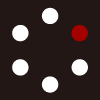
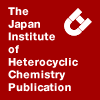
HETEROCYCLES
An International Journal for Reviews and Communications in Heterocyclic ChemistryWeb Edition ISSN: 1881-0942
Published online by The Japan Institute of Heterocyclic Chemistry
e-Journal
Full Text HTML
Received, 17th December, 2013, Accepted, 8th January, 2014, Published online, 15th January, 2014.
DOI: 10.3987/COM-13-12917
■ Synthesis of Quinolizidinone and Indolizidinone Using N-Acyliminium Ion Cyclization and a One-Pot Procedure for Preparation of Benzoquinolizidinone
Punlop Kuntiyong,* Nuanpan Piboonsrinakara, Pijitra Bunrod, Duangkamol Namborisut, Sunisa Akkarasamiyo, Poramate Songthammawat, Chitlada Hemmara, Artid Buaphan, and Boonsong Kongkathip
Department of Chemistry, Faculty of Science, Silpakorn University, 6 Rajamanka Nai Rd, Muang Nakhon Pathom 73000, Thailand
Abstract
Quinolizidinone, and indolizidinone systems were synthesized in a non-racemic form using cyclization of chiral N-acyliminium ions. The key reactive intermediates were prepared from the corresponding 2-arylethylamine or 3-butenylamine and L-glutamic acid or L-aspartic acid. The stereocenter bearing a dibenzylamino group was used for stereocontrol and resulted in diastereomeric products. A one-pot procedure for synthesis of benzoquinolizidinone was also developed. The dibenzylamino moiety was subsequently converted via Cope elimination to the corresponding cyclic enamide which is suitable as a synthetic precursor for a variety of quinolizidine alkaloids in enantiomerically pure form.INTRODUCTION
Quinolizidine and indolizidine are important structural features in many biological active alkaloids.1 Examples of these alkaloids are shown in Figure 1. Alkaloids from Corynanthe family with a wide range of biological activities such as potent antiviral hirsutine and cytotoxic corynantheidine and poisonous reserpine are representatives of indoloquinolizidine alkaloids.2 Examples of benzoquinolizidine alkaloids include α-glucosidase inhibiting schulzeines3 and the synthetic chorea drug tetrabenazine.4 Simple quinolizidine system can also be found, for example, in epiquinamide.5 Benzoindolizidine alkaloids are represented by antihyperglycemic jamtine and cytotoxic crispine A.6 Examples of simple indolizidine alkaloids are a family of hydroxylated indolizidine such as anti-glucosidase lentiginosine.7
Pictet-Spengler reaction is instrumental in syntheses of tetrahydroisoquinoline and tetrahydro-β-carboline. There are numerous reports of applications of Pictet-Spengler reaction and its variants in syntheses of these alkaloids in both racemic and enantiopure forms.8,9 Diastereoselective Pictet-Spengler reactions of tryptophan or phenylalanine derivatives with aldehyde can provide tetrahydro-β-carboline or tetrahydroisoquinoline, respectively, as mixture of diastereomers with certain level of diastereoselectivity.10 Asymmetric or enantioselective Pictet-Spengler reactions of achiral tryptamine derivatives or arylethylamines with aldehydes in the presence of chiral Lewis or Brönsted acid giving the products in moderate to high enantioselectivities have also been reported in recent years.11 N-Acyliminium ion cyclization, a more reactive variation of Pictet-Spengler reaction, has also been applied to synthesize numerous natural products.12 Herein we report applications of cyclization of chiral N-acyliminium ion prepared from L-glutamic acid or L-aspartic acid and arylethylamines in syntheses of quinolizidinone and indolizidinone systems in enantiopure form.
RESULTS AND DISCUSSION
In this approach, the amine starting materials were tryptamine (1), arylethylamine or 3-butenylamine. Tryptamine reacted with 1-benzyl N,N-dibenzyl-L-glutamate (2), prepared from benzylation of L-glutamic acid, in the presence of DCC and DMAP to give the corresponding amido-ester 3. The amido-ester was then treated with lithium aluminum hydride in THF to give imide 4. Carbonyl reduction at the less hindered site of the imide with DIBALH in toluene at -78 °C gave δ-hydroxy-δ-lactam 5 (Scheme 1).
A similar approach was applied with homoveratrylamine (6), however, amido-ester 7 obtained from its reaction with 1-benzyl N,N-dibenzyl-L-glutamate (2) was converted in one step to the corresponding δ-hydroxy-δ-lactam 8 via tandem imide formation/carbonyl reduction. Tethered alkene-amines can also be used as substrates for N-acyliminium ion cyclization. In the majority of reported cases, the cationic intermediate derived from the initial cyclization would be trapped with nucleophilic species in the form of conjugate base of the acid mediator or solvent to give the final product.13 Using the same reaction sequence as for the synthesis of hydroxylactam 8, 3-butenylamine-hydrochloride (9) reacted with 1-benzyl N,N-dibenzyl-L-glutamate (2) to give amido-ester 10 which was converted to δ-hydroxy-δ-lactam 11 after treatment with lithium aluminum hydride (Scheme 2).
Applying this approach to L-aspartic acid derivative led to γ-hydroxy-γ-lactam. Interestingly, homoveratrylamine (6) reacted with 4-benzyl N,N-dibenzyl-L-aspartate (12) to give directly imide 13, presumably via the corresponding amido-ester. The selective carbonyl reduction at the less hindered site was accomplished with DIBALH and the γ-hydroxy-γ-lactam 14 was obtained in excellent yield (Scheme 3). In the meantime, 3-butenylamine hydrochloride reacted with 4-benzyl N,N-dibenzyl-L-aspartate (12) to give amido-ester 15 which was converted to hydroxylactam 16 upon treatment with LAH.
Once the hydroxylactams are on hands, the N-acyliminium ion cyclization was carried out. In general, the cyclized products were obtained in good yields upon treatment of the hydroxylactam with TMSOTf in dichloromethane at 0 °C under argon. The results are summarized in Table 1. Hydroxylactams tethered with butenyl moiety underwent cyclization to give quinolizidinone and indolizidinone in an aza-Prins mode without detection of product derived from trapping of carbocationic intermediate with fluoride ion (entries 3 and 5). The cyclized products were obtained as mixture of two diastereomers at the newly generated stereocenter. The diastereoselectivities are low to moderate with the product of α-H configuration at the stereocenter as the major diastereomer in all cases, resulting from addition of the nucleophile from the same face as the dibenzylamino group.13 Considering that these reactions tend to give kinetically controlled products,12 the results suggest of stabilizing effect of the dibenzylamino group i.e. π-π interaction directing the cyclization to the re-face of N-acyliminium ion,14 rather than destabilizing steric effect (Figure 2).
