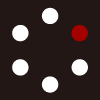
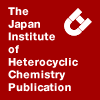
HETEROCYCLES
An International Journal for Reviews and Communications in Heterocyclic ChemistryWeb Edition ISSN: 1881-0942
Published online by The Japan Institute of Heterocyclic Chemistry
e-Journal
Full Text HTML
Received, 30th October, 2013, Accepted, 6th December, 2013, Published online, 6th January, 2014.
DOI: 10.3987/COM-13-12876
■ Synthesis and Photochemical Properties of 2,4,6-Triaryl-4H-1,4-oxazines
Hongbo Tan, Hongxing Xin, and Hong Yan*
College of Life Sciences & Bioengineering, Beijing University of Technology, Pingleyuan 100, Chaoyang District, Beijing 100124, China
Abstract
A series of 2,4,6-triaryl-4H-1,4-oxazines was synthesized, and their photochemical properties were studied. The 2,4,6-triaryl-4H-1,4-oxazines underwent photoreaction to N-(1-methoxy-2-oxo-2-arylethyl)-N-arylformamides determined by 1H NMR, 13C NMR, MS, and single crystal X-ray diffraction analyses.INTRODUCTION
Heterocycles possess intrinsic reactivity that enables a wide variety of versatile and productive transformations. Because of their ubiquitous presence in natural products and drugs, the development of novel and efficient preparations of these structures remains a fundamental goal of organic synthesis. Although the importance of 1,4-oxazines has been extensively documented, primarily as a result of pharmaceutical and chromogenic research,1-8 there is only one known synthesis of 2,4,6-triphenyl-4H-1,4-oxazine.9 In connection with our research on the synthesis of heterocycles containing nitrogen or oxygenand particular interest in six-membered rings,10-12 we intended to prepare a series of oxazines and broaden the scope of the reported method. A series of the desired 2,4,6-triaryl-4H-1,4-oxazines was synthesized by the reaction of N,N-bis(phenacyl)anilines with an excess of POCl3 in pyridine (Scheme 1). Because we are interested in the photochemical properties of heterocycles containing nitrogen or oxygen,13-16 the photochemical properties of the triaryl-4H-1,4-oxazines were investigated. The 2,4,6-triaryl-4H-1,4-oxazine was unstable and photoreacted to the N-(1-methoxy-2-oxo-2-arylethyl)-N-arylformamide during UV irradiation in MeOH (Scheme 2).
RESULTS AND DISCUSSION
Synthesis of 2,4,6-triaryl-4H-1,4-oxazines (5-8)
The synthesis of the 2,4,6-triaryl-4H-1,4-oxazines was carried out according to the literature,9 and 2,4,6-triphenyl-4H-1,4-oxazine (5a) was obtained as the only product by the reaction of N,N-bis(phenacyl)aniline (1a) with an excess of POCl3 in pyridine. When substituents (R1 and R2) were introduced on the phenyl rings, the breadth of the method was shown by the formation of various 4H-1,4-oxazines (Table 1). The yields of the triaryl-4H-1,4-oxazines varied predictably with the identity of the substituents (R1 and R2), and the best yields of 7c were obtained when R1 and R2 were both 4-Cl (74%). For example, when R1 is H, the yield of 5c (R2 = 4-Cl) is higher than that of 5a and 5b. When R2 is H, the yield of 7a (R1 = 4-Cl) was higher than that of 5a or 6a. These results show that an electron-withdrawing group at R1 and R2 is preferred. It is probably because the electron-withdrawing group is beneficial for the large conjugated structure and decreases the electronic density throughout. When R1 is 4-OMe, a trace of 8a-c was observed by TLC during the reaction and disappeared within a few minutes. The phenyl rings with strong electron-donating groups (R1 = 4-OMe) may cause the 2,4,6-triaryl-4H-1,4-oxazines to be unstable.
Photochemical study of 2,4,6-triaryl-4H-1,4-oxazines (5-8)
In this experiment, the 2,4,6-triaryl-4H-1,4-oxazines were found to be stable in the solid state and unstable in solution. Due to their common features, 5a was chosen as a representative compound to investigate their stability in a variety of conventional solvents (such as MeOH, benzene, hexane, dichloromethane, EtOAc, acetone, tetrahydrofuran, acetonitrile, pyridine, and so on). The results showed that 5a was unstable in solvents, except for MeOH, acetone and pyridine. And 5a was found more soluble in MeOH than acetone and pyridine. So, MeOH was chosen as the solvent in the study of the photochemical properties of the title compounds.
