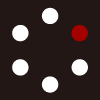
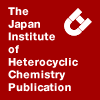
HETEROCYCLES
An International Journal for Reviews and Communications in Heterocyclic ChemistryWeb Edition ISSN: 1881-0942
Published online by The Japan Institute of Heterocyclic Chemistry
e-Journal
Full Text HTML
Received, 28th November, 2013, Accepted, 6th January, 2014, Published online, 20th January, 2014.
DOI: 10.3987/COM-13-12903
■ An Improved Synthesis of Di-Nitro-Functionalized trans-A2B2-Tetraphenylporphyrins
Zheng-Lin Zhang, Yuan-Bin She,* Hai-Yan Fu,* Xu-Feng Song, and Zhi-Cheng Sun
Department of Chemistry & Chemical Engineering, Institute of Green Chemistry & Fine Chemicals, Beijing University of Technology, 100 Ping Le Yuan, Chaoyang District, Beijing 100124, China
Abstract
An improved Macdonald-type 2+2 condensation was used to synthesize a series of di-nitro-functionalized trans-A2B2-tetraphenylporphyrins, and all the structures were characterized by melting point analysis, electronic absorption spectroscopy, infrared spectroscopy, 1H NMR spectroscopy, high-resolution mass spectroscopy, and elemental analysis. The synthesis process was systematically investigated, and several important factors were examined to increase the product yield. The optimum reaction conditions were then established, and the method was found to have the advantages of high reactant concentration, no quinone as oxidant, and good yields of 5,15-bis(p-nitrophenyl)-10,20-bis(p-R-phenyl)porphyrins (R = H, F, Cl, Br, CH3, and CH3O). Moreover, side reactions, such as scrambling, could be suppressed easily by regulating reaction condition.Among variously substituted meso-tetraphenylporphyrins, nitryl-substituted porphyins have always attracted the attention of researchers. The nitro group, which is an important functional group, can be further transformed into various derivatives for different applications. Moreover, trans-A2B2-porphyins, given their special symmetry structure in the porphyrin macro ring, modified by the nitro group can be used as important precursors making them applicable in artificial photosynthesis,1-3 chiral catalysts,4-7 potential medicines for diagnosis and therapy of cancer,8-10 dye-sensitized solar cell,11,12 and polymeric materials.13
Three kinds of methods are currently used in the synthesis processes to di-nitro-functionalized trans-A2B2-tetraphenylporphyrin (trans-A2B2-TPP), including mixed-aldehyde condensation,14 nitration of p-phenyl groups of TPP,10, 15-17 and MacDonald-type 2+2 condensation.12,13,18,19 For mixed-aldehyde condensation, the theoretical yield of trans-A2B2-porphyrin can presently reach a maximum value of 12.5% of total porphyrins. However, 25% A3B-TPP, 25% AB3-TPP, 25% cis-A2B2-TPP, 6.25% A4-TPP, and 6.25% B4-TPP in the porphyrins have been achieved as well. Apparently, mixed-aldehyde condensation does not yield trans-A2B2-TPP as the main product. Furthermore, a significant yield of cis-A2B2-TPP, composed with the same number of substituents as trans-A2B2-TPP, have been obtained through the mixed-aldehyde condensation, which would make the separation of trans-A2B2-TPP from cis-A2B2-TPP become a difficult task. Kruper et al.15 investigated the nitration of p-phenyl groups of TPP to obtain nitro-TPP by using fuming nitric acid. Since then, many researchers10,16,17,20 improved the nitration condition to obtain mono-, di-, tri-, and tetra-nitro-TPPs selectively. When the nitration was controlled to obtain di-nitro-TPP, the reaction produces cis-A2B2-TPP as the main product and trans-A2B2-TPP as the minor product. Zhang et al.21 showed that the electron-withdrawing nitro group would lead to the preferential occurrence of the second nitration to the neighboring phenyl ring, resulting in cis-A2B2-TPP as the main product. Therefore, the synthesis and separation of di-nitro-functionalized trans-A2B2-TPP are inconvenient to perform through the methods of mixed-aldehyde condensation or nitration of TPP. In view of the symmetrical structure of trans-A2B2-TPP, MacDonald-type 2+2 condensation may be a good choice for the synthesis of trans-A2B2-TPP. Most MacDonald-type 2+2 condensations follow the method of Lindsey et al.,13,18,19 which has relatively low reactant concentrations (only 10 mmol/L) require expensive DDQ or TCQ to oxidize porphyrinogen to porphyrin and is unfavorable for large-scale preparation and resource conversation.22 Nian Lin et al.23 have recently reported that 5-(p-nitrophenyl)dipyrromethane (NPDM (1)) directly reacted with benzaldehyde (PhCHO (2’)) in refluxing propanoic acid and produced 5,15-bis(p-nitrophenyl)-10,20-diphenylporphyrin (trans-(p-NO2Ph)2(Ph)2 (2)) with 20% yield. Besides, in the synthesis of symmetric porphyrins, a slight change of condition of solvent, such as mixed solvent of nitroaromatic compound and carboxylic acid, could considerably influence the result of reaction,22,24 which inspired us to find a more efficient and simple method for the synthesis of di-nitro-functionalized trans-A2B2-TPP.