A one-pot procedure for synthesis of dibenzylaminobenzoquinolizidinone was also established. In this regard, amido-ester 7 was treated with LAH in THF to give the hydroxylactam. The unreacted LAH was quenched with methanol and the solvent was evaporated under a stream of argon. Dry dichloromethane was added and to this resulting solution was added TMSOTf under argon at 0 °C. Even though the yield of the one-pot procedure is lower than the overall yield of the three-step process, it offers a practical procedure for facile preparation of benzoquinolizidinone system in a non-racemic form (Scheme 4).
Even though the lack of high diastereoselectivities seems unattractive at the first glance, in cases where families of natural products are present in nature as diastereomers, this provides an opportunity to synthesize several members of the families in the same time. We have reported the use of N-acyliminium ion cyclization in a formal synthesis of diastereomeric schulzeines B and C.15 We envision that the two diastereomers of indoloquinolizidinone 17α and 17β can be used as precursors for synthesis of corynantheidine and hirsutine, respectively. The dibenzylamino group, derived from the amino acid, exerted a certain degree of stereocontrol and provided the quinolizidinone system in non-racemic form. Once the two diastereomers were chromatographically separated, functionalization by Cope elimination gave the corresponding cyclic enamide for further synthetic steps toward benzoquinolizidine and indoloquinolizidine alkaloids. The resulting functionality is susceptible for Michael reaction, Diels-Alder reaction, lactam reduction, and other reactions of the C=C double bond. In this regard, Dibenzylaminoindoloquinolizidinone 17β was converted to enamide 23 (via N-Boc-indoloquinolizidinone 22) using an N-protection/Cope elimination sequence. Similarly, dibenzylaminobenzoquinolizidinone 18 underwent Cope elimination upon treatment with m-CPBA in CHCl3 to give tricyclic enamide 24 in good yield (Scheme 5).
In summary, we report a facile synthesis of quinolizidinone and indolizidinone systems using N-acyliminium ion cyclization. This procedure offers an alternative approach to the tryptophan’s diastereoselective Pictet-Spengler reaction and enantioselective variation using chiral catalyst. The diastereomeric ratios were low to moderate and provided two diastereomers of the cyclized products which could be used for synthesis of families of natural products that exist in more than one diastereomers. A one-pot procedure for synthesis of dibenzylaminobenzoquinolizidinone was also established. We envision that the tetracyclic enamide 23 and its diastereomer could lead to Corynanthe alkaloids via malonate Michael addition and yohimbane alkaloids via Diels-Alder reaction. The tricyclic enamide 24 could lead to tetrabenazine using hetero-Michael addition, whereas the benzoindolizidinone 20 contains the tricyclic core of crispine A and jamtine. Synthetic studies of these alkaloids using this approach are being carried out in our laboratory. The results of the conversions of these cyclized products toward quinolizidine alkaloids will be reported in due course.
EXPERIMENTAL
Starting materials and reagents were obtained from commercial sources and were used without further purification. Solvents were dried by distillation from the appropriate drying reagents immediately prior to use. Tetrahydrofuran and ether were distilled from sodium and benzophenone under argon. Toluene, triethylamine, and dichloromethane were distilled from calcium hydride under arg
on. Moisture- and air-sensitive reactions were carried out under an atmosphere of argon. Reaction flasks and glassware were oven-dried at 105 °C overnight. Unless otherwise stated, concentration was performed under reduced pressure. Analytical thin-layer chromatography (TLC) was conducted using Fluka precoated TLC plates (0.2 mm layer thickness of silica gel 60 F-254). Compounds were visualized by ultraviolet light and/or by heating the plate after dipping in a 1% solution of vanillin in 0.1M sulfuric acid in EtOH. Flash chromatography was carried out using Scientific Absorbents Inc. silica gel (40 μm particle size). Optical rotations were measured with a Perkin-Elmer 243 polarimeter at ambient temperature using a 0.9998 dm cell with 1 mL capacity. Infrared (IR) spectra were recorded on a Nicolet 5DXB FT-IR spectrometer. Proton and carbon nuclear magnetic resonance (NMR) spectra were obtained using a Bruker AC-300 spectrometer.
Amido-ester 3. A solution of 1-benzyl N,N-dibenzyl-L-glutamate (332 mg, 0.796 mmol), tryptamine (255 mg, 1.59 mmol), DCC (328 mg, 1.59 mmol) and DMAP (10.0 mg, 0.0818 mmol) in CH2Cl2 (10 mL) was stirred at room temperature for 4 h. The mixture was filtered and the filtrate concentrated under reduced pressure. The crude product was purified by flash chromatography (silica gel, 2:1 hexane/ EtOAc) to provide amido-ester 3 (352 mg, 79%) as a colorless oil; Rf (2:1 hexane/EtOAc) 0.28; δH (300 MHz, CDCl3) 7.60 (d, J = 7.1 Hz, 1H), 7.50-7.10 (m, 18H), 6.90 (s, 1H), 5.27 (d, J = 12.2 Hz, 1H), 5.17 (d, J = 12.2 Hz, 1H), 5.12 (brs, 1H), 3.84 (d, J = 13.8 Hz, 2H), 3.53 (d, J = 13.8 Hz, 2H), 3.52-3.50 (m, 1H), 3.41-3.28 (m, 2H), 2.83-2.91 (m, 2H), 2.30-2.15 (m, 1H), 2.15-1.95 (m, 3H), 1.90-1.75 (m, 1H); δC (75 MHz, CDCl3) 172.2, 172.2, 139.4, 136.4, 136.0, 129.0, 128.7, 128.6, 128.5, 128.3, 127.3, 127.1, 122.2, 122.0, 119.4, 118.7, 113.0, 111.2, 66.2, 60.3, 54.5, 39.7, 33.2, 25.4, 25.2; [α]D25 –54.3 (c 1.4, CH2Cl2); νmax (film) 3419, 3298, 3060, 3031, 2938, 2850, 1726, 1655, 1523, 1494, 1455, 1212 cm-1; ESI-HRMS calculated for C36H37N3O3Na [M+Na]+ 582.2732, found 582.2712.