The UV-Vis absorption spectra of 5 and 7 in MeOH at a concentration of 1×10−5 M are shown in Table 2 and Figure 1. Several absorption peaks were observed in the linear absorption spectra for all of the molecules in the wavelength ranging from 220 to 600 nm, while almost no linear absorption was observed beyond 600 nm. The spectral shapes are similar because that these compounds have the same stem nuclei of the 2,4,6-triaryl-4H-1,4-oxazine structure. The maximum absorptions range from 236 to 242 nm, 346 to 356 nm, and 436 to 456 nm and are similar to the UV-Vis absorption spectra of 5a.9
The results in Table 2 and Figure 1 show that the red shifts of the absorption peaks are observed solely because of the introduction of auxochrome groups on the phenyl ring. For example, when R1 is the same, the wavelength maximum of 5b (R1 = H, R2 = p-Me) is 444 nm, while the wavelength maximum of 7b (R1 = p-Cl, R2 = p-Me) is 456 nm, which is 12 nm longer than that of 5b. According to the data above, obviously that when R1 is auxochrome group, such as -Cl, the UV-Vis absorption spectra of 2,4,6-triaryl-4H-1,4-oxazines show red shifts of the absorption peaks.
The photostability was investigated by the changes of UV-Vis absorption spectra irradiated with UV lights of 450 W medium pressure mercury lamp in MeOH at a concentration of 10−5 M (Figure2). After irradiation for 1 h, all three wavelength maxima of 5a and 7a (236-244 nm, 346-354 nm, and 436-454 nm) decreased dramatically from those taken before the irradiation. In particular, the wavelength maximum at 346-354 nm (the absorbance of the phenyl rings in the 2- and 6-position) decreased in magnitude, and the 436-454 nm peaks (the absorbance of the conjugated ring) disappeared. The changes in the UV-Vis absorption spectra may be caused by fragmenting the conjugated structures during irradiation.
To elucidate the photochemical reaction of 2,4,6-triaryl-4H-1,4-oxazines, 5a was used as reactant in a model reaction in MeOH. 5a was irradiated by a 450 W medium pressure mercury lamp, and the progress of the reaction was monitored by TLC. After irradiation for approximately 1 h, 9a and benzoic acid 11 were obtained in the yields of 12% and 10% (Scheme 2). A similar transformation of 5a also occurred by irradiation with sunlight for about 3 days, and the yields of 9a and 11 were almost the same as those obtained from irradiation with the mercury lamp. The structure of 9a was N-(1-methoxy-2-oxo-2-phenylethyl)-N-phenylformamide confirmed by 1H NMR, 13C NMR, and MS. Due to their structural similarity, compounds 5b and 7a were irradiated under the same conditions used for 5a in MeOH. The results indicate that the 2,4,6-triaryl-4H-1,4-oxazines undergo a photoreaction to the N-(1-methoxy-2-oxo-2-arylethyl)-N-arylformamides (9, 10) and corresponding benzoic acids (11, 12). The substituents on the benzene rings have minor effects on the yields of the photoreaction, e.g., the yields of 9b and 10a, which have electron-donating and electron-withdrawing groups, respectively, are similar.
The structures of 9a-b, 10a were confirmed by 1H NMR, 13C NMR, and MS. Compound 10a will be used as a representative example for discussing the details of the structures. In the1H NMR spectra of 10a, there was a signal from the aldehyde group at δ 8.50, signals corresponding to the 9 protons on the two benzene rings in the range of δ 6.99-7.76, a signal from the methine group at δ 6.92, a signal from the methoxy groupat δ 3.67. In the 13C NMR spectra of 10a there were only 12 signals due to the symmetry of the benzene ring. The two carbonyl carbons resided at δ 190.8 and 163.4 respectively, the 8 signals of the benzene rings appeared at δ 82.8-140.4, and the 2 signals from the the two saturated carbons were found at δ 56.6 and 76.7. MS (ESI) m/z (%) = 325.7 [M+Na]+. Single crystal X-ray diffraction of 10a (Deposition number CCDC-735378) further proves that the photoreaction product is N-(1-methoxy-2-oxo-2-phenylethyl)-N-phenylformamide (Figure 3).