In this paper, we present an improved MacDonald-type 2+2 condensation to synthesize di-nitro-functionalized trans-A2B2-TPP with a high spectral yield up to 31.2%, which was performed with NPDM and aromatic aldehyde dissolved in a mixed solvent of nitrobenzene and acetic acid. The synthesis process was systematically investigated, and several important factors were explored. The optimum reaction conditions were then established, and the method was found to have the advantages of high reactant concentration, no quinone as oxidant, and good yields of 5,15-bis(p-nitrophenyl)-10,20-bis(p-R-phenyl)porphyrins (R = H, F, Cl, Br, Me, and MeO). Furthermore, side reactions, such as scrambling, could be suppressed easily by regulating reaction condition.
In this paper, the condensation of NPDM + PhCHO to obtain trans-(p-NO2Ph)2(Ph)2 as the model reaction was investigated. The ideal route of the model reaction is that the acid-catalyzed condensation of NPDM and PhCHO form the corresponding porphyrinogen; and then the porphyrinogen is oxidized to give trans-(p-NO2Ph)2(Ph)2 (Scheme 1). Unfortunately, the decomposition of NPDM and the rearrange- ment of porphyrinogen would occur under acidic conditions, resulting in scrambling products.25 Thus, the suppression of the acid-catalyzed scrambling process is the key solution for obtaining more trans-(p-NO2Ph)2(Ph)2.
As can be seen in Scheme 1, the condensation of NPDM + PhCHO and the decomposition of NPDM competed with each other under certain acid-catalyzed condition. The choice of suitable reaction conditions is important, that is, the conditions should be favorable for condensation of NPDM + PhCHO, obtaining trans-(p-NO2Ph)2(Ph)2, and suppressing scrambling porphyrins. Several factors were discussed to determine the optimum conditions for the synthesis of trans-(p-NO2Ph)2(Ph)2.
The effect of reaction temperature was examined in the range from 70 to 145 °C (refluxing temperature). NPDM (1 mmol) and PhCHO (1 mmol) were dissolved in nitrobenzene (10 mL) and reacted under the catalysis of acetic acid (5 mL) for 1 h at a certain temperature. The results, as summarized in Table 1, show the influence of reaction temperature. The yield of trans-(p-NO2Ph)2(Ph)2 increased with the raising of reaction temperature up to 100 °C. The spectral yield of trans-(p-NO2Ph)2(Ph)2 slightly fluctuated at approximately 30% with the further increase in reaction temperature. High reaction temperature increases the degree of the decomposition of NPDM, producing more scrambling porphyrins and high amounts of tar, which make purification difficult. Thus, the temperature of 100 °C is a good choice because it could suppress decomposition of NPDM and provide a good yield with a relatively low temperature.
Nitrobenzene amount and acetic acid amount were found to be critical parameters under our reaction conditions. As can be seen in Table 2 (entries 1–5), the addition of suitable amount of nitrobenzene significantly raised the yield of trans-(p-NO2Ph)2(Ph)2 and restrained the generation of tar. However, further increase in nitrobenzene amount would drastically low the yield (entries 5–7). On the other side, without acetic acid, the model reaction would not generate any porphyrin, proving that the condensation of NPDM + PhCHO was an acid-catalyzed process (entry 7). These observations indicate that suitably relative amounts of nitrobenzene as solvent and acetic acid as catalyst were necessary to accommodate concentration of the acid, which could promote the condensation of NPDM + PhCHO and suppress the decomposition of NPDM. As shown in Table 2, a good yield of trans-(p-NO2Ph)2(Ph)2 can be obtained using 10 mL of nitrobenzene and 5 mL of acetic acid.
