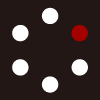
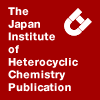
HETEROCYCLES
An International Journal for Reviews and Communications in Heterocyclic ChemistryWeb Edition ISSN: 1881-0942
Published online by The Japan Institute of Heterocyclic Chemistry
e-Journal
Full Text HTML
Received, 19th November, 2013, Accepted, 27th December, 2013, Published online, 14th January, 2014.
DOI: 10.3987/COM-13-12892
■ Synthetic Studies of Yessotoxin: Iterative Synthesis of the AB Ring System via Pd(II)-Catalyzed Cyclization of Alcohol
Hajime Yokoyama,* Yasuhiro Kusumoto, Koshiro Sumiyoshi, Masahiro Miyazawa, and Yoshiro Hirai*
Department of Chemistry, Graduate School of Science and Engineering, Toyama University, Gofuku 3190, Toyama 930-8555, Japan
Abstract
We report an iterative synthesis of the AB ring system of yessotoxin by using our Pd(II)-catalyzed cyclization reaction, as an approach towards a total synthesis.Marine dinoflagellates produce many natural products, including polyethers, with unique structures and biological activities. Yessotoxin is a polyether isolated from the digestive glands of the scallop Patinopecten yessoensis (Figure 1).1 Because of its complex structure and biological activities, which include the cytotoxicity and modulation of cytosolic calcium level in human lymphocytes, yessotoxin has attracted the attention of synthetic chemists.2 We have been studying the Pd(II)-catalyzed stereoselective cyclization reaction of urethane and alcohol,3 and herein we describe the application of this reaction for iterative synthesis of the AB ring system of yessotoxin.
Our starting material was the alcohol (1), which was prepared from 1,3-propanediol by 4 steps.4 Asymmetric dihydroxylation of 1 with AD-mix-α and MeSO2NH2 gave the triol (2) in 98% yield and 89% ee.5 In next protection step, we examined several conditions. At 0 °C, the protection of the triol (2) was selective and was achieved in the presence of 1.3 equivalents of p-MeOC6H4CH(OMe)2 and a catalytic amount of PPTS in CH2Cl2 for 0.5 h to give the acetal (3) in 93% yield. However at room temperature, 1,3-acetal (5) was obtained in 63% yield. This phenomenon could be due to the kinetic control at 0 °C. The hydroxyl group of 3 was protected with BnBr and TBS protection of the resulting benzyl ether was deprotected by TBAF treatment to afford the alcohol (4) in 94% yield (Scheme 1).
Oxidation of 4 with IBX, followed by continuous addition of cerium acetylide (prepared from tert-butyldimethyl(2-propynyloxy)silane with n-BuLi and CeCl3) provided the alcohol (6) as a diastereomixture in 85% yield. Lindlar hydrogenation of 6, followed by deprotection with TBAF treatment and regioselective cleavage6 of the acetal moiety gave the triol (7) in 55% yield (3 steps). Next the triol (7) was treated with 30 mol% PdCl2(PhCN)2 in THF at 0 °C to afford the alcohol (8) and diastereomer (9) on C5 position in the ratio of 60:40 in 55% yield.
Oxidation of the hydroxyl moiety, followed by reduction with NaBH4 provided the A ring system (8) and the diastereomer (9) in the ratio of 90:10 (Scheme 2). The A ring system (8) was isolated in 73% yield (2 steps) and the stereochemistry of 8 was determined by means of NOE experiments.7
Hydroboration of 8 with 9-BBN, followed by protection of two hydroxyl moeities with TBSOTf, 2,6-lutidine and deprotection of only primary hydroxyl moiety with TFA treatment gave the alcohol (10) in 73% yield (3 steps). Oxidation of 10 with IBX, followed by the addition of cerium acetylide gave the alcohol (11) as a diastereomixture of 50:50 in 86% yield (2 steps). Lindlar hydrogenation and the following deprotection of TBS protection of 11 with TBAF provided the allylic alcohol (12) in 47% yield (2 steps) (Scheme 3).
The allylic alcohol (12) was treated with 30 mol% PdCl2(PhCN)2 in THF at 0 °C to give the bicyclic compound (13) and diastereomer (14) in the ratio of 50:50 on C8 position in 78% yield. Successive oxidation and reduction of 13 and 14 provided the AB ring system (13) and the diastereomer (14) in the ratio of 90:10 (Scheme 4). The AB ring system (13) was isolated in 49% yield (2 steps) and the stereochemistry of 13 was determined by the coupling constant (J8-9 = 11.7 Hz) of C8-C9 and extensive NMR experiment.8
A plausible mechanism of the Pd(II)-catalyzed cyclization of 7 is shown in Figure 2. Pd π-complex is formed by coordination of PdCl2Ln with the allylic alcohol, and one of the π-faces of the olefin may be preferentially recognized with the assistance of the adjacent hydroxyl group. The resulting complex may be present as an equilibrium mixture of two structures (A and B). Conformation B is destabilized by non-bonding interaction between the Pd catalyst and Me group. Although conformation B is strongly destabilized by A1,2 strain, conformation A is not. Because of these two factors, the overall mechanism can account for the observed predominant formation of 8 and 9.