Imide 4. To a solution of amido-ester 3 (400 mg, 0.714 mmol) in THF (20.0 mL) was added LiAlH4 (41.0 mg, 1.07 mmol) in one portion at 0 °C. The mixture was stirred for 15 min and sat. aq. NaHCO3 (10.0 mL) was added drop-wise. The mixture was extracted with EtOAc (3 x 20 mL). The combined organic layers were dried over anhyd Na2SO4, filtered and concentrated under reduced pressure. The crude product was purified by flash chromatography (silica gel, 4:1 hexane/EtOAc) to give imide 4 (210 mg, 65%) as colorless oil; Rf (2:1 hexane/EtOAc) 0.63; δH (300 MHz, CDCl3) 7.96 (brs, 1H), 7.72 (d, J = 7.2 Hz, 1H), 7.48-7.19 (m, 11H), 7.14 (td, J = 7.0, 1.4 Hz, 1H), 7.10 (td, J = 7.0, 1.4 Hz, 1H), 7.02 (d, J = 2.1 Hz, 1H), 4.13 (ddd, J = 12.7, 9.0, 7.4 Hz, 1H), 3.99 (ddd, J = 12.7, 8.7, 5.4 Hz, 1H), 3.84 (d, J = 14.0 Hz, 2H), 3.54 (d, J = 14.0 Hz, 2H), 3.39 (dd, J = 12.1, 5.6 Hz, 1H), 3.11-2.91 (m, 2H), 2.73 (ddd, J = 17.3, 4.0, 2.9 Hz, 1H), 2.35 (ddd, J = 17.4, 13.3, 5.8 Hz, 1H), 1.93 (m, 2H); δC (75 MHz, CDCl3) 172.2, 171.0, 138.7, 135.1, 127.5, 127.3, 126.6, 126.1, 121.3, 120.9, 118.4, 117.9, 111.6, 110.1, 58.2, 53.8, 39.6, 31.2, 22.5, 21.4; [α]D25 –45.7 (c 1.1, CH2Cl2); νmax (film) 3058, 3029, 2925, 2854, 1723, 1671, 1494, 1455 cm-1; HRMS (ESI) calculated for C29H30N3O2 [M+H]+ 452.2338, found 452.2331.
Hydroxylactam 5. To a solution of imide 4 (191 mg, 0.423 mmol) in toluene (10 mL) was added DIBALH (0.63 mL, 1.0M in toluene, 0.630 mmol) via syringe at -78 °C. The mixture was stirred for 45 min and sat. aq. potassium sodium tartrate (5 mL) was added drop-wise. The mixture was stirred at room temperature for 45 min and extracted with EtOAc (3 x 20 mL). The combined organic layers were dried over anhyd Na2SO4, filtered and concentrated under reduced pressure. The crude product was purified by flash chromatography (silica gel, 2:1 hexane/EtOAc) to give hydroxylactam 5 (188 mg, 98%) as a colorless oil; Rf (1:1 hexane/EtOAc) 0.60; δH (300 MHz, CDCl3) 7.97 (brs, 1H), 7.67 (d, J = 7.8 Hz, 1H), 7.50-7.13 (m, 12H), 7.13-7.03 (m, 1H), 7.00 (s, 1H), 4.67 (t, J = 7.2 Hz, 1H), 4.00 (d, J = 14.0 Hz, 2H), 3.94-3.70 (m, 3H), 3.64 (d, J = 14.0 Hz, 2H), 3.38-3.22 (m, 1H), 3.17-3.02 (m, 2H), 2.20-2.00 (m, 2H), 2.00-1.71 (m, 2H); δC (75 MHz, CDCl3) 171.7, 140.5, 136.2, 128.6, 128.5, 128.2, 126.8, 122.2, 122.1, 119.5, 118.9, 113.6, 111.2, 80.7, 58.1, 55.5, 44.0, 31.4, 23.9, 23.2; [α]D25 –11.4 (c 0.8, CHCl3); νmax (film) 3300, 3060, 3025, 2928, 2853, 1723, 1671, 1455 cm-1; ESI-HRMS calculated for C29H32N3O2 [M+H]+ 454.2494, found 454.2494.
Amido-ester 7. A solution of 1-benzyl N,N-dibenzyl-L-glutamate (224 mg, 0.536 mmol), homoveratrylamine (0.18 mL, 1.07 mmol), DCC (221 mg, 1.07 mmol) and DMAP (6.1 mg, 0.0499 mmol) in CH2Cl2 (10 mL) was stirred at room temperature for 4 h. The mixture was filtered and the filtrate concentrated under reduced pressure. The crude product was purified by flash chromatography (silica gel, 2:1 hexane/EtOAc) to provide amido-ester 7 (252 mg, 81%) as a colorless oil; Rf (1:1 hexane/ EtOAc) 0.59; δH (300 MHz, CDCl3) 7.60-7.10 (m, 15H), 6.81 (d, J = 8.5 Hz, 1H), 6.65-6.75 (m, 2H), 5.29 (d, J = 12.1 Hz, 1H), 5.19 (s, 1H), 5.18 (d, J = 12.1 Hz, 1H), 3.89 (d, J = 13.7 Hz, 2H), 3.88 (s, 3H), 3.87 (s, 3H), 3.55 (d, J=13.7 Hz, 2H), 3.47-3.31 (m, 2H), 3.25 (ddd, J= 20.1, 13.1, 6.7 Hz, 1H), 2.65 (t, J = 7.1 Hz, 2H), 2.32-2.18 (m, 1H), 2.18-1.95 (m, 3H); δC (75 MHz, CDCl3) 172.2, 172.2, 149.0, 147.7, 139.4, 135.9, 131.4, 129.0, 128.6, 128.5, 128.4, 128.3, 127.1, 120.6, 111.9, 111.3, 66.2, 60.3, 55.9, 54.5, 40.8, 35.2, 33.2, 25.4, 14.2; [α]D25 –56.6 (c 1.1, CH2Cl2); νmax (film) 3418, 3057, 3031, 2937, 2837, 1727, 1630, 1591, 1455, 1419, 1371, 1237, 1156 cm-1; ESI-HRMS calculated for C36H40N2O5Na [M+Na]+ 603.2835, found 603.2814.
Hydroxylactam 8. To a solution of amido-ester 7 (334 mg, 0.575 mmol) in THF (10 mL) was added LiAlH4 (26.2 mg, 0.690 mmol) in one portion at 0 °C. The mixture was stirred for 15 min and sat. aq. NaHCO3 (5 mL) was added drop-wise. The mixture was extracted with EtOAc (3 x 20 mL). The combined organic layers were dried over anhyd Na2SO4, filtered and concentrated under reduced pressure. The crude product was purified by flash chromatography (silica gel, 2:1 hexane/EtOAc) to give hydroxylactam 8 (202 mg, 74%) as a colorless oil; Rf (1:1 hexane/EtOAc) 0.40; δH (300 MHz, CDCl3) 7.45-7.03 (m, 10H), 6.75 (brs, 2H), 6.70 (s, 1H), 4.59 (brs, 1H), 4.03 (d, J = 14.1 Hz, 2H), 3.80 (s, 3H), 3.75 (s, 3H), 3.73 (d, J = 14.1 Hz, 2H), 3.75-3.72 (m, 1H), 3.65-3.40 (m, 2H), 3.35-3.20 (m, 1H), 2.92-2.82 (m, 2H), 2.30-2.10 (m, 1H), 1.91-1.77 (m, 2H), 1.52-1.72 (m, 1H); δC (75 MHz, CDCl3) 171.8, 149.0, 147.7, 140.2, 131.8, 128.9, 128.2, 126.9, 120.8, 112.1, 111.3, 80.6, 59.2, 55.9, 55.8, 55.5, 48.7, 34.1, 29.5, 21.5; [α]D25 –11.6 (c 1.8, CHCl3); νmax (film) 3373, 3054, 2937, 2838, 1638, 1515, 1455, 1420, 1237 cm-1; ESI-HRMS calculated for C29H35N2O4 [M+H]+ 475.2597, found 475.2590.