A mechanism of the formation of 9a is speculated via the photoaddition by the MeOH and photooxygen by the singlet oxygenshown in Scheme 3. The 5a is added by MeOH molecule to give M1 via anti-Markownikoff addition. Actually, when other alcohols were used in this reaction, 5a could not give the corresponding photoadditional products. The reaction may proceed via photoinduced electron-transfer (PET) between photoexcited 5a with some kind of electron acceptor (O2 is possible candidate) to give radical cation of 5a, then the radical cation of 5a attacks on MeOH, then deprotonation, one-electron reduction of the resulting radical (maybe from O2•-) to give anion, and protonation, according to Mizuno.17 The conversion of M1 to M2 is speculated to be associated with the singlet oxygen which probably comes from air in solution by absorbing UV (wavelengths <320 nm).18-22 It was proved by the additions of air and N2. The formation of 9a was accelerated with the addition of air and abated under nitrogen atmosphere. The presence of singlet oxygen was proved by the addition of quencher or photosensitizer which can capture the singlet oxygen or convert the oxygen molecule to singlet oxygen. The quencher was 1,4-diazabicyclo[2.2.2]octane which slowed down the formation of 9a and 11 dramatically. The photosensitizer was a rubrene which speeded up the reaction considerably. The 1,2-dioxetane M2 is the precursor of the C-C bond cleavage product M3 and an intramolecular electron-transfer mechanism is proposed for the cleavage of these peroxides. The M2 is decomposed by a stepwise process involving homolysis of the peroxide bond to form a diradical with subsequent C-C bond cleavage product M3.23 The N-formyl group stabilizes M3 probably because the electron-withdrawing effect of the formyl prevents the lone-pair electron of the nitrogen from participating in breaking the C-O bond of M3.24 In Scheme 3, there is another possibility that reverses the order of photoaddition of MeOH and singlet oxygen. Namely, route of addition of singlet oxygen occurs first, then MeOH photochemically adds. The accurate and reasonable explanation of the mechanism of the photoreaction of 2,4,6-triaryl-4H-1,4-oxazines will be proposed by the further studies.
EXPERIMENTAL
Materials and Methods
All chemicals were purchased from commercial sources and used without further purification. Thin-layer chromatography (TLC) was conducted on silica gel 60 F254 plates (Merck KGaA). Melting points were determined on a XT-5A digital melting point apparatus and are uncorrected. 1H NMR spectra and 13C NMR spectra were recorded on a Bruker Avance 400 spectrometer at 400 MHz and 100 MHz using CDCl3 or acetone-d6 as the solvent and tetramethylsilane (TMS) as the internal standard. High-resolution mass spectral (HRMS) analyses were carried out using a VG 70SE mass spectrometer (Manchester, UK), which was operated in electron impact or electrospray ionization mode. UV-Vis absorption spectra were measured using a U-4100 UV/VIS spectrophotometer (Hitachi). Elemental analysis was performed on a vario EL elemental analyzer. Irradiation for both the photostability studies and photochemical reactions was conducted using an Osram HBO 450W/2 medium pressure mercury lamp. To mimic UV radiation, a 10-mm-thick Pyrex filter was placedbetween the lamp and the sample that was irradiated. The filter allows irradiation only by wavelengths greater than 290 nm. The samples were irradiated while in quartz cuvettes.
General procedure for the synthesis of N,N-bis(phenacyl)anilines
A mixture of phenacyl bromide (12 mmol), Na2CO3 (12 mmol), and aniline (6.0 mmol) was stirred under reflux at 110 °C. The progress of the reaction was monitored by TLC. After the indicated reaction time, the solid products 1-4 were recrystallized from EtOH.
N,N-Bis(phenacyl)aniline (1a). Yellow needles, yield 65%; mp 196.2-197.4 °C (mp 198 °C11); 1H NMR spectra (400 MHz, CDCl3, 25 °C): δ 8.03 (d, J = 7.2 Hz, 4H, Ar-H), 7.62 (t, J = 7.6 Hz, 2H, Ar-H), 7.51 (t, J = 7.6 Hz, 4H, Ar-H), 7.16 (t, J = 7.6 Hz, 2H, Ar-H), 6.73 (t, J = 7.6 Hz, 1H, Ar-H), 6.55 (d, J = 8.4 Hz, 2H, Ar-H), 4.95 (s, 4H, N-CH2).
N,N-Bis(phenacyl)-4-toluidine (1b). Yellow needles, yield 73%; mp 153.2-154.1 °C (mp 158 °C11); 1H NMR spectra (400 MHz, CDCl3, 25 °C): δ 8.01 (d, J = 7.2 Hz, 4H, Ar-H), 7.61 (t, J = 7.6 Hz, 2H, Ar-H), 7.49 (t, J = 7.6 Hz, 4H, Ar-H), 6.75 (d, J = 8.8 Hz, 2H, Ar-H), 6.57 (d, J = 8.8 Hz, 2H, Ar-H), 4.95 (s, 4H, N-CH2), 2.24 (s, 3H, CH3).
