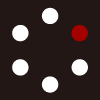
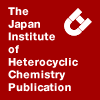
HETEROCYCLES
An International Journal for Reviews and Communications in Heterocyclic ChemistryWeb Edition ISSN: 1881-0942
Published online by The Japan Institute of Heterocyclic Chemistry
e-Journal
Full Text HTML
Received, 18th December, 2013, Accepted, 16th January, 2014, Published online, 27th January, 2014.
DOI: 10.3987/COM-13-12918
■ Bisindole Alkaloids Condensed with a Cyclopropane Ring, Part 1. 14,15-Cyclopropanovinblastine and -vincristine
Péter Keglevich, László Hazai, Zsófia Dubrovay, Miklós Dékány, Csaba Szántay, Jr., György Kalaus, and Csaba Szántay*
Department of Organic Chemistry and Technology, Budapest University of Technology and Economics, H-1111 Budapest, Szt. Gellért tér 4, Hungary
Abstract
A new type of vinblastine and vincristine derivatives was synthesized. The carbon carbon double bond in position 14 and 15 of the vindoline ring was cyclopropanated. In the course of the synthetic work 14,15-cyclopropanovindoline was coupled with catharanthine, resulting in the cyclopropanated anhydrovinblastine as a key intermediate to 14,15-cyclopropano-vinblastine and -vincristine.INTRODUCTION
The dimeric alkaloids vinblastine (1) and vincristine (2) (Scheme 1) were isolated from the Madagascar periwinkle Catharanthus roseus. Both materials have been used in anticancer chemotherapy for more than forty years.1,2
Vinblastine (1) is an antimicrotubule drug used to treat certain kinds of cancer, including Hodgkin's lymphoma, non-small cell lung cancer, breast cancer, head and neck cancer, and testicular cancer. Vincristine (2), the formyl derivative of vinblastine, can be used in various types of chemotherapy regimens. Its main uses are in non-Hodgkin's and Hodgkin's lymphoma, acute lymphoblastic leukemia, and in the treatment of nephroblastoma (Wilms tumor, a kidney tumor most common in young children).
In the course of the synthetic investigations of dimeric Vinca alkaloids various methods have been elaborated to synthesize new derivatives of vinblastine (1) and vincristine (2) with improved therapeutic effect exhibiting higher selectivity and lower toxicity.3,4 One of the structural modifications was very simple and only a little part of the large molecule was changed; 14,15-dihydrovinblastine (3) having a saturated vindoline ring was simply prepared by catalytic hydrogenation of vinblastine (1), and the antitumor activity almost ceased.5
The introduction of a cyclopropane ring in place of the C(14)=C(15) carbon carbon double bond of the vindoline ring (4) can be regarded as a minor change within the overall molecular framework of vinblastine; however, the electronic nature of this small ring has entirely special properties. According to NMR and X-ray crystallographic studies, the electronic structure of the cyclopropane ring cannot be described in terms of classical methods.6,7
Our enquiry was aimed at finding out how the introduction of this unique structural system into vinblastine and vincristine will affect their biological effects. To that end we set out to synthesize 14,15-cyclopropanovinblastine (4) and some derivatives thoseof.
RESULTS AND DISCUSSION
Synthesis
A number of cyclopropanation reactions are known in the literature8 such as the Simmons-Smith reaction9,10 and its variants, e.g. that involve triisobutylaluminium11 or diazomethane in the presence of a catalyst.12
First we attempted the direct cyclopropanation of vinblastine (VBL) (1) and vincristine (VCR) (2). In the course of the reaction of vinblastine (1) using diiodomethane in the presence of diethylzinc in dichloromethane solution no cyclopropanation occurred; a quaternary salt (5) was isolated in 18% yield and two rearranged products were found in a 2:1 mixture by NMR spectroscopy, vinamidine13-16 (7) and cyclovinblastine17 (9) (Scheme 2). The latter are known in the literature and were identified by NMR.
Similarly, when treating vincristine (2) under such reaction conditions the corresponding 6 quaternary salt was isolated in 9% yield, and N-formylcatharinine18 (8) and cyclovincristine17 (10) were identified by NMR spectroscopy in a 1:1 mixture. Using the triisobutylaluminium method,11 only decomposed products were obtained from the reaction mixtures.
Therefore the coupling reaction traditionally used to obtain dimeric alkaloids was selected to synthesize the expected cyclopropanated derivatives.
In the reaction of vindoline (11) with diiodomethane in the presence of diethylzinc in dichloromethane solution, only the cyclopropanated dimer (12) could be isolated (Scheme 3) with 6% yield.
The 14,15-cyclopropano derivative of 10-bromovindoline (14) was previously prepared by us19 from 10-bromovindoline20 (13) in the reaction with diiodomethane and with diethylzinc in dichloromethane (Scheme 3). 10-Bromo-14,15-cyclopropanovindoline (14) was treated in methanol with sodium borohydride in the presence of palladium on charcoal, which resulted in the expected 14,15-cyclopropanovindoline (15) unsubstituted in position 10.
Compound 15 proved to be suitable for the coupling reaction with catharanthine to give cyclopropanovinblastine and cyclopropano-vincristine.