Amido-ester 10. A solution of 1-benzyl N,N-dibenzyl-L-glutamate (170 mg, 0.407 mmol), 3-butenylamine hydrochloride (87.6 mg, 0.814 mmol), DCC (168 mg, 0.814 mmol) and DMAP (4.9 mg, 0.0401 mmol) in CH2Cl2 (10 mL) was stirred at room temperature for 4 h. The mixture was filtered and the filtrate concentrated under reduced pressure. The crude product was purified by flash chromatography (silica gel, 2:1 hexane/EtOAc) to provide amido-ester 10 (180 mg, 94%) as a colorless oil; Rf (1:1 hexane/EtOAc) 0.59; δH (300 MHz, CDCl3) 7.25-7.05 (m, 15H), 5.59 (dddd, J = 16.6, 9.5, 6.8, 6.8 Hz, 1H), 5.17 (d, J = 12.2 Hz, 1H), 5.10 (m, 1H), 5.07 (d, J = 12.2 Hz, 1H) 4.93 (d, J = 9.5 Hz, 1H), 4.92 (d, J = 16.6 Hz, 1H), 3.79 (d, J = 13.7 Hz, 2H), 3.44 (d, J = 13.7 Hz, 2H), 3.24 (dd, J = 7.7, 6.2 Hz, 1H), 3.09 (ddd, J = 19.9, 13.3, 6.7 Hz, 1H), 2.97 (ddd, J = 19.9, 12.8, 6.7 Hz, 1H), 2.30-1.80 (m, 6H); δC (75 MHz, CDCl3) 172.3, 172.2, 139.4, 135.9, 135.3, 129.0, 128.7, 128.6, 128.4, 128.3, 127.2, 117.0, 66.2, 60.3, 54.6, 38.4, 33.7, 33.1, 25.4; [α]D25 –72.1 (c 2.1, CH2Cl2); νmax (film) 3434, 3084, 3055, 3031, 2980, 2940, 2847, 1728, 1663, 1519, 1495, 1456, 1372, 1211 cm-1; ESI-HRMS calculated for C30H35N2O3 [M+H]+ 471.2648, found 471.2636.
Hydroxylactam 11. To a solution of amido-ester 10 (217 mg, 0.461 mmol) in THF (10 mL) was added LiAlH4 (21.0 mg, 0.553 mmol) in one portion at 0 °C. The mixture was stirred for 15 min and sat. aq. NaHCO3 (5 mL) was added drop-wise. The mixture was extracted with EtOAc (3 x 10 mL). The combined organic layers were dried over anhyd Na2SO4, filtered and concentrated under reduced pressure. The crude product was purified by flash chromatography (silica gel, 2:1 hexane/EtOAc) to give hydroxylactam 11 (153 mg, 91%) as a colorless oil; Rf (1:1 hexane/EtOAc) 0.71; δH (300 MHz, CDCl3) 7.47 (d, J = 7.1 Hz, 4H), 7.33 (t, J = 7.1 Hz, 4H), 7.24 (t, J =7.1 Hz, 2H), 5.83 (dddd, J = 18.1, 10.1, 6.9, 6.9 Hz, 1H), 5.13-5.03 (buried m, 1H), 5.10 (dd, J = 18.1, 1.5 Hz, 1H), 5.04 (d, J = 10.1 Hz, 1H), 4.84 (dd, J = 8.0, 5.1 Hz, 1H), 4.03 (d, J = 14.1 Hz, 2H), 3.81 (dd, J = 14.3, 7.1 Hz, 1H), 3.72 (d, J = 14.1 Hz, 2H), 3.52-3.38 (m, 1H), 3.28 (dd, J = 10.9, 5.9 Hz, 1H), 2.51-2.27 (m, 2H), 2.24 (dq, J = 13.5, 5.0 Hz, 1H), 2.03-1.86 (m, 1H), 1.75 (ddd, J = 24.0, 12.3, 3.4 Hz, 1H), 1.60-1.42 (m, 1H); δC (75 MHz, CDCl3) 171.7, 140.2, 135.9, 128.8, 128.2, 126.9, 117.0, 80.1, 59.1, 55.5, 45.7, 33.8, 29.5, 21.6; [α]D25
–20.5 (c 1.7, CHCl3); νmax (film) 3333, 2923, 2853, 1642, 1493, 1454, 1363, 1261, 1027, 739, 698 cm-1; ESI-HRMS calculated for C23H28N2O2Na [M+Na]+ 387.2048, found 387.2048.
Imide 13. A solution of 4-benzyl N,N-dibenzyl-L-aspartate 12 (397 mg, 0.984 mmol), homoveratrylamine (0.330 mL, 1.97 mmol), DCC (406 mg, 1.97 mmol) and DMAP (12.2 mg, 0.0999 mmol) in CH2Cl2 (10 mL) was stirred at room temperature for 4 h. The mixture was filtered and the filtrate concentrated under reduced pressure. The crude product was purified by flash chromatography (silica gel, 4:1 hexane/EtOAc) to provide imide 13 (325 mg, 72%) as colorless oil; Rf (2:1 hexane/EtOAc) 0.55; δH (300 MHz, CDCl3) 7.46-7.16 (m, 10H), 6.81 (s, 1H), 6.62 (d, J = 8.2 Hz, 1H), 6.65 (d, J = 8.2 Hz, 1H), 3.88-3.70 (buried m, 3H), 3.85 (s, 3H), 3.71 (s, 3H), 3.62 (d, J = 13.5 Hz, 2H), 3.46 (d, J = 13.5 Hz, 2H), 2.87 (t, J = 7.6 Hz, 2H), 2.70 (dd, J = 18.4, 9.0 Hz, 1H), 2.53 (dd, J = 18.4, 5.2 Hz, 1H); δC (75 MHz, CDCl3) 177.0, 175.1, 148.9, 147.8, 138.1, 129.8, 128.8, 128.5, 127.5, 121.0, 111.9, 111.0, 57.2, 55.9, 55.7, 54.5, 39.4, 32.8, 32.0; [α]D25 –10.2 (c 0.9, CHCl3); νmax (film) 2930, 1702, 1516, 1264, 1115, 699 cm-1; ESI-HRMS calculated for C28H30N2O4Na [M+Na]+ 481.2103, found 481.2117.