N,N-Bis(phenacyl)-4-chloroaniline (1c). Yellow needles, yield 55%; mp 158.3-160.2 °C11; 1H NMR spectra (400 MHz, CDCl3, 25 °C): δ 8.02 (d, J = 7.6 Hz, 4H, Ar-H), 7.67 (t, J = 7.2 Hz, 2H, Ar-H), 7.56 (t, J = 7.6 Hz, 4H, Ar-H), 7.09 (d, J = 8.8 Hz, 2H, Ar-H), 6.50 (d, J = 8.8 Hz, 2H, Ar-H), 5.03 (s, 4H, N-CH2).
N,N-Bis(4-methylphenacyl)aniline (2a). Yellow needles, yield 62%; mp 103.7-104.5 °C (mp 103 °C11); 1H NMR spectra (400 MHz, CDCl3, 25 °C): δ 7.93 (d, J = 8.0 Hz, 4H, Ar-H), 7.30 (d, J = 8.0 Hz, 4H, Ar-H), 7.14 (t, J = 7.6 Hz, 2H, Ar-H), 6.73 (d, J = 7.2 Hz, 1H, Ar-H), 6.52 (d, J = 8.4 Hz, 2H, Ar-H), 4.92 (s, 4H, N-CH2), 2.43 (s, 6H, CH3).
N,N-Bis(4-methylphenacyl)-4-toluidine (2b). Yellow needles, yield 70%; mp 142.7-144.3 °C (mp 128°C11); 1H NMR spectra (400 MHz, CDCl3, 25 °C): δ 7.91 (d, J = 8.0 Hz, 4H, Ar-H), 7.29 (d, J = 8.0 Hz, 4H, Ar-H), 6.95 (d, J = 8.0, 2H, Ar-H), 6.47 (d, J = 8.4 Hz, 2H, Ar-H), 4.90 (s, 4H, N-CH2), 2.43 (s, 6H, CH3), 2.20 (s, 3H, CH3).
N,N-Bis(4-methylphenacyl)-4-chloroaniline (2c). Yellow needles, yield 54%; mp 178.5-179.3 °C11; 1H NMR spectra (400 MHz, CDCl3, 25 °C): δ 7.90 (d, J = 8.0, 4H, Ar-H), 7.29 (d, J = 8.0 Hz, 4H, Ar-H), 7.08 (d, J = 9.2 Hz, 2H, Ar-H), 6.43 (d, J = 9.2 Hz, 2H, Ar-H), 4.89 (s, 4H, N-CH2), 2.43 (s, 6H, CH3).
N,N-Bis(4-chlorophenacetyl)aniline (3a). Yellow needles, yield 76%; mp 108.1-109.8 °C (mp 110 °C11); 1H NMR spectra (400 MHz, CDCl3, 25 °C): δ 7.96 (d, J = 8.4 Hz, 4H, Ar-H), 7.48 (d, J = 8.4 Hz, 4H, Ar-H), 7.17 (t, J = 8.0 Hz, 2H, Ar-H), 6.76 (t, J = 7.2 Hz, 1H, Ar-H), 6.52 (d, J = 8.0 Hz, 2H, Ar-H), 4.90 (s, 4H, N-CH2).
N,N-Bis(4-chlorophenacyl)-4-toluidine (3b). Yellow needles, yield 80%; mp 137.3-138.1 °C (mp 140.0 °C11); 1H NMR spectra (400 MHz, CDCl3, 25 °C): δ 7.93 (d, J = 7.6 Hz, 4H, Ar-H), 7.33 (d, J = 7.6 Hz, 4H, Ar-H), 6.97 (d, J = 8.4 Hz, 2H, Ar-H), 6.49 (d, J = 8.4 Hz, 2H, Ar-H), 4.88 (s, 4H, N-CH2), 2.23 (s, 3H, CH3).
N,N-Bis(4-chlorophenacyl)-4-chloroaniline (3c). Yellow needles, yield 67%; mp 181.5-182.3 °C11; 1H NMR spectra (400 MHz, CDCl3, 25 °C): δ 7.94 (d, J = 8.4, 4H, Ar-H), 7.49 (d, J = 8.4 Hz, 4H, Ar-H), 7.11 (d, J = 8.4 Hz, 2H, Ar-H), 6.43 (d, J = 8.8 Hz, 2H, Ar-H), 4.87 (s, 4H, N-CH2).
