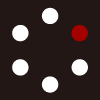
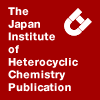
HETEROCYCLES
An International Journal for Reviews and Communications in Heterocyclic ChemistryWeb Edition ISSN: 1881-0942
Published online by The Japan Institute of Heterocyclic Chemistry
e-Journal
Full Text HTML
Received, 21st November, 2013, Accepted, 20th December, 2013, Published online, 25th December, 2013.
DOI: 10.3987/COM-13-12895
■ Facile Synthesis of Glycidates via Oxidation of Acrylates with Aqueous Solution of NaOCl in the Presence of Ammonium Salts
Bungo Ochiai* and Taiki Hirano
Faculty of Engineering, Department of Chemistry and Chemical Enginnering, Yamagata University, 3-16 Jonan 4-Chome, Yonezawa 992-8510, Japan
Abstract
Various glycidates were obtained in high yields via green oxidation of acrylates with aqueous solution of NaOCl in the presence of ammonium salts. The appropriate choice of ammonium salts fitting to the polarity of the substrates and the reaction under slightly basic conditions were essential for efficient reactions.Facile synthesis of epoxides with easily accessible reagents is a very important subject due to the high importance of epoxides both as intermediates1,2 and reactive3 materials. A typical method for preparing epoxides is oxidation of C=C double bond moieties.3,4-16 However, only a few effort has been conducted for efficient oxidation of acrylates yielding epoxides bearing ester moieties (glycidates) in spite of their wide variety of the commercial products. Glycidates are interesting building blocks for various pharmaceutical and biologically active compounds.5-7,9,17 Typical oxidants employed for this oxidation are NaOCl,5-10 dimethyldioxirane,11 t-BuOOH with BuLi,12,13 and H2O2 with hetero-polyacid14 or Mn15 catalysts and NaHCO3 as the activator.16 The yields attained by references 14 and 15 were quantitative, but the reagents used are not easily accessible. NaOCl is advantageous in price and accessibility. The oxidation without catalysts resulted in moderate yields, but a patent literature reported that use of methyltrioctylammonium chloride (Oct3MeNCl) as a phase transfer catalyst significantly improved the yields of the synthesis of n-butyl (BG) and ethyl glycidates from n-butyl (BA) and ethyl (EA) acrylates to 94 and 86%, respectively.10 We presume that the ammonium salts probably exchange the anion with NaOCl to obtain OCl- in place of Cl-, and served as amphiphilic oxidation species. Although oxidation of other α,β-unsaturated esters such as crotonates was also reported, oxidation of other acrylates was not disclosed. This oxidation was conducted in the mixture of NaOCl aqueous solution and ethyl acetate, and took relatively long time (e.g., 4 hours of dropwise addition of NaOCl solution and additional 3 hours of stirring). Accordingly, we examined the oxidation of various acrylates and optimized the conditions to obtain various glycidates in high yields.
First, the reaction conditions were optimized employing BA at 40 °C in 1 h in a similar manner with the patent literature (Scheme 1). The effect of organic solvent was examined using Bu4NCl (32 mol%) and NaHCO3 aqueous solution (pH = 8.2) (Table 1). We used a commercial 5wt% aqueous solution of NaOCl ([NaOCl]0/[BA]0 = 1.2). As a result, the best result was obtained without the use of organic solvents regardless of the polarity or the water-miscibility. Hydrolysis to produce a diol was responsible to the difference between the conversion of BA and the formation of BG.8 Longer time did not increase the conversion, but led to hydrolysis of the resulting epoxide. This result implies that additional organic solvents and longer times are not necessary in this case. Pure BG could be isolated by washing with brine after adding EtOAc followed by distillation or SiO2 column chromatography.
Next, the effect of pH was investigated using various aqueous solutions with pH values adjusted by NaHCO3 and acetic acid (Table 2). The conversions of BA and the formation of BG were identical under basic conditions. The conversions decreased under acidic conditions probably because decomposition of NaOCl by acetic acid. The slightly basic solution with the pH value of 8.2 resulted in the highest formation of BG owing to the suppression of hydrolysis of epoxide moieties (run 5). We accordingly used the aqueous solution of NaHCO3 with the pH value of 8.2.
The effect of the halide was also investigated (Table 3). The reaction without catalysts was also conducted as a reference, and resulted in a very unsuccessful result as reported.6,8 Both the conversions and the formations were in the order of Bu4NCl > Bu4NBr > Bu4NI. This result supports our presumption that the actual oxidation species are ammonium hypochlorites produced in situ via anionic exchange with NaOCl in a similar manner with the anion exchange between KOCl and an ammonium salt for enantioselective epoxidation of enones.18 Sodium halides are produced by this anion exchange that should proceed smoothly in the order of the affinity of the anions with Na+, namely Cl- > Br- > I-.