The reactant concentration also is an important factor for the condensation of NPDM + PhCHO. Thus, the effects of reactant concentration on the reaction were explored to determine the suitable point with optimum ratio of 2 mL/1 mL of nitrobenzene/acetic acid according to Table 2, and the results are shown in Table 3. The increase in the yield of trans-(p-NO2Ph)2(Ph)2 occurred until the reactant concentration reached 131 mM. However, the yield decreased with the continuous increase in reactant concentration. The results indicate that low concentration was not conducive to the condensation of NPDM + PhCHO, while an excessively high concentration may cause excessive condensation of NPDM + PhCHO, both of which would be adverse to the formation of porphyrinogen. The model reaction produced a high yield at the reactant concentration of 131 mM, which was achieved with 1 mmol of NPDM and 1 mmol of PhCHO in 5 mL of nitrobenzene and 2.5 mL of acetic acid.
The condensation of NPDM + PhCHO and decomposition of NPDM are acid-catalyzed reactions, and thus, the nature of acid catalyst can significantly affect the selection of the reaction route. A series of straight chain alkyl acids was chosen to investigate the effect of acid catalyst on the model reaction. The condensation of 1 mmol of NPDM and 1 mmol of PhCHO in 5 mL of nitrobenzene was catalyzed by the equimolar of different straight chain alkyl carboxylic acids. The results are provided in Table 4. Formic acid, which can promote the decomposition of NPDM, produced large numbers of scrambling porphyrins and only 1.6% of trans-(p-NO2Ph)2(Ph)2. From acetic acid to n-heptanoic acid, the yield of trans-(p-NO2Ph)2(Ph)2 decreased with the increase in the lengths of alkyl chain of acid catalysts. Interestingly, n-hexanoic acid or n-heptanoic acid, weak acid catalyst, which would suppress the condensation of NPDM + PhCHO to leading a bad yield of trans-(p-NO2Ph)2(Ph)2, suppress the decomposition of NPDM to resulting in less scrambling porhyrins and was conducive to the separation of trans-(p-NO2Ph)2(Ph)2. In view of the scrambling effect and the yield of trans-(p-NO2Ph)2(Ph)2, acetic acid was selected as the acid catalyst in the following study.
As discussed above, the preferred reaction condition includes 1 mmol of NPDM and 1 mmol of PhCHO in 5 mL of nitrobenzene and 2.5 mL of acetic acid at 100 °C for 1 h, which could give a spectral yield of 31.2% of trans-(p-NO2Ph)2(Ph)2 (with isolated yield of 26.0%). Only a small amount of scrambling porphyrins was found. The reasons for the efficient suppressing scrambling are as follows: 1. the nitro group of NDPM, as electron-withdrawing group, made the decomposition of NDPM difficult,19 and 2. the suitable reaction condition resulted in the desired reaction route. Furthermore, the degree of scrambling should be high relative to the nature of the used aldehyde. Then, the optimized procedure was repeated for the preparation of 5,15-bis(p-nitrophenyl)-10,20-bis(p-R-phenyl)porphyrins (8-12) from NDPM and aromatic aldehydes (8’-12’) (Scheme 2 and Table 5).
The isolated yields of 5,15-bis(p-nitrophenyl)-10,20-bis(p-R-phenyl)porphyrins fluctuated between 20% and 25%, indicating that the substituent had insignificant electronic effect on the yield of 5,15-bis(p-nitrophenyl)-10,20-bis(p-R-phenyl)porphyrin. However, the electronic effect of the substituent affected the degree of scrambling. Small amounts of 5,10,15-tri(p-nitrophenyl)-20-(p-fluorophenyl)-porphyrin (17) and 5-(p-nitrophenyl)-10,15,20-tri-(p-fluorophenyl)porphyrin (14) were observed in the NPDM + p-fluorobenzaldehyde (8’); while no 5-(p-nitrophenyl)-10,15,20-tri(p-methylphenyl)porphyrin (15) generated from NPDM + p-methylbenzaldehyde (11’), but a small amount of 5,10,15-tri(p-nitrophenyl)-20-(p-methylphenyl)porphyrin (18) was found. Different degrees of scrambling observed in the NPDM + aromatic aldehydes might imply different reaction route for each.