The next step in our synthetic work was to prepare the cyclopropanated anhydrovinblastine (17), which can be an important intermediate in the synthesis of further derivatives (Scheme 4). 14,15-Cyclopropanovindoline (15) was coupled with catharanthine (16) by the method of biomimetic iron(III)-promoted coupling reaction.21,22 The procedure used by us is the traditional coupling reaction for the preparation of dimeric alkaloids.
Catharanthine (16) and 14,15-cyclopropanovindoline (15) were dissolved in diluted aqueous hydrochloric acid solution together with the non-nuclepophilic cosolvent 2,2,2-trifluoroethanol (Scheme 4). The reaction was carried out at room temperature. Sodium borohydride was used as the reducing agent in water at 0 oC. 14,15-Cyclopropanoanhydrovinblastine (17) was obtained in 51% yield and was isolated by preparative thin-layer chromatography. From 17 base the sulfate salt was prepared immediately.
Oxidation of the obtained anhydrovinblastine derivative (17) to 14,15-propanovinblastine (18) was carried out by the known oxidation protocol21,22 utilizing the oxalate salt Fe2(ox)3 and air at 0 oC in water, diluted aqueous hydrochloric acid and 2,2,2-trifluoroethanol mixture. After purification the crude product with preparative thin-layer chromatography was transformed to the sulfate salt. 14,15-Cyclopropanovinblastine (18) was obtained in 13% yield.
The new cyclopropano derivative of vinblastine (18) was oxidized23 to 14,15-cyclopropanovincristine (19) by chromium(VI) oxide in acetone-acetic acid solution in the presence of acetic anhydride at -60 oC. The crude product which contained some N-demethylvincristine was reformylated with formic acid/acetic anhydride. After isolation the product by preparative thin-layer chromatography, sulfate salt was prepared and the expected product (19) was obtained in 52% yield.
Biology
The new compounds synthesized by us were sent to the US National Institute of Health (NIH) where they were subjected to pharmacological investigations. These experiments on 56 different tumor cell lines embracing 9 frequently occurring tumor types demonstrated the therapeutic activity of these substances in comparison with other known effective catharanthus alkaloids.
Tumor types and cell lines were the following: leukemia (CCRF-CEM, HL(60)-TB, K-562, MOLT-4, RPMI-8226, SR), non-small cell lung cancer (A549/ATCC, EKVX, HOP-62, HOP-92, NCI-H226, NCI-H23, NCI-H322M, NCI-H460, NCI-H522), colon cancer (COLO 205, HCC-2998, HCT-116, HCT-15, HT-29, KM12, SW-620), CNS cancer (SF-268, SF-295, SF-539, SNB-19, SNB-75, U251), melanoma (LOX IMVI, MALME-3M, M14, MDA-MB-435, SK-MEL-2, SK-MEL-28, SK-MEL-5, UACC-257, UACC-62), ovarian cancer (IGROV1, OVCAR-3, OVCAR-4, OVCAR-8, NCI/ADR-RES, SK-OV-3), renal cancer (786-0, A498, ACHN, CAKI-1, RXF 393, SN12C, TK-10, UO-31), prostate cancer (PC-3, DU-145), breast cancer (MCF7, MDA-MB-231/ATCC, HS 578T, BT-498, T-47D, MDA-MB-468).
The NCI screening procedures were described24 as were the origins and processing of the cell lines.24-26 In Table 1 percentages of growths are listed for the reference alkaloids and the appropriate cyclopropano derivatives at the concentration of 10-5M. The bigger negative numbers show more significant decrease of cell number or stronger inhibition effects.
In the case of leukemia cancer cell line HL-60(TB) treated with 14,15-cyclopropano-vinblastine (18) a significant cell number decrease was observed. On SR cell line cyclopropanation also enhanced the inhibiting effect. Cyclopropano-vincristine (19) was, however, ineffective on all of leukemia cell lines investigated.
From the non-small cell lung cancer lines cells of NCI-H226 treated with cyclopropanated vinblastine (18) and in the case of HOP-92 treated with cyclopropano-vincristine (19) a significant cell number decrease was observed, and in the cell line of NCI-H23 treated with compound 19 the intensive cell number increase was practically stopped. On lines HOP-92 and NCI-H23 cyclopropanated derivative 18 proved to be also ineffective.
In the case of colon cancer cell line COLO-205 all the two molecule types gave great cell number decrease and on both molecule types (18, 19) cyclopropanation resulted in significant extra decreasing effect against the non-cyclopropanated forms. In the case of HCT-116 line treated with cVBL (18) a significant cell number decrease was observed as a result of cyclopropanation. In the case of the HT29 line treated with cVCR (19), also a significant cell number decrease was observed. The KM12 cell line treated with cVCR (19) cyclopropanation also resulted in a significant decrease of cell numbers.
On CNS tumor cell lines cyclopropanated vinblastine (18) showed moderate inhibiting effect, in the case of cyclopropano-vincristine (19) anticancer activity was not observed.
In two melanoma lines (M14 and SK-MEL-5) significant inhibiting effects were detected with cVBL (18) with more decided inhibition than