Under the optimized conditions, epoxidation of various alkyl acrylates were examined using various tetraalkylammonium chlorides (Figure 1). Catalysts with alkyl chains similar to substrates tended to gave higher yields. Oct3MeNCl, reported in the patent,10 was most suitable for quantitative formation of glycidates with long alkyl chains (92, 94, and 92% for n-Bu, 2-ethylhexyl, and n-dodecyl glycidates, respectively), but was not for the methyl (58%) and ethyl (69%) analogues. The decrease of the yield in the reaction of EA from the patent literature is attributable to the absence of EtOAc allowing the interaction between the oxidation species with the very low polarity and EA with the relatively high polarity.
Use of Bu4NCl having shorter alkyl chains was effective to the reactions of these acrylates with lower hydrophobicity, and the yields (82% and 80% for methyl and ethyl glycidate, respectively) were comparable to that reported in the patent. Cost effective Bu4NCl is advantageous over Oct3MeNCl. Catalysts with shorter chains were useless for the reactions of acrylates with long alkyl chains due to the very low ability as phase transfer catalysts. The highest yields for the examined substrates were comparable to those in the previous patent, and this reaction system is advantageous by the elimination of EtOAc from the reaction system and the significantly shortened reaction time.
In conclusion, various glycidates could be obtained via a facile and fast oxidation of acrylates by NaOCl aq. under ambient conditions. The use of ammonium catalysts with appropriate structures and the reaction under slightly basic conditions were important for selective reactions. The improvements from previous works are the shorter reaction time, the simpler procedure, and the cost effectiveness of the catalyst for methyl and ethyl glycidates. The organic solvent free reaction is also a potential advantage, and we are now trying to construct the reaction system excluding any organic solvents from the total synthetic process by optimizing the purification process. Investigations on the reactions of the glycidates are also ongoing.
EXPERIMENTAL
Materials. NaOCl was purchased as 5-wt% aqueous solution (Kanto Chemical, Tokyo, Japan). Water was distilled using a STILL ACE SA-2100A water distillation instrument (EYELA, Tokyo, Japan) prior to use. Other reagents were used as received.
Instruments. 1H (400 MHz) and 13C (100 MHz) nuclear magnetic resonance (NMR) spectra were measured on a JEOL ECX-400 instrument using tetramethylsilane as an internal standard. Resolved 1H-NMR spectra could be obtained using DMSO-d6 as a solvent, whereas the spectra obtained using CDCl3 showed overlaps of signals. Fourier transform infrared (FT-IR) spectra were measured on a JASCO FT/IR-470 Plus instrument. Elemental analysis was performed on a Perkin Elmer CHNS/O2400II analyzer.
Typical reaction procedure: synthesis of 2-ethylhexyl glycidate
MeOct3NCl (3.23 g, 40 mmol) and 2-ethylhexyl acrylate (EHA) (7.37 g, 400 mmol) were added to a test tube. Then, 5 wt-% NaOCl aq. (65.4 mL) and a buffer solution (7.18 g, 78 g/L) with pH (8.2) adjusted by NaHCO3 were added. The solution was stirred for 1 h at 40 °C. The contents were analyzed by 1H NMR spectroscopy to determine the conversion of the acrylate and the formation of the product. EtOAc (50 mL) and brine (50 mL) were added to the reaction mixture, and the separated organic layer was washed three times with brine (50 mL). The organic layer was dried with MgSO4. After volatile substances were evaporated off, the product was purified by silica gel column chromatography (7.68 g, 387 mmol, 96.8%).
1H NMR (DMSO-d6, 400 MHz, δ in ppm): 4.18–4.05 (m, 2H, -COOCH2-), 3.53 (dd, 2H, J = 3.2 and 3.6 Hz, -OCHCOO-), 2.91 (dd, 1H, J = 3.6 and 6.4 Hz, -OCH2CHCOO-), 2.83 (dd, 1H, J = 3.2 and 6.4 Hz, -OCH2CHCOO-), 1.60–1.50 (m, 1H, CH3CH2CH<), 1.35–1.24 (m, 2H, -CH(CH2CH3)(CH2)3-), 0.87 (t, 3H, J = 6.8 Hz, -CH(CH2CH3)), 0.86 (t, 3H, J = 7.6 Hz, -(CH2)3CH3). 13C NMR (CDCl3, 100 MHz, δ in ppm): 169.4 (>C=O), 67.8 (-COOCH2-), 47.3 (-CHCH2- in epoxy ring), 46.2 (-CHCH2- in epoxy ring), 38.6 (CH3CH2CH<), 30.2 (CH3CH2CH2CH2-), 28.8 (CH3CH2CH2-), 23.6 (CH3CH2CH<), 22.9 (CH3CH2CH2-), 14.0 (CH3(CH2)3-), 10.9 (CH3CH2CH<). Anal. Calcd for C11H20O3: C 65.97; H 10.07. Found: C 65.95; H 10.10.