The different degrees of scrambling of NPDM + aromatic aldehydes might follow the mechanism shown in Scheme 3.26 For the aromatic aldehyde with electron-releasing group, such as Me, the scrambling tended to route B, generating more stable carbocation B (16) intermediate and resulting in the formation of 5,10,15-tri(p-nitrophenyl)-20-(p-methylphenyl)porphyrin (18). For the aromatic aldehyde with electron-withdrawing group, such as F, although scrambling mainly followed route B, with improving the stability of carbocation A (13) intermediate, the probability of scrambling via route A increased, and resulting in the formation of 5-(p-nitrophenyl)-10,15,20-tri(p-fluorophenyl)porphyrin (14).
In summary, we developed a simple method of using the mixed solvent of nitrobenzene and acetic acid to synthesize 5,15-bis(p-nitrophenyl)-10,20-bis(p-R-phenyl)porphyrin. The method allowed high reactant concentration (131 mmol/L) and generated a good yield of 5,15-bis(p-nitrophenyl)-10,20-bis(p-R-phenyl)porphyrin, but it did not require quinone as oxidant. Furthermore, we found that the degree of scrambling could be controlled by selecting the suitable reaction condition and that the electronic effect of the substituents would affect the route of scrambling, which could cause the formation of different scrambling porphyrins.
EXPERIMENTAL
Melting points were recorded on SGW X-4B melting-point apparatus and are uncorrected. The 1H NMR spectra were obtained using a Bruker AV 400 M spectrometer in solvent (CDCl3/TFA) with TMS as internal reference. Electronic absorption spectra were measured using a UV1901 spectrophotometer (Shanghai Phenix). High resolution mass spectra (ESI) were obtained on a Bruker micrOTOF-QII. Infrared spectra (IR) were recorded as thin films on KBr plates with a Bruker Vertex 70 spectrometer. Elemental analysis was performed on a Euro Vector EA3000. Column chromatography was performed using silica gel (300-400 mesh) produced by Qingdao Marine Chemical Factory, Qingdao (China). Commercially available solvents and reagents were used without further purification. The NDPM that was not commercially available was synthesized following procedures described in the literature.27
General method for the synthesis of 5,15-bis(p-nitrophenyl)-10,20-bis(p-R-phenyl)porphyrin
1 mmol NDPM and 1 mmol aromatic aldehyde were added to a mixture of 5 mL nitrobenzene and 2.5 mL acetic acid preheated to 100 °C. After 1 h of stirring, the mixture was cooled to room temperature. Then, nitrobenzene and acetic acid were evaporated under reduced pressure, and the residue was purified by silica gel column chromatography with mixture of CH2Cl2 and n-hexane. After evaporation, the crude product was washed with methanol via centrifugation, resulting in pure 5,15-bis(p-nitrophenyl)-10,20-bis(p-R-phenyl)porphyrin.
General method for measuring the spectral yield of 5,15-bis(p-nitrophenyl)-10,20-diphenylporphyrin (2)
The solutions, which concentrations ranged from 1.0217 to 2.0434 × 10-6 mol/L, were prepared by dissolving and diluting purified 5,15-bis(p-nitrophenyl)-10,20-diphenylporphyrin with CH2Cl2 as solvent. According to the Beer-Lambert law, the linear regression equation as A = 298508C + 0.0399 (the regression coefficient R2 = 0.9997) could be constructed by the concentration (C (mol/L)) and the corresponding absorbance (A) of the Soret band (λmax = 420.1 nm) of 5,15-bis(p-nitrophenyl)-10,20- diphenylporphyrin solution. Subsequently, the unknown concentration of the diluted crude product of 5,15-bis(p-nitrophenyl)-10,20-diphenylporphyrin could be found with the measured absorbance via the linear regression equation, and then the corresponding spectral yield could be acquired by relevant calculation.