In the case of 12, conformation D would be unfavorable, because of non-bonding interaction between the Pd catalyst and juncture protons. The stereochemical outcome can be explained from conformation C (Figure 3).
In conclusion, we have synthesized the AB ring system of yessotoxin by means of Pd(II)-catalyzed cyclization. Further studies aimed at total synthesis of yessotoxin are under way in our laboratory.
ACKNOWLEDGEMENTS
This work was supported by Grant-in Aid for Scientific Research on Priority Areas (18032032 & 20590101) from The Ministry of Education, Culture, Sports, Science and Technology (MEXT), Japan.
References
1. (a) M. Murata, M. Kumagai, J. S. Lee, and T. Yasumoto, Tetrahedron Lett., 1987, 28, 5869; CrossRef (b) M. Satake, K. Terasawa, Y. Kadowaki, and T. Yasumoto, Tetrahedron Lett., 1996, 37, 5955; CrossRef (c) H. Takahashi, T. Kusumi, Y. Kan, M. Satake, and T. Yasumoto, Tetrahedron Lett., 1996, 37, 7087. CrossRef
2. Synthetic studies of yessotoxin and its analogs, see: (a) Y. Mori and H. Hayashi, Tetrahedron, 2002, 58, 1789; CrossRef (b) K. Suzuki and T. Nakata, Org. Lett., 2002, 4, 3943; CrossRef (c) Y. Mori, K. Nogami, H. Hayashi, and R. Noyori, J. Org. Chem., 2003, 68, 9050; CrossRef (d) Y. Mori, T. Takase, and R. Noyori, Tetrahedron Lett., 2003, 44, 2319; CrossRef (e) T. Oishi, K. Watanabe, and M. Murata, Tetrahedron Lett., 2003, 44, 7315; CrossRef (f) I. Kadota, H. Ueno, and Y. Yamamoto, Tetrahedron Lett., 2003, 44, 8935; CrossRef (g) K. Watanabe, M. Suzuki, M. Murata, and T. Oishi, Tetrahedron Lett., 2005, 46, 3991; CrossRef (h) I. Kadota, H. Ueno, Y. Sato, and Y. Yamamoto, Tetrahedron Lett., 2006, 47, 89; CrossRef (i) T. Oishi, M. Suzuki, K. Watanabe, and M. Murata, Tetrahedron Lett., 2006, 47, 3975; CrossRef (j) I. Kadota, T. Abe, Y. Sato, C. Kabuto, and Y. Yamamoto, Tetrahedron Lett., 2006, 47, 6545; CrossRef (k) K. Torikai, K. Watanabe, H. Minato, T. Imaizumi, M. Murata, and T. Oishi, Synlett, 2008, 2368; CrossRef (l) T. Oishi, T. Imaizumi, and M. Murata, Chem. Lett., 2010, 39, 108; CrossRef (m) T. Sakai, A. Sugimoto, H. Tatematsu, and Y. Mori, J. Org. Chem., 2012, 77, 11177.. CrossRef
3. (a) H. Yokoyama, S. Nakayama, M. Murase, M. Miyazawa, S. Yamaguchi, and Y. Hirai, Heterocycles, 2009, 77, 211; CrossRef (b) H. Yokoyama and Y. Hirai, Heterocycles, 2008, 75, 2133; CrossRef (c) H. Yokoyama, H. Kobayashi, M. Miyazawa, S. Yamaguchi, and Y. Hirai, Hetreocycles, 2007, 74, 283; CrossRef (d) H. Yokoyama, H. Ejiri, M. Miyazawa, S. Yamaguchi, and Y. Hirai, Tetrahedron: Asymmetry, 2007, 18, 852; CrossRef (e) M. Miyazawa, M. Narantsetseg, H. Yokoyama, S. Yamaguchi, and Y. Hirai, Heterocycles, 2004, 63, 1017; CrossRef (f) H. Yokoyama, K. Otaya, H. Kobayashi, M. Miyazawa, S. Yamaguchi, and Y. Hirai, Org. Lett., 2000, 2, 2427; CrossRef (g) Y. Hirai, J. Watanabe, T. Nozaki, H. Yokoyama, and S. Yamaguchi, J. Org. Chem., 1997, 62, 776. CrossRef