Spectroscopic data of other novel compounds.
n-Hexyl glycidate. 1H NMR (CDCl3, 400 MHz, δ in ppm): 4.09 (t, 2H, J = 6.8 Hz, -COOCH2-), 3.52 (dd, 1H, J = 3.7 and 3.6 Hz, -CH-), 2.94 (dd, 1H, J = 3.6 and 6.0 Hz, -OCH2CHCOO-), 2.82 (dd, 1H, J = 3.6 and 6.4 Hz, -OCH2CHCOO-), 1.80–1.60 (m, 2H, -COOCH2CH2-), 1.40–1.25 (m, 6H, -(CH2)3CH3), 0.85 (t, 3H, J = 7.0 Hz, -CH3). 13C NMR (CDCl3, 100 MHz, δ in ppm): 169.3 (>C=O), 66.5 (-COOCH2-), 47.3 (-CHCH2- in epoxy ring), 46.2 (-CHCH2- in epoxy ring), 31.4 (-COOCH2CH2-), 28.4 (CH3CH2CH2CH2-), 25.4 (CH3CH2CH2-), 22.4 (CH3CH2-), 13.9 (CH3-). Anal. Calcd for C9H16O3: C 62.77; H 9.36. Found: C 62.72; H 9.38.
n-Dodecyl glycidate. 1H-NMR (400 MHz, CDCl3 at rt, δ in ppm): 4.21 (m, 2H, C10H21-CH2-), 3.43 (dd, 1H, J = 2.0 and 4.2 Hz, -CH-), 2.95 (dd, 1H, J = 2.0 and 6.6 Hz, -OCH2CHCOO-), 2.82 (dd, 1H, J = 4.2 and 6.6 Hz, -OCH2CHCOO-), 1.64 (m, 2H, -O-CH2-CH2), 1.37 (m, 2H, -CH2-C9H18-CH2-), 0.9 (d, 3H, J = 7.5 Hz, -CH2-CH3). 13C-NMR (100 MHz, CDCl3 at rt, δ in ppm): 169.4 (>C=O), 65.9 (-COOCH2-), 47.4 (-CHCH2- in epoxy ring), 46.4 (-CHCH2- in epoxy ring), 32.0 (-COOCH2CH2-), 29.7 (-OCH2CH2CH2-), 29.6-29.2 (-O(CH2)3(CH2)3-), 28.5 (CH3CH2CH2CH-), 25.8 (CH3CH2CH2-), 22.8 (CH3CH2-), 14.2 (CH3-). Anal. Calcd for C15H28O3: C 70.27; H 11.01. Found: C 70.23; H 10.97.
Syntheses of methyl glycidate,8 ethyl glycidate,10 and n-butyl glycidate10 have already been reported.
ACKNOWLEDGEMENT
The authors thank Mr. Takeyoshi Takahashi for his contribution to elemental analysis.
References
1. O. A. Wong and Y. Shi, Chem. Rev., 2008, 108, 3958. CrossRef
2. R. Noyori, M. Aoki, and K. Sato, Chem. Commun., 2003, 1977. CrossRef
3. K. Dusek, Adv. Polym. Sci., 1986, 78, 1; CrossRef K. D. Weiss, Prog. Polym. Sci., 1997, 22, 203; CrossRef B. Ochiai and T. Endo, Prog. Polym. Sci., 2005, 30, 183. CrossRef
4. A. Nemes, L. Czibula, G. Visky, M. Farkas, and J. Kreidl, Heterocycles, 1991, 32, 2329. CrossRef
5. J. Pitlik and C. A. Townsend, J. Labelled Comp. Radiopharm., 1997, 39, 999. CrossRef
6. K. B. Hansen, P. Rabbat, S. A. Springfield, P. N. Devine, E. J. J. Grabowski, and P. J. Reider, Tetrahedron Lett., 2001, 42, 8743. CrossRef
7. B. Moon, S. Han, and D. Kim, Org. Lett., 2005, 7, 3359. CrossRef
8. C. P. Stevenson, L. P. C. Nielsen, and N. E. Jacobsen, Org. Synth., 2006, 83, 162.
9. O. Barykina and B. B. Snider, Org. Lett., 2010, 12, 2664. CrossRef
10. Y. Moriwaki and R. Akaishi, Jpn. Kokai Tokkyo Koho, H5-39277, 1993.
11. A. L. Baumstark P. C. Vasquez, M. Aly, and J. P. Sansone, Heterocycl. Commun., 2010, 16, 183.
12. C. Clark, P. Hermans, O. Meth-Cohn, C. Moore, H. C. Taljaard, and G. van Vuuren, J. Chem. Soc., Chem. Commun., 1986, 1378. CrossRef
13. O. Meth-Cohn, C. Moore, and H. C. Taljaard, J. Chem. Soc., Perkin Trans. 1, 1988, 2663. CrossRef
14. A. Berkessel and C. A. Sklorz, Tetrahedron Lett., 1999, 40, 7965. CrossRef
15. K. Kamata, K. Sugahara, K. Yonehara, R. Ishimoto, and N. Mizuno, Chem. Eur. J., 2011, 17, 7549. CrossRef
16. H. Yao and D. E. Richardson, J. Am. Chem. Soc., 2000, 122, 3220. CrossRef
17. T. Yamaguchi, N. Harada, K. Ozaki, M. Hayashi, H. Arakawa, and T. Hashiyama, Tetrahedron, 1999, 55, 1005. CrossRef
18. E. J. Corey and F. Y. Zhang, Org. Lett., 1999, 1, 1287. CrossRef