5,15-Bis(p-nitrophenyl)-10,20-diphenylporphyrin (2)
Purple powder. Spectral yield 31.2% (isolate yield 26.0%). Mp >300 °C. UV-vis (CH2Cl2) λmax/nm (logε): 420.1 (5.48), 515.9 (4.41), 551.9 (4.20), 590.4 (4.07), 646.5 (4.03). IR (KBr): 3317.95, 3101.63, 3070.84, 1595.00, 1558.79, 1516.04, 1472.54, 1400.30, 1344.14, 1223.13, 1186.39, 1107.42, 1018.78, 965.16, 847.26, 801.04, 746.92, 725.72, 700.42 cm-1. HRMS (ESI): m/z [M+H]+ calculated for C44H28N6O4+H: 705.2245, found: 705.2183. 1H NMR (400 MHz, CDCl3/TFA) δ 8.900 (d, J = 8.4 Hz, 4 H), 8.793 (d, J = 8.4 Hz, 4 H), 8.765 (d, J = 4.8 Hz, 4 H), 8.688 (d, J = 4.8 Hz, 4 H), 8.574 (t, J = 3.6 Hz, 4 H), 8.068 (d, J = 4.8 Hz, 6 H), -0.417 (s, 4 H). Anal. Calcd for C44H28N6O4: C 74.99, H 4.00, N 11.93. Found: C 72.71, H 4.07, N 11.40.
5,15-Bis(p-nitrophenyl)-10,20-bis(p-fluorophenyl)porphyrin (8)
Purple powder. Isolated yield 25.1%. Mp >300 °C. UV-vis (CH2Cl2) λmax/nm (logε): 419.8 (5.53), 515.9 (4.47), 551.8 (4.25), 590.4 (4.12), 645.9 (4.05). IR (KBr): 3318.29, 3107.17, 3075.98, 1596.51, 1559.67, 1519.53, 1473.82, 1400.08, 1347.49, 1224.38, 1157.47, 1108.10, 1016.67, 966.67, 850.52, 798.31, 729.10 cm-1. HRMS (ESI): m/z [M+H]+ calculated for C44H26F2N6O4+H: 741.2056, found: 741.1959. 1H NMR (400 MHz, CDCl3/TFA) δ 8.900 (d, J = 8.4 Hz, 4 H), 8.775 (d, J = 8.4 Hz, 4 H), 8.731 (d, J = 4.8 Hz, 4 H), 8.694 (d, J = 4.8 Hz, 4 H), 8.564 (dd, J1 = 8.4 Hz, J2 = 5.2 Hz, 4 H), 7.781 (t, J = 8.4 Hz, 4 H), -0.350 (s, 4 H). Anal. Calcd for C44H26F2N6O4: C 71.35, H 3.54, N 11.35. Found: C 70.71, H 3.82, N 10.91.
5,15-Bis(p-nitrophenyl)-10,20-bis(p-chlorophenyl)porphyrin (9)
Purple powder. Isolated yield 20.3%. Mp >300 °C. UV-vis (CH2Cl2) λmax/nm (logε): 420.7 (5.28), 515.9 (4.13), 552.1 (3.85), 589.7 (3.69), 646.5 (3.58). IR (KBr): 3320.08, 3102.71, 3073.31, 1594.79, 1559.88, 1517.25, 1472.24, 1397.42, 1345.46, 1216.57, 1178.40, 1091.68, 1017.14, 965.92, 849.30, 800.98, 734.54 cm-1. HRMS (ESI): m/z [M+H]+ calculated for C44H26Cl2N6O4+H: 773.1465, found: 773.1350. 1H NMR (400 MHz, CDCl3/TFA) δ 8.905 (d, J = 8.4 Hz, 4 H), 8.773 (d, J = 8.4 Hz, 4 H), 8.745 (d, J = 4.4 Hz, 4 H), 8.695 (d, J = 4.4 Hz, 4 H), 8.509 (d, J = 8.0 Hz, 4 H), 8.063 (d, J = 8.0 Hz, 4 H), -0.357 (s, 4 H). Anal. Calcd for C44H26Cl2N6O4: C 68.31, H 3.39, N 10.86. Found: C 69.77, H 3.70, N 10.12.
5,15-Bis(p-nitrophenyl)-10,20-bis(p-bromophenyl)porphyrin (10)
Purple powder. Isolated yield 19.7%. Mp >300 °C. UV-vis (CH2Cl2) λmax/nm (logε): 421.0 (5.49), 516.0 (4.39), 551.7 (4.17), 590.4 (4.05), 645.9 (3.97). IR (KBr): 3321.31, 3101.22, 3076.35, 1595.25, 1560.17, 1518.67, 1472.36, 1393.16, 1345.83, 1220.34, 1182.67, 1105.61, 1013.07, 966.11, 848.89, 799.35, 732.38 cm-1. HRMS (ESI): m/z [M+H]+ calculated for C44H26Br2N6O4+H: 863.0440, found: 863.0328. 1H NMR (400 MHz, CDCl3/TFA) δ 8.900 (d, J = 8.8 Hz, 4 H), 8.772 (d, J = 8.8 Hz, 4 H), 8.736 (d, J = 4.8 Hz, 4 H), 8.684 (d, J = 4.8 Hz, 4 H), 8.433 (d, J = 8.4 Hz, 4 H), 8.220 (d, J = 8.4 Hz, 4 H), -0.166 (s, 4 H). Anal. Calcd for C44H26Br2N6O4: C 61.27, H 3.04, N 9.74. Found: C 60.85, H 3.34, N 9.38.