Hydroxylactam 14. To a solution of imide 13 (321 mg, 0.699 mmol) in toluene (10 mL) was added DIBALH (1.05 mL, 1M in toluene, 1.05 mmol) via syringe at –78 °C. The mixture was stirred for 45 min and sat. aq. potassium sodium tartrate (5 mL) was added drop-wise. The mixture was stirred at room temperature for 45 min and extracted with EtOAc (3 x 20 mL). The combined organic layers were dried over anhyd Na2SO4, filtered and concentrated under reduced pressure. The crude product was purified by flash chromatography (silica gel, 2:1 hexane/EtOAc) to give hydroxylactam 14 (306 mg, 95%) as a colorless oil; Rf (2:1 hexane/EtOAc) 0.18; δH (300 MHz, CDCl3) 7.50-7.26 (m, 10H), 6.71 (s, 3H), 4.75 (brs, 1H), 3.89 (d, J = 13.7 Hz, 2H), 3.80 (s, 3H), 3.77 (s, 3H), 3.68 (d, J = 14.2 Hz, 2H), 3.66-3.57 (m, 1H), 3.55-3.39 (m, 2H), 3.01 (brs, 1H), 282 (m, 2H), 2.43 (ddd, J = 14.1, 9.0, 7.0 Hz, 1H), 1.70 (ddd, J = 12.6, 7.3, 4.7 Hz, 1H); δC (75 MHz, CDCl3) 173.1, 148.9, 147.6, 139.3, 131.4, 128.8, 128.3, 127.1, 120.7, 112.0, 111.3, 80.5, 58.9, 55.8, 55.8, 54.7, 41.7, 33.4, 32.7; [α]D25 +3.8 (c 1.3, CHCl3); νmax (film) 3392, 2929, 1672, 1515, 1453, 1261, 1028, 699 cm-1; ESI-HRMS calculated for C28H32N2O4Na [M+Na]+ 483.2260, found 483.2252.
Amido-ester 15. A solution of 1-benzyl N,N-dibenzyl-L-aspartate 12 (224 mg, 0.555 mmol), 3-butenylamine hydrochloride (0.19 mL, 1.10 mmol), DCC (227 mg, 1.10 mmol) and DMAP (6.8 mg, 0.0555 mmol) in CH2Cl2 (10 mL) was stirred at room temperature for 4 h. The mixture was filtered and the filtrate concentrated under reduced pressure. The crude product was purified by flash chromatography (silica gel, 2:1 hexane/EtOAc) to provide amido-ester 15 (197 mg, 78%) as a colorless oil; Rf (2:1 hexane/EtOAc) 0.43; δH (300 MHz, CDCl3) 7.50-7.15 (m, 15H), 7.08 (t, J = 5.5 Hz, 1H), 5.78 (ddd, J = 17.4, 10.7, 6.8, 6.8 Hz, 1H), 5.24 (d, J = 12.4 Hz, 1H), 5.16 (d, J = 12.4 Hz, 1H), 5.12 (d, J = 10.1 Hz, 1H), 5.11 (d, J = 17.0 Hz, 1H), 3.95 (dd, J = 8.9, 4.5 Hz, 1H), 3.75 (d, J = 13.4 Hz, 2H), 3.41 (d, J = 13.4 Hz, 2H), 3.35 (t, J = 6.4 Hz, 2H), 3.02 (dd, J = 15.5, 9.0 Hz, 1H), 2.72 (dd, J = 15.5, 4.4 Hz, 1H), 2.27 (dt, J = 12.2, 6.4 Hz, 2H); δC (75 MHz, CDCl3) 172.2, 171.9, 138.3, 135.9, 135.4, 128.8, 128.6, 128.5, 128.3, 128.2, 127.6, 117.4, 66.6, 59.5, 54.7, 38.32, 33.7, 29.2; [α]D25 –1.96 (c 1.6, CH2Cl2); νmax (film) 3389, 3030, 2844, 1742, 1682, 1515, 1455, 1356, 1167, 994, 917, 747, 698 cm-1; ESI-HRMS calculated for C29H32N2O3 [M+H]+ 457.2491, found 457.2495.
Hydroxylactam 16. To a solution of amido-ester 15 (200 mg, 0.438 mmol) in THF (10 mL) was added LiAlH4 (24.9 mg, 0.657 mmol) in one portion at 0 °C. The mixture was stirred for 15 min and sat. aq. NaHCO3 (5 mL) was added drop-wise. The mixture was extracted with EtOAc (3 x 20 mL). The combined organic layers were dried over anhyd Na2SO4, filtered and concentrated under reduced pressure. The crude product was purified by flash chromatography (silica gel, 2:1 hexane/EtOAc) to give hydroxylactam 16 (101 mg, 66%) as a colorless oil; Rf (2:1 hexane/EtOAc) 0.20; δH (300 MHz, CDCl3) 7.65-7.10 (m, 10H), 5.79 (dddd, J = 17.0, 10.1, 6.8, 6.8 Hz, 1H), 5.13-5.23-4.94 (m, 3H), 3.95 (d, J = 13.7 Hz, 2H), 3.75 (d, J = 13.7 Hz, 2H), 3.66-3.47 (m, 2H),3.47-3.25 (m, 1H), 2.55 (ddd, J = 14.0, 9.5, 6.8 Hz, 1H), 2.41-2.28 (m, 2H), 1.78 (ddd, J = 14.0, 7.6, 4.5 Hz, 1H); δC (75 MHz, CDCl3) 172.9, 139.4, 135.5, 128.9, 128.3, 127.1, 117.0, 80.4, 59.0, 54.9, 39.4, 33.4, 32.1; [α]D25 –0.06 (c 1.7, CH2Cl2); νmax (film) 3331 (broad), 2851, 1668, 1454, 1076, 916, 745, 699 cm-1; ESI-HRMS calculated for C22H26N2O2 [M+H]+ 351.2073, found 351.2073.
Dibenzylaminoinodoloquinolizidinone 17. To a solution of hydroxylactam 5 (204 mg, 0.450 mmol) in CH2Cl2 (10 mL) at 0 °C was added TMSOTf (0.122 mL, 0.675 mmol) via syringe. The mixture was stirred at this temperature for 3 h. CH2Cl2 (5 mL) and sat. aq. NaHCO3 (5 mL) were added and phases were separated. The aqueous phase was extracted with CH2Cl2 (3 x 10 mL). The combined organic layers were dried over anhyd Na2SO4, filtered and concentrated under reduced pressure. The crude product was purified by flash chromatography (silica gel, 4:1 hexane/EtOAc) to provide dibenzylaminoindoloquinolizidinone 17α (102 mg, 52%) and 17β (43.0 mg, 22%) as colorless oil; 17α Rf (4:1 hexane/EtOAc) 0.27; δH (300 MHz, CDCl3) 7.85 (brs, 1H), 7.50 (d, J = 7.20 Hz, 1H), 7.42 (m, 13H), 5.03 (dd, J = 12.0, 3.2 Hz, 1H), 4.75 (brs, 1H), 4.04 (d, J = 13.9 Hz, 2H), 3.65 (d, J = 13.9 Hz, 2H), 3.41 (dd, J = 10.1, 6.8 Hz, 1H), 3.07-2.82 (m, 2H), 2.73 (d, J = 12.0 Hz, 1H), 2.30-2.10 (m, 2H), 2.00-1.77 (m, 2H); δC (75 MHz, CDCl3) 171.4, 140.6, 136.0, 132.9, 128.6, 128.6, 128.1, 127.3, 126.7, 122.2, 119.9, 118.3, 111.0, 58.4, 55.7, 53.7, 42.0, 26.0, 24.3, 21.2; [α]D25 -43.3 (c 1.5, CHCl3); νmax (film) 3319, 2925, 2852, 1728, 1624, 1454, 1303 cm-1; ESI-HRMS calculated for C29H30N3O [M+H]+ 436.2389, found 436.2409.
