. CrossRef
10. Some selected examples for tautomerism in pyrazoles, see: D. S. Wofford, D. M. Forkey, and J. G. Russell, J. Org. Chem., 1982, 47, 5132; CrossRef M. T. Chenon, C. Coupry, D. M. Grant, and R. J. Pugmire, J. Org. Chem., 1977, 42, 659; CrossRef J. Elguero, C. Marzin, and J. D. Roberts, J. Org. Chem., 1974, 39, 357. CrossRef
11. Z. Zhang, Y.-W. Dong, and G.-W. Wang, Chem. Lett., 2003, 32, 966. CrossRef
12. P. Liu, Q.-Q. Xu, C. Dong, X.-S. Lei, and G.-Q. Lin, Synlett, 2012, 23, 2087. CrossRef
13. J. P. Stonehouse, D. S. Chekmarev, N. V. Ivanova, S. Lang, G. Pairaudeau, N. Smith, M. J. Stocks, S. I. Sviridov, and L. M. Utkina, Synlett, 2008, 19, 100. CrossRef
14. Z.-J. Ren, W.-G. Cao, J. Chen, Y. Wang, and W.-Y. Ding, J. Heterocycl. Chem., 2006, 43, 495. CrossRef
15. G. Cocconcelli, E. Diodato, A. Caricasole, G. Gaviraghi, E. Genesio, C. Ghiron, L. Magnoni, E. Pecchioli, P. V. Plazzi, and G. C. Terstappen, Bioorg. Med. Chem., 2008, 16, 2043. CrossRef
16. V. K. Aggarwal, J. D. Vicente, and R. V. Bonnert, J. Org. Chem., 2003, 68, 5381. CrossRef
17. B. A. Bhat, S. C. Puri, M. A. Qurishi, K. L. Dhar, and G. N. Qazi, Synth. Commun., 2005, 35, 1135. CrossRef
18. M. Lipp, F. Dallacker, and S. Munnes, Justus Liebigs Ann. Chem., 1958, 618, 110. CrossRef