5,15-Bis(p-nitrophenyl)-10,20-bis(p-methylphenyl)porphyrin (11)
Purple powder. Isolated yield 24.3%. Mp >300 °C. UV-vis (CH2Cl2) λmax/nm (logε): 421.2 (5.49), 517.1 (4.43), 553.1 (4.25), 591.7 (4.08), 647.5 (4.05). IR (KBr): 3319.42, 3104.01, 3075.62, 3020.47, 2919.36, 2851.40, 1595.46, 1560.35, 1517.10, 1473.35, 1400.09, 1345.63, 1220.87, 1183.83, 1108.77, 1019.98, 966.12, 848.03, 800.06, 726.93 cm-1. HRMS (ESI): m/z [M+H]+ calculated for C46H32N6O4+H: 733.2558, found: 733.2479. 1H NMR (400 MHz, CDCl3/TFA) δ 8.896 (d, J = 8.8 Hz, 4 H), 8.804 (d, J = 8.4 Hz, 4 H), 8.725 (d, J = 4.8 Hz, 4 H), 8.646 (d, J = 4.8 Hz, 4 H), 8.481 (d, J = 8.0 Hz, 4 H), 7.880 (d, J = 7.6 Hz, 4 H), 2.825 (s, 6 H), -0.014 (s, 4 H). Anal. Calcd for C46H32N6O4: C 75.40, H 4.40, N 11.47. Found: C 72.59, H 4.21, N 10.39.
5,15-Bis(p-nitrophenyl)-10,20-bis(p-methoxyphenyl)porphyrin (12)
Purple powder. Isolated yield 24.2%. Mp >300 °C. UV-vis (CH2Cl2) λmax/nm (logε): 423.4 (5.58), 518.2 (4.49), 555.5 (4.38), 592.9 (4.23), 649.0 (4.22). IR (KBr): 3315.23, 3103.62, 3072.92, 2928.43, 2835.77, 1595.44, 1557.91, 1515.69, 1473.13, 1400.70, 1345.94, 1287.45, 1247.60, 1175.78, 1107.32, 1031.93, 965.67, 848.84, 798.66, 727.95 cm-1. HRMS (ESI): m/z [M+H]+ calculated for C46H32N6O6+H: 765.2456, found: 765.2385. 1H NMR (400 MHz, CDCl3/TFA) δ 8.886 (d, J = 8.4 Hz, 4 H), 8.783 (d, J = 8.8 Hz, 4 H), 8.690 (d, J = 4.8 Hz, 4 H), 8.638 (d, J = 4.8 Hz, 4 H), 8.514 (d, J = 8.4 Hz, 4 H), 7.595 (d, J = 8.8 Hz, 4 H), 4.195 (s, 6 H), -0.268 (s, 4 H). Anal. Calcd for C46H32N6O6: C 72.24, H 4.22, N 10.99. Found: C 72.32, H 4.49, N 10.52.
ACKNOWLEDGEMENTS
This work was supported by the Key Program and the General Projects of National Natural Science Foundation of China (Grant No. 21276006, 21036009, 21076004, 21205145), the Funding Project for Academic Human Resources Development in Institutions of Higher Learning under the Jurisdiction of Beijing Municipality (Grant No. PHR 201107104), and the Talents Fund and Team Program of South-Central University for Nationalities (YZZ 12002, XTZ13002).