Dibenzylaminoinodoloquinolizidinone 17β.
Rf (4:1 hexane/EtOAc) 0.42; δH (300 MHz, CDCl3) 7.82 (brs, 1H), 7.60-7.02 (m, 14H), 5.22 (dt, J = 16.0, 8.0 Hz, 1H), 4.70 (d, J = 8.0 Hz, 1H), 4.15 (d, J = 14.0 Hz, 2H), 3.83 (d, J = 14.0 Hz, 2H), 3.39 (dd, J = 11.6, 6.3 Hz, 1H), 2.94-2.70 (m, 3H), 2.40 (dq, J = 13.2, 3.5 Hz, 1H), 2.20-2.02 (m, 1H), 2.06-1.91 (m, 1H), 1.80-1.60 (m, 1H); δC (75MHz, CDCl3) 170.6, 140.5, 136.2, 133.2, 128.7, 128.2, 128.7, 126.9, 122.2, 119.9, 118.4, 110.9, 109.5, 58.6, 55.7, 54.1, 39.6, 29.7, 28.5, 26.0, 21.0; [α]D25 34.1 (c 0.5, CHCl3); νmax (film) 3284, 3026, 2921, 1617, 1493, 1428, 1352, 1302; ESI-HRMS calculated for C29H30N3O [M+H]+ 436.2389, found 436.2397.
Dibenzylaminobenzoquinolizidinone 18. To a solution of hydroxylactam 8 (198 mg, 0.417 mmol) in CH2Cl2 (7 mL) at 0 °C was added TMSOTf (0.151 mL, 0.834 mmol) via syringe. The mixture was stirred at this temperature for 3 h. CH2Cl2 (5 mL) and sat. aq. NaHCO3 (5 mL) were added and phases were separated. The aqueous phase was extracted with CH2Cl2 (3 x 10 mL). The combined organic layers were dried over anhyd Na2SO4, filtered and concentrated under reduced pressure. The crude product was purified by flash chromatography (silica gel, 4:1 hexane/EtOAc) to provide dibenzylaminobenzoquinolizidinone 18 (mixture of two diastereomers in approximately 5.7:1 ratio as determined by 1H NMR, 181 mg, 95%) as colorless oil.
One-pot procedure for synthesis of dibenzylaminobenzoquinolizidinone 18 from amido-ester 8
To a solution of amido-ester 7 (264 mg, 0.455 mmol) in THF (10 mL) was added LiAlH4 (25.9 mg, 0.682 mmol) in one portion at 0 °C. The mixture was stirred for 15 min and MeOH (2.0 mL) was added drop-wise. The solvent was evaporated under argon and CH2Cl2 (5 mL) was added to the residue. To the resulting solution at 0 °C was added TMSOTf (0.247 mL, 1.365 mmol) via syringe. The mixture was stirred at this temperature for 3 h. CH2Cl2 (5.0 mL) and sat. aq. NaHCO3 (5 mL) were added and phases were separated. The aqueous phase was extracted with CH2Cl2 (3 x 10 mL). The combined organic layers were dried over anhyd Na2SO4, filtered and concentrated under reduced pressure. The crude product was purified by flash chromatography (silica gel, 2:1 hexane/EtOAc) to provide benzoindolizidinone 18 (96 mg, 46%) as a colorless oil; Rf (2:1 hexane/EtOAc) 0.52; δH (300 MHz, CDCl3) 7.70-7.15 (m, 10H), 6.64 (s, 1H), 6.62 (s, 1H), 4.82 (dd, J = 9.7, 1.8 Hz, 1H), 4.49 (t, J = 5.5 Hz, 1H), 4.12 (d, J = 14.2 Hz, 2H), 3.87 (s, 3H), 3.86 (s, 3H), 3.75 (d, J = 14.2 Hz, 2H), 3.46 (dd, J = 10.0, 6.6 Hz, 1H), 3.02-2.76 (m, 2H), 2.67 (d, J = 12.0 Hz, 1H), 2.24-1.80 (m, 4H); δC (75 MHz, CDCl3) 171.5, 147.9, 147.7, 140.7, 128.6, 128.5, 128.2, 127.8, 126.8, 111.8, 108.1, 57.7, 56.1, 55.9, 55.4, 55.1, 40.4, 28.7, 28.5, 23.9; [α]D25
–54.2 (c 1.6, CHCl3); νmax (film) 3054, 2986, 1638, 1513, 1421, 1359, 1224 cm-1; ESI-HRMS calculated for C29H33N2O3 [M+H]+ 457.2491, found 457.2494.
Dibenzylaminoquinolizidinone 19. To a solution of hydroxylactam 11 (152 mg, 0.419 mmol) in CH2Cl2 (7 mL) at 0 °C was added TMSOTf (0.152 mL, 0.838 mmol) via syringe. The mixture was stirred at this temperature for 3 h. CH2Cl2 (5 mL) and sat. aq. NaHCO3 (5 mL) were added and phases were separated. The aqueous phase was extracted with CH2Cl2 (3 x 10 mL). The combined organic layers were dried over anhyd Na2SO4, filtered and concentrated under reduced pressure. The crude product was purified by flash chromatography (silica gel, 4:1 hexane/EtOAc) to provide dibenzylaminoquinolizidinone 19 (mixture of two diastereomers in approximately 3.0:1 ratio as determined by 1H NMR, 132 mg, 91%) as a colorless oil; Rf (4:1 hexane/EtOAc) 0.28; δH (300 MHz, CDCl3) 7.47 (d, J = 7.1 Hz, 4H), 7.33 (t, J = 7.1 Hz, 4H), 7.24 (t, J = 7.1 Hz, 2H), 5.81-5.64 (m, 2H), 4.89 (d, J = 18.0 Hz, 1H), 4.06 (d, J = 14.1 Hz, 2H), 3.74 (d, J = 14.1 Hz, 2H), 3.55-3.43 (m, 1H), 3.37 (d, J = 18.0 Hz, 1H), 3.31-3.23 (m, 1H), 2.30 (t, J = 13.2 Hz, 1H), 2.10-1.50 (m, 5H); δC (75 MHz, CDCl3) 170.4, 140.7, 128.8, 128.2, 126.7, 125.1, 124.8, 58.7, 55.7, 52.1, 42.5, 32.0, 26.2, 23.1; [α]D25 –1.4 (c 2.3, CHCl3); νmax (film) 3028, 2928, 1634, 1493, 1452, 739, 698 cm-1; ESI-HRMS calculated for C23H26N2ONa [M+Na]+ 369.1943, found 369.1950.