N,N-Bis(4-methoxyphenacyl)aniline (4a). Yellow needles, yield 60%; mp 150.9-151.2 °C (mp 152.0 °C11); 1H NMR spectra (400 MHz, CDCl3, 25 °C): δ 8.01 (d, J = 8.8 Hz, 4H, Ar-H), 7.14 (t, J = 8.0 Hz, 2H, Ar-H), 6.97 (d, J = 8.8 Hz, 4H, Ar-H), 6.71 (t, J = 7.6 Hz, 1H, Ar-H), 6.53 (d, J = 8.4 Hz, 2H, Ar-H), 4.89 (s, 4H, N-CH2), 3.88 (s, 6H, OCH3).
N,N-Bis(4-methoxyphenacyl)-4-toluidine (4b). Yellow needles, yield 66%; mp 169.2-171.1 °C (mp 170.0 °C11); 1H NMR spectra (400 MHz, CDCl3, 25 °C): δ 8.21 (d, J = 8.8 Hz, 4H, Ar-H), 7.96 (d, J = 8.8 Hz, 4H, Ar-H), 6.94 (d, J = 8.0 Hz, 2H, Ar-H), 6.41 (d, J = 8.4 Hz, 2H, Ar-H), 4.80 (s, 4H, N-CH2), 3.90 (s, 6H, OCH3), 2.20 (s, 3H, CH3).
N,N-Bis(4-methoxyphenacyl)-4-chloroaniline (4c). Yellow needles, yield 49%; mp 168.7-169.3 °C11; 1H NMR spectra (400 MHz, CDCl3, 25 °C): δ 8.06 (d, J = 8.4 Hz, 4H, Ar-H), 7.31 (d, J = 8.4 Hz, 4H, Ar-H), 7.03 (d, J = 8.4 Hz, 2H, Ar-H), 6.50-6.52 (m, 2H, Ar-H), 4.94 (s, 4H, N-CH2), 3.63 (s, 6H, OCH3).
General procedure for the synthesis of 2,4,6-triaryl-4H-1,4-oxazines
A mixture of N,N-bis(phenacyl)anilines (5 mmol) and 0.87 mL (9.4 mmol) of POCl3 in 30 mL of pyridine (dried over CaH2) was heated with occasional swirling at 100 °C for 45 min. The deep red solution was poured onto 50 mL of crushed ice, and the resulting solid was filtered. The crude products were washed twice with a small amount of MeOH to purify the products.
2,4,6-Triphenyl-4H-1,4-oxazine (5a). Orange red powder, yield 50%; mp 181.6-182.4 °C (mp 183.0-185.0 °C9); 1H NMR spectra (400 MHz, acetone-d6, 25 °C): δ 7.55 (d, J = 6.8 Hz, 4H, Ar-H), 7.29-7.43 (m, 10H, Ar-H), 7.03 (t, J = 6.8 Hz, H, Ar-H), 6.94 (s, 2H, N-CH=); 13C NMR spectra (100 MHz, 25 °C, acetone-d6): δ 142.3, 138.7, 132.8, 129.7, 128.4, 127.5, 122.8, 120.9, 113.8, 109.3.
2,6-Diphenyl-4-(4-tolyl)-4H-1,4-oxazine (5b). Orange red powder, yield 53%; mp 115.7-117.2 °C; 1H NMR spectra (400 MHz, acetone-d6, 25 °C): δ 7.75 (d, J = 7.6 Hz, 4H, Ar-H), 7.40 (t, J = 7.6 Hz, 4H, Ar-H), 7.29 (t, J = 7.6 Hz, 2H, Ar-H), 7.18 (d, J = 8.4 Hz, 4H, Ar-H), 6.98 (s, 2H, N-CH=), 2.28 (s, 3H, Ar-CH3); 13C NMR spectra (100 MHz, acetone-d6, 25 °C): δ 140.0, 137.8, 132.9, 130.0, 129.9, 128.4, 127.1, 122.6, 113.9, 110.0, 19.7; HRMS(ESI): (m/z) calcd for C23H19NO: 325.1467 [M]+; found 325.1453.
2,6-Diphenyl-4-(4-chlorophenyl)-4H-1,4-oxazine (5c). Orange red powder, yield 63%; mp 98.6-99.5 °C; 1H NMR spectra (400 MHz, acetone-d6, 25 °C): δ 7.77 (d, J = 7.6 Hz, 4H, Ar-H), 7.29-7.43 (m, 10H, Ar-H), 7.03 (s, 2H, N-CH=); 13C NMR spectra (100 MHz, acetone-d6, 25 °C): δ 140.7, 138.3, 132.6, 129.2, 128.4, 127.5, 124.6, 122.9, 114.9, 109.0; HRMS(ESI): (m/z) calcd for C22H16ClNO: 345.0830 [M]+; found 345.0812.
2,6-Bis(4-tolyl)-4-phenyl-4H-1,4-oxazine (6a). Orange red powder, yield 53%; mp 113.5-114.2 °C; 1H NMR spectra (400 MHz, acetone-d6, 25 °C): δ 7.64 (d, J = 7.6 Hz, 4H, Ar-H), 6.92-7.35 (m, 9H, Ar-H), 6.91 (s, 2H, N-CH=), 2.35 (s, 6H, Ar-CH3); 13C NMR spectra (100 MHz, acetone-d6, 25 °C): δ 142.0, 138.5, 137.0, 130.1, 129.4, 129.0, 122.8, 120.3, 113.4, 108.6, 20.4; HRMS(ESI): (m/z) calcd for C24H21NO: 339.1623 [M]+; found 339.1602.
2,4,6-Tri(4-tolyl)-4H-1,4-oxazine (6b). Orange red powder, yield 57%; mp 133.6-134.2 °C; 1H NMR spectra (400 MHz, acetone-d6, 25 °C): δ 7.13-7.60 (m, 12H, Ar-H), 6.87 (s, 2H, N-CH=), 2.27 (s, 6H, Ar-CH3), 2.04 (s, 3H, Ar-CH3); 13C NMR spectra (100 MHz, acetone-d6, 25 °C): δ 140.0, 137.8, 132.9, 130.0, 129.9, 128.4, 127.1, 122.6, 113.9, 110.0, 19.7; HRMS(ESI): (m/z) calcd for C25H23NO: 353.1736 [M]+; found 353.1702.
2,6-Bis(4-tolyl)-4-(4-chlorophenyl)-4H-1,4-oxazine (6c). Orange red powder, yield 63%; mp 98.6-99.9 °C; 1H NMR spectra (400 MHz, acetone-d6, 25 °C): δ 6.90-7.47 (m, 12H, Ar-H), 6.44 (s, 2H, N-CH=), 2.38 (s, 6H, Ar-CH3); 13C NMR spectra (100 MHz, acetone-d6, 25 °C): δ 140.7, 138.5, 129.4, 129.3, 129.1, 124.2, 122.9, 122.7, 114.7, 109.1, 20.2; HRMS(ESI): (m/z) calcd for C24H20ClNO: 373.1140 [M]+; found 373.1126.
2,6-Bis(4-chlorophenyl)-4-phenyl-4H-1,4-oxazine (7a). Orange red powder, yield 60%; mp 132.5-133.6 °C; 1H NMR spectra (400 MHz, acetone-d6, 25 °C): δ 7.78 (d, J = 8.0 Hz, 4H, Ar-H), 7.30-7.54 (m, 9H, Ar-H), 7.05 (s, 2H, N-CH=); 13C NMR spectra (100 M Hz, acetone-d6, 25 °C): δ 140.6, 138.8, 132.6, 129.2, 128.4, 127.5, 124.6, 122.9, 114.9, 109.0; HRMS(ESI): (m/z) calcd for C22H15Cl2NO: 379.0531 [M]+; found 379.0516.
2,6-Bis(4-chlorophenyl)-4-(4-tolyl)-4H-1,4-oxazine (7b). Orange red powder, yield 65%; mp 153.9-154.7 °C; 1H NMR spectra (400 MHz, acetone-d6, 25 °C): δ 7.74 (d, J = 8.8 Hz, 4H, Ar-H), 7.39-7.42 (m, 4H, Ar-H), 7.21 (d, J = 8.4 Hz, 2H, Ar-H), 7.16 (d, J = 8.4 Hz, 4H, Ar-H), 6.97 (s, 2H, N-CH=), 2.29 (s, 3H, Ar-CH3); 13C NMR spectra (100 MHz, acetone-d6, 25 °C): δ 140.0, 138.8, 132.3, 130.0, 128.4, 128.3, 127.6, 124.5, 113.8, 110.1, 19.6; HRMS(ESI): (m/z) calcd for C23H17Cl2NO: 393.0687 [M]+; found 393.0689.
2,4,6-Tri(4-chlorophenyl-4H-1,4-oxazine (7c). Orange red powder, yield 74%; mp 145.3-146.7 °C; 1H NMR spectra (400 MHz, acetone-d6, 25 °C): δ 7.65 (d, J = 6.8 Hz, 4H, Ar-H), 7.08-7.45 (m, 8H, Ar-H), 6.95 (s, 2H, N-CH=); 13C NMR spectra (100 MHz, acetone-d6, 25 °C): δ 140.6, 138.7, 132.5, 129.2, 128.2, 127.5, 124.6, 122.8, 114.7, 109.0; HRMS(ESI): (m/z) calcd for C22H14Cl3NO: 413.0112 [M]+; found 413.0087.