References
1. T. A. Moore, D. Gust, P. Mathis, J.-C. Mialocq, C. Chachaty, R. V. Bensasson, E. J. Land, D. Doizi, P. A. Liddell, W. R. Lehman, G. A. Nemeth, and A. L. Moore, Nature, 1984, 307, 630. CrossRef
2. G. Steinberg-Yfrach, J.-L. Rigaud, E. N. Durantini, A. L. Moore, D. Gust, and T. A. Moore, Nature, 1998, 392, 479. CrossRef
3. M. Hambourger, G. F. Moore, D. M. Kramer, D. Gust, A. L. Moore, and T. A. Moore, Chem. Soc. Rev., 2009, 38, 25. CrossRef
4. S. Zhu, J. V. Ruppel, H. Lu, L. Wojtas, and X. P. Zhang, J. Am. Chem. Soc., 2008, 130, 5042. CrossRef
5. Y. Chen, J. V. Ruppel, and X. P. Zhang, J. Am. Chem. Soc., 2007, 129, 12074. CrossRef
6. Y. Chen and X. P. Zhang, J. Org. Chem., 2007, 72, 5931. CrossRef
7. Y. Chen, K. B. Fields, and X. P. Zhang, J. Am. Chem. Soc., 2004, 126, 14718. CrossRef
8. D. Kessel, R. Luguya, and M. G. H. Vicente, Photochem. Photobiol., 2003, 78, 431. CrossRef
9. G. G. Meng, B. R. James, and K. A. Skov, Can. J. Chem.-Rev. Can. Chim., 1994, 72, 1894. CrossRef
10. R. Luguya, L. Jaquinod, F. R. Fronczek, M. G. H. Vicente, and K. M. Smith, Tetrahedron, 2004, 60, 2757. CrossRef
11. M. G. Walter, C. C. Wamser, J. Ruwitch, Y. Zhao, D. Braden, M. Stevens, A. Denman, R. Pi, A. Rudine, and P. J. Pessiki, J. Porphyr. Phthalocyanines, 2007, 11, 601. CrossRef
12. M. E. Milanesio, M. Gervaldo, L. A. Otero, L. Sereno, J. J. Silber, and E. N. Durantini, J. Porphyr. Phthalocyanines, 2003, 7, 42. CrossRef
13. W. Anannarukan, S. Tantayanon, D. Zhang, E. A. Alemán, D. A. Modarelli, and F. W. Harris, Polymer, 2006, 47, 4936. CrossRef
14. J. S. Lindsey, ‘The Porphyrin Handbook’, Vol. 1, ed. by K. M. Kadish, K. M. Smith, and R. Guilard, Academic Press, San Diego, CA, 2000, pp. 45-118.
15. W. J. Kruper, T. A. Chamberlin, and M. Kochanny, J. Org. Chem., 1989, 54, 2753. CrossRef
16. S. Ostrowski and B. Łopuszyńska, Synth. Commun., 2003, 33, 4101. CrossRef
17. N. W. Smith and S. V. Dzyuba, ARKIVOC, 2010, vii, 10. CrossRef
18. B. J. Littler, Y. Ciringh, and J. S. Lindsey, J. Org. Chem., 1999, 64, 2864. CrossRef
19. C. Frixa, M. F. Mahon, A. S. Thompson, and M. D. Threadgill, Org. Biomol. Chem., 2003, 1, 306. CrossRef
20. Z. Xue, P. P. S. Lee, Y. Wang, D. W. J. Kwong, J. Li, J. H. Xin, W.-K. Wong, and K. K. L. Cheuk, Tetrahedron, 2011, 67, 6030. CrossRef
21. H.-L. Zhang, W.-M. Shi, and J. Wu, Heterocycles, 2005, 65, 3001. CrossRef
22. R. A. W. Johnstone, M. L. P. G. Nunes, M. M. Pereira, A. M. d'A. Rocha Gonsalves, and A. C. Serra, Heterocycles, 1996, 43, 1423. CrossRef
23. T. Lin, X. S. Shang, J. Adisoejoso, P. N. Liu, and N. Lin, J. Am. Chem. Soc., 2013, 135, 3576. CrossRef
24. A. C. Serra and A. M. d'A. Rocha Gonsalves, Tetrahedron Lett., 2010, 51, 4192. CrossRef
25. D. M. Wallace, S. H. Leung, M. O. Senge, and K. M. Smith, J. Org. Chem., 1993, 58, 7245. CrossRef
26. H. K. Hombrecher, G. Horter, and C. Arp, Tetrahedron, 1992, 48, 9451. CrossRef
27. T. Rohand, E. Dolusic, T. H. Ngo, W. Maes, and W. Dehaen, ARKIVOC, 2007, x, 307. CrossRef