Benzoindolizidinone 20. To a solution of hydroxylactam 14 (219 mg, 0.602 mmol) in CH2Cl2 (7 mL) at 0 °C was added TMSOTf (0.218 mL, 1.204 mmol) via syringe. The mixture was stirred at this temperature for 3 h. CH2Cl2 (5.0 mL) and sat. aq. NaHCO3 (5 mL) were added and phases were separated. The aqueous phase was extracted with CH2Cl2 (3 x 10 mL). The combined organic layers were dried over anhyd Na2SO4, filtered and concentrated under reduced pressure. The crude product was purified by flash chromatography (silica gel, 2:1 hexane/EtOAc) to provide benzoindolizidinone 20 (mixture of two diastereomers in approximately 2.8:1 ratio as determined by 1H NMR, 192 mg, 72%) as a colorless oil; Rf (2:1 hexane/EtOAc) 0.36; δH (300 MHz, CDCl3) 7.47 (d, J = 7.1 Hz, 4H), 7.33 (t, J = 7.1 Hz, 4H), 7.24 (t, J = 7.1 Hz, 2H), 6.57 (s, 1H), 6.53 (s, 1H), 4.74 (t, J = 7.3 Hz, 1H), 4.38 (dd, J = 12.0, 6.2 Hz, 1H), 3.94 (d, J = 13.7 Hz, 2H), 3.87 (s, 3H), 3.85 (s, 3H), 3.76 (d, J = 13.7 Hz, 2H), 3.68 (dd, J = 10.5, 4.0 Hz, 1H), 3.25 (td, J = 11.8, 4.6 Hz, 1H), 2.94-2.78 (m, 1H), 2.68-2.53 (m, 2H), 2.14 (ddd, J = 13.9, 10.4, 6.1 Hz, 1H); δC (75MHz, CDCl3) 172.4, 148.1, 147.9, 139.4, 129.8, 129.0, 128.2, 127.0, 125.4, 111.6, 107.9, 59.9, 55.9, 54.9, 37.4, 33.9, 31.3, 28.1, 25.0; [α]D25 +27.2 (c 3.0, CHCl3); νmax (film) 3331, 3060, 2931, 2850, 1684, 1494, 1455, 1227, 1117, 735, 699 cm-1; ESI-HRMS calculated for C28H30N2O3Na [M+Na]+ 465.2154, found 465.2166.
Indolizidinone 21. To a solution of hydroxylactam 16 (80 mg, 0.228 mmol) in CH2Cl2 (5 mL) at 0 °C was added TMSOTf (0.124 mL, 0.684 mmol) via syringe. The mixture was stirred at this temperature for 3 h. CH2Cl2 (5 mL) and sat. aq. NaHCO3 (5 mL) were added and phases were separated. The aqueous phase was extracted with CH2Cl2 (3 x 10 mL). The combined organic layers were dried over anhyd Na2SO4, filtered and concentrated under reduced pressure. The crude product was purified by flash chromatography (silica gel, 2:1 hexane/EtOAc) to provide indolizidinone 21 (mixture of two diastereomers in approximately 2.9:1 ratio as determined by 1H NMR, 42 mg, 56%) as a colorless oil; Rf (2:1 hexane/EtOAc) 0.28; δH (300 MHz, CDCl3) 7.70-7.20 (m, 10H), 5.60-5.90 (m, 2H), 4.37 (d, J = 20.6 Hz, 1H), 3.94 (d, J = 13.7 Hz, 2H), 3.80-3.45 (buried m, 3H), 3.70 (d, J = 13.7 Hz, 2H), 2.43-2.18 (m, 2H), 2.02-1.80 (m, 2H); δC (75MHz, CDCl3) 172.7, 139.6, 129.0, 128.3, 127.0, 124.3, 123.8, 59.8, 54.6, 50.7, 40.0, 33.0, 29.3; [α]D25 –1.90 (c 1.1, CHCl3); νmax (film) 3028, 2925, 2842, 1685, 1453, 746, 699 cm-1; ESI-HRMS calculated for C22H24N2O [M+H]+ 333.1967, found 333.1966.
N-Boc-dibenzylaminoindoloquinolizidinone 22. To a solution of tetracyclic indoloquinolizidinone 17β (45.0 mg, 0.103 mmol) in CH2Cl2 (3 mL) was added Boc2O (112 mg in 1 mL CH2Cl2, 0.515 mmol), Et3N (72.0 µL, 0.515 mmol) and DMAP (1.3 mg, 0.0103 mmol). The mixture was stirred at room temperature for 6 h. Water (3 mL) and CH2Cl2 (3 mL) were added and phases were separated. The aqueous phase was extracted with CH2Cl2 (2 x 10 mL) and the combined organic layers were dried over anhyd Na2SO4, filtered and concentrated under reduced pressure. The crude product was purified by flash chromatography (silica gel, 10:1 hexane/EtOAc) to provide N-Boc-indoloquinolizidinone 22 (55.0 mg, quantitative) as a colorless oil; Rf (4:1 hexane/EtOAc) 0.56; δH (300 MHz, CDCl3) 8.03 (d, J = 8.0 Hz, 1H), 7.50-7.10 (m, 13H), 5.20 (d, J = 11.7 Hz, 1H), 5.08 (d, J = 10.5 Hz, 1H), 4.10 (d, J = 13.9 Hz, 2H), 3.83 (d, J = 13.8 Hz, 2H), 3.39 (dd, J = 11.3, 7.6 Hz, 1H), 2.90-2.69 (m, 3H), 2.59 (d, J = 13.2 Hz, 1H), 2.15-1.90 (m, 3H), 1.65 (s, 9H); δC (75 MHz, CDCl3) 170.9, 150.2, 140.8, 136.8, 135.2, 128.7, 128.5, 128.2, 127.2, 126.8, 124.6, 123.0, 118.3, 115.5, 84.3, 58.7, 56.2, 55.4, 38.7, 29.7, 28.2, 26.4, 21.6; [α]D25 +71.8 (c 1.6, CHCl3); νmax (film) 3417, 2924, 2853, 1730, 1644, 1455, 1368, 1141, 737, 699 cm-1; ESI-HRMS calculated for C34H38N3O3 [M+H]+ 536.2913, found 536.2903.