General procedure for the photoreaction of 2,4,6-triaryl-4H-1,4-oxazines
The 2,4,6-triaryl-4H-1,4-oxazines (0.32 mmol) were dissolved in MeOH (200 mL) and poured into the photolysis unit. Photo-irradiations were performed using a medium pressure mercury lamp (450 W), and a 10-mm-thick Pyrex filter was placed between the lamp and sample. The reaction was monitored by TLC. After completion, the solvent was removed under reduced pressure, and the residue was purified by chromatography on silica gel using a mixed solvent of petroleum ether and EtOAc (20:3 v/v) to provide 9, 10 and the corresponding benzoic acids 11 and 12.
N-(1-Methoxy-2-oxo-2-phenylethyl)-N-phenylformamide (9a). Pale yellow solid, yield 12%; mp 127.2-128.7 °C; 1H NMR spectra (400 MHz, CDCl3, 25 °C): δ 8.49 (s, 1H, CHO), 6.98-7.80 (m, 10H, Ar-H), 6.97 (s, 1H, CH), 3.69 (s, 3H, OCH3); 13C NMR spectra (100 MHz, CDCl3, 25 °C): δ 191.9, 163.5, 137.0, 134.5, 133.8, 129.3, 128.7, 128.2, 128.1, 126.3, 82.8, 56.6; MS(ESI) m/z (%) = 291.7 [M+Na]+. Anal. Calcd (%) for C16H15NO3: C 71.36, H 5.61, N 5.20. Found: C 71.35, H 5.58, N 5.22.
N-(1-Methoxy-2-oxo-2-phenylethyl)-N-(4-tolyl)formamide (9b). Pale yellow solid, yield 15%; mp 135.5-136.6 °C ; 1H NMR spectra (400 MHz, CDCl3, 25 °C): δ 8.47 (s, 1H, CHO), 7.43-7.85 (m, 5H, Ar-H), 7.06 (d, J = 8.0 Hz, 2H, Ar-H), 6.98 (s, 1H, CH), 6.86 (d, J = 8.0 Hz, 2H, Ar-H), 3.69 (s, 3H, OCH3), 2.31 (s, 3H, CH3); 13C NMR spectra (100 MHz, CDCl3, 25 °C): δ 191.9, 163.5, 138.2, 134.4, 134.3, 133.8, 129.9, 128.7, 128.2, 126.4, 82.7, 56.6, 21.0; MS (ESI) m/z (%) = 306.7 [M+Na]+. Anal. Calcd (%) for C17H17NO3: C 72.07, H 6.05, N 4.94. Found: C 72.06, H 6.02, N 4.93.
N-(2-(4-Chlorophenyl)-1-methoxy-2-oxoethyl)-N-phenylformamide (10a). Pale yellow solid, yield 12%; mp 145.1-146.3 °C; 1H NMR spectra (400 MHz, CDCl3, 25 °C): δ 8.50 (s, 1H, CHO), 6.99-7.76 (m, 9H, Ar-H), 6.92 (s, 1H, CH), 3.67 (s, 3H, OCH3); 13C NMR spectra (100 MHz, CDCl3, 25 °C): δ 190.8, 163.4, 140.4, 136.8, 132.7,129.6, 129.4, 129.1, 128.3, 126.2, 82.8, 56.6; MS (ESI) m/z (%) = 325.7 [M+Na]+. Anal. Calcd (%) for C16H14ClNO3: C 63.27, H 4.65, N 4.61. Found: C 63.25, H 4.63, N 4.59.
X-Ray diffraction analysis for 10a (Table 3 and Figure 3)
Crystals of 10a suitable for X-ray diffraction analysis were obtained by the slow evaporation of an EtOAc solution of 10a at room temperature. The single crystal X-ray diffraction measurement was conducted on a Rigaku Saturn CCD area-detector diffractometer at 113(2) K using graphite monochromated Mo Kα radiation (λ = 0.71070 Å) in the ω and φ scanning mode. An empirical absorption correction was applied using the ABSCOR program.25 All structures were solved by direct methods using the SHELXS-97 program26 and refined by full matrix least squares on F2 using the SHELXL-97 program.27 All of the hydrogen atoms were geometrically fixed using the riding model. Details, including the crystal data, data collection, and structure refinements, are summarized in Table 3. Deposition number CCDC-735378 forcompound 10a. Free copies of the data can be obtained via http://www.ccdc.cam.ac.uk/conts/retrieving.html (or from the Cambridge Crystallographic Data Centre, 12 Union Road, Cambridge, CB21EZ, UK; Fax: +44 1223 336033; e-mail: deposit@ccdc.cam.ac.uk).
ACKNOWLEDGMENTS
This work was financially supported by the Key Projects in the National Science & Technology Pillar Program (No. 2012ZX10001007-008-002) and the Doctoral Fund of Innovation of Beijing University of Technology.
References
1. P. J. Rybczynski, R. E. Zeck, J. Dudash, D. W. Combs, T. P. Burris, M. Yang, M. C. Osborne, X. L. Chen, and K. Demarest, J. Med. Chem., 2004, 47, 196. CrossRef
2. G. Caliendo, E. Perissutti, V. Santagada, F. Fiorino, B. Severino, D. Cirillo, R. Emmanuele, L. Lippolis, A. Pinto, and R. Sorrentino, Eur. J. Med. Chem., 2004, 39, 815. CrossRef
3. W. Yang, Y. Wang, Z. Ma, R. Golla, T. Stouch, R. Seethala, S. Johnson, R. Zhou, J. Feyen, and J. K. Dickson, Jr., Bioorg. Med. Chem. Lett., 2004, 14, 2327. CrossRef
4. P. L. Gentili, M. Nocchetti, C. Miliani, and G. Favaro, New J. Chem., 2004, 28, 379. CrossRef
5. G. Castaldi, P. Allegrini, R. Fusco, L. Longo, and V. Malatesta, J. Chem. Soc., Chem. Commun., 1991, 18, 1257. CrossRef
6. X. Sun, M. Fan, X. Meng, and E. Knobbe, J. Photochem. Photobiol., A, 1997, 102, 213.
7. P. S. Anderluh, M. Anderluh, J. Ilaš, J. Mravljak, D. M. Sollner, M. S. Dolenc, M. Stegnar, and D. Kikelj, J. Med. Chem., 2005, 48, 3110. CrossRef
8. D. Xu, A. Chiaroni, M. B. Fleury, and M. Largeron, J. Org. Chem., 2006, 71, 6374. CrossRef
9. J. Correia, J. Org. Chem., 1973, 38, 3433. CrossRef
10. R. Q. Li and H. Yan, Hecheng Huaxue, 2005, 13, 597.
11. C. L. Ni, X. H. Song, H. Yan, X. Q. Song, and R. G. Zhong, Ultrason. Sonochem., 2010, 17, 367. CrossRef
12. H. Yan, J. Y. He, Z. L. Tan, X. Q. Song, and R. G. Zhong, Inventor Patent CN101492424, 2009.
13. X. H. Zhu, C. L. Ni, H. Yan, and R. G. Zhong, J. Photopolym. Sci. Technol., 2009, 22, 379. CrossRef
14. H. Yan, H. Q. Wang, C. L. Ni, and X. Q. Song, Acta Crystallogr. Sect. E., 2006, 62, 1951. CrossRef
15. H. Yan, C. L. Ni, and H. Q. Wang, Inventor Patent CN 101041662, 2007.
16. J. L. He, Z. L. Tan, and H. Yan, Acta Crystallogr. Sect. E., 2009, 65, 1129. CrossRef
17. K. Mizuno, I. Nakanishi, N. Ichinose, and Y. Otsuji, Chem. Lett., 1989, 18, 1095. CrossRef
18. X. Zhang, C. S. Foote, and S. I. Khan, J. Org. Chem., 1993, 58, 47. CrossRef
19. W. Adam, M. Ahrweiler, K. Peters, and B. Schmiedeskamp, J. Org. Chem., 1994, 59, 2733. CrossRef
20. E. L. Clennan, Tetrahedron, 2000, 56, 9151. CrossRef
21. E. L. Clennan, Tetrahedron, 2005, 61, 6665. CrossRef
22. R. A. Larson and K. A. Marley, Environmental Photochemistry: Springer, 1999. pp. 123-37.
23. A. P. Schaap, S. D. Gagnon, and K. Zaklika, Tetrahedron Lett., 1982, 23, 2943. CrossRef
24. R. S. Handley, A. J. Stern, and A. P. Schaap, Tetrahedron Lett., 1985, 26, 3183. CrossRef
25. G. Sheldrick, SADABS, Program for Empirical Absorption Correction of Area Detector Data, University of Göttingen, Germany, 1996.
26. G. Sheldrick, SHELXS-97, Program for Crystal Structure Solution, University of Göttingen: Göttingen, Germany, 1997.
27. G. Sheldrick, SHELXL-97, Program for the Refinement of Crystal Structures from Diffraction Data, University of Göttingen, Göttingen, Germany, 1997.