4. H. Yokoyama, Y. Hayashi, Y. Nagasawa, H. Ejiri, M. Miyazawa, and Y. Hirai, Tetrahedron, 2010, 66, 8458. CrossRef
5. Absolute configuration of the triol (2) was assigned tentatively by application of the Sharpless mnemonic. See: S. Alvarez, R. Alvarez, and A. R. deLera, Tetrahedron: Asymmetry, 2004, 15, 839. The enantiomeric ratio of the triol (2) was determined by 1H NMR analysis of O-methylmandelate ester derivatives of 2. CrossRef
6. (a) R. Johansson and B. Samuelsson, J. Chem Soc., Perkin Trans. 1, 1984, 10, 2371; CrossRef (b) P. J. Garegg, H. Hultberg, and S. Wallin, Carbohydr. Res., 1982, 108, 97. CrossRef
7. The selected data for 8: 1H NMR (600 MHz, CDCl3) δ: 7.32-7.29 (m, 2H), 7.28-7.22 (m, 5H), 6.84-6.82 (m, 2H), 5.8 (ddd, J = 16.6, 10.3, 7.0 Hz, 1H), 5.39 (ddd, J = 16.6, 1.5, 1.1 Hz, 1H), 5.31 (ddd, J = 10.6, 1.1, 0.7 Hz, 1H), 4.58 (d, J = 11.7 Hz, 1H), 4.56 (d, J = 11.7 Hz, 1H), 4.45 (d, J = 11.7 Hz, 1H), 4.39 (d, J = 11.7 Hz, 1H), 3.81-3.76 (m, 1H), 3.77 (s, 3H), 3.70 (dd, J = 12.0, 4.8 Hz, 1H), 3.53 (d, J = 10.5 Hz, 1H), 3.43 (d, J = 10.5 Hz, 1H), 3.33 (ddd, J = 12.0, 10.3, 4.8 Hz, 1H), 2.39 (ddd, J = 11.9, 4.8, 4.8 Hz, 1H), 1.62 (ddd, J = 12.0, 12.0, 11.9 Hz, 1H), 1.18 (s, 3H); 13C NMR (150 MHz, CDCl3) δ:159.1, 138.5, 136.5, 130.6, 129.4, 128.3, 127.50, 127.48, 119.1, 113.7, 77.5, 74.1, 73.7, 73.2, 71.3, 69.0, 55.2, 32.6, 13.7; IR (neat); 3741-3112, 3340, 1651 cm-1; EIMS m/z 398 (M+); HREIMS calcd. for C17H23O5 (M+-Bn) 307.1545, found 307.1540.
8. The selected data for 13: 1H NMR (600 MHz, CDCl3) δ: 7.30-7.17 (m, 7H), 6.84-6.83 (m, 2H), 5.84 (ddd, J = 17.4, 10.6, 7.5 Hz, 1H), 5.43 (dd, J = 17.4, 0.7 Hz, 1H), 5.36 (dd, J = 10.6, 0.7 Hz, 1H), 4.57 (d, J = 12.1 Hz, 1H), 4.53 (d, J = 11.7 Hz, 1H), 4.44 (d, J = 12.1 Hz, 1H), 4.34 (d, J = 11.7 Hz, 1H), 3.77 (s, 3H), 3.74 (dd, J = 11.7, 4.7 Hz, 1H), 3.54 (dd, J = 8.8, 7.5 Hz, 1H), 3.50 (d, J = 10.6 Hz, 1H), 3.49-3.44 (m, 1H), 3.41 (d, J = 10.6 Hz, 1H), 3.34 (ddd, J = 11.4, 9.3, 4.0 Hz, 1H), 3.05 (ddd, J = 11.7, 9.3, 4.7 Hz, 1H), 2.37 (ddd, J = 14.7, 4.7, 4.7 Hz, 1H), 2.36 (ddd, J = 13.9, 4.0, 4.0 Hz, 1H), 1.59-1.51 (m, 2H), 1.17 (s, 3H); 13C NMR (75 MHz, CDCl3) δ: 159.11, 138.41, 135.45, 132.44, 130.89, 130.47, 129.45, 128.81, 128.27, 127.52, 113.68, 83.77, 77.80, 77.22, 74.11, 73.11, 71.18, 69.05, 68.16, 55.22, 23.74, 22.99, 13.88; IR (neat); 3773-3098, 1613 cm-1; EIMS m/z 454 (M+); HREIMS calcd. for C20H27O6 (M+-Bn) 363.1808, found 363.1794.