(S)-tert-Butyl 1,6,7,12b-tetrahydro-4-oxoindolo[2,3-a]quinolizine-12(4H)-carboxylate (23). To a solution of N-Boc-dibenzylamino-indoloquinolizidinone 22 (48.0 mg, 0.0901 mmol) in CHCl3 (5 mL) was added m-CPBA (33.3 mg, 70%, 0.135 mmol) at 0 °C. The mixture was stirred for 30 min CH2Cl2 (5 mL) and sat. aq. NaHCO3 (5 mL) were added and phases were separated. The aqueous phase was extracted with CH2Cl2 (2 x 10 mL) and the combined organic layers were dried over anhyd Na2SO4, filtered and concentrated under reduced pressure. The crude product was purified by flash chromatography (silica gel, 4:1 hexane/EtOAc) to provide tetracyclic enamide 20 (18.0 mg, 59%) as a colorless oil; Rf (2:1 hexane/EtOAc) 0.45; δH (300 MHz, CDCl3) 8.10 (d, J = 7.5 Hz, 1H), 7.50 (dd, J = 7.5, 1.6 Hz, 1H) 7.46-7.23 (m, 2H), 6.72 (ddd, J = 9.1, 6.7, 2.0 Hz, 1H), 6.13 (dd, J = 9.7, 2.9 Hz, 1H), 5.28 (d, J = 11.7 Hz, 1H), 5.05 (dd, J = 12.7, 3.4 Hz, 1H), 3.06 (ddd, J = 17.1, 6.5, 3.5 Hz, 1H), 2.96-2.71 (m, 2H), 2.28-2.12 (m, 1H), 1.70 (s, 9H), 1.90-1.40 (buried m, 1H); δC (75 MHz, CDCl3) 164.9, 150.1, 139.1, 136.6, 134.1, 128.6, 125.5, 124.7, 123.1, 118.4, 118.0, 115.8, 84.5, 53.3, 37.6, 31.7, 28.2, 21.6; [α]D25 +110.1 (c 0.9, CHCl3); νmax (film) 3422, 2924, 2853, 1727, 1660, 1613 1456, 1422, 1369, 1145cm-1; ESI-HRMS calculated for C20H22N2O3Na [M+Na]+ 361.1528, found 361.1532.
(S)-6,7-Dihydro-9,10-dimethoxy-1H-pyrido[2,1-a]isoquinolin-4(11bH)-one (24). To a solution of dibenzylaminobenzoquinolizidinone 18 (76.0 mg, 0.167 mmol) in CHCl3 (4 mL) was added m-CPBA (61.6 mg, 0.250 mmol) at 0 °C. The mixture was stirred for 30 min CH2Cl2 (5 mL) and sat. aq. NaHCO3 (5 mL) were added and phases were separated. The aqueous phase was extracted with CH2Cl2 (2 x 10 mL) and the combined organic layers were dried over anhyd Na2SO4, filtered and concentrated under reduced pressure. The crude product was purified by flash chromatography (silica gel, 4:1 hexane/ EtOAc) to provide tricyclic enamide 24 (32.0 mg, 74%) as a colorless oil; Rf (2:1 hexane/EtOAc) 0.44; δH (300 MHz, CDCl3) 6.70-6.60 (m, 1H), 6.67 (s, 1H), 6.62 (s, 1H), 6.10 (dd, J = 9.8, 2.9 Hz, 1H), 4.91-4.58 (m, 2H), 3.91 (s, 3H), 3.87 (s, 3H), 3.08-2.55 (m, 4H), 2.31 (dddd, J = 16.7, 14.0, 2.3, 2.3 Hz, 1H); δC (75 MHz, CDCl3) 164.8, 147.9, 147.9, 138.9, 127.6, 127.1, 125.6, 111.5, 108.6, 56.1, 55.9, 54.4, 38.0, 33.7, 29.0; [α]D25 –206.8 (c 0.8, CHCl3); νmax (film) 3448, 2923, 2851, 1659, 1610, 1515, 1431, 1257, 1228, 1124, 816, 786 cm-1. ESI-HRMS calculated for C15H17NO3Na [M+Na]+ 282.1106, found 282.1115.
ACKNOWLEDGEMENTS
The financial supports for this research were provided by the Thailand Research Fund (MRG5280114) and Faculty of Science, Silpakorn University. Scholarships for graduate study were supported by the Department of Chemistry, Faculty of Science, Silpakorn University for NP, PB, SA, CH, PS, and AB.
References
1. J. P. Michael, Nat. Prod. Rep., 2008, 25, 139. CrossRef
2. D. Gome-Pardo, D. Desmalële, and J. d’Angelo, Tetrahedron Lett. 1992, 33, 6633; CrossRef L. F. Tietze and Y. Zhou, Angew. Chem. Int. Ed., 1999, 38, 2045. CrossRef
3. K. Takada, T. Uehara, Y. Nakao, S. Matsunaga, R. W. M. Van Soest, and N. Fusetani, J. Am. Chem. Soc., 2004, 126, 187. CrossRef
4. M. J. Rishel, K. K. D. Amarasinghe, S. R. Dinn, and B. J. Johnson, J. Org. Chem., 2009, 74, 4001. CrossRef
5. R. W. Fitch, G. D. Sturgeon, S. R. Patel, T. F. Spande, H. M. Garraffo, J. W. Daly, and R. H. Blauuw, J. Nat. Prod., 2009, 72, 243. CrossRef
6. V. U. Ahmad, A. Rahman, T. Rasheed, and H. Rehman, Heterocycles, 1987, 26, 1251; CrossRef Q. Zhang, G. Tu, Y. Zhao, and T. Cheng, Tetrahedron, 2002, 58, 6795. CrossRef
7. I. Pastuszak, R. J. Molyneux, L. F. James, and A. D. Elbein, Biochemistry, 1990, 29, 1886. CrossRef
8. J. Stöckigt, A. P. Antonchick, F. Wu, and H. Waldman, Angew. Chem. Int. Ed., 2011, 50, 8538. CrossRef
9. V. Farina, J. T. Reeves, C. H. Senanayake, and J. J. Song, Chem. Rev., 2006, 106, 2734. CrossRef
10. D. Soerens, J. Sandrin, F. Ungemach, P. Morky, G. S. Wu, E. Yamanaka, L. Hutchins, M. Dipierro, and J. M. Cook, J. Org. Chem., 1979, 44, 535. CrossRef
11. M. E. Muratore, C. A. Holloway, A. W. Pilling, R. I. Storer, G. Trevitt, and D. J. Dixon, J. Am. Chem. Soc., 2009, 131, 10796. CrossRef
12. B. B. Maryanoff, H.-C. Zhang, J. H. Cohen, I. J. Turchi, and C. A. Maryanoff, Chem. Rev., 2004, 104, 1431. CrossRef
13. The configuration of the newly generated stereocenter was established using NOESY experiments. The major products have the configuration with α-H at the stereocenter in accord with our previously reported results of N-acyliminium ion cyclization leading to the tricyclic core of schulzeines (see ref. 15).
14. An example of π-π interaction directing the facial preference in N-acyliminium ion cyclizations; A. G. Schultz and L. Pettus, J. Org. Chem., 1997, 62, 6855. CrossRef
15. P. Kuntiyong, S. Akkarasamiyo, and G. Eksinitkun, Chem. Lett., 2006, 35, 1008; CrossRef P. Kuntiyong, S. Akkarasamiyo, N. Piboonsrinakara, C. Hemmara, and P. Songthammawat, Tetrahedron, 2011, 67, 8034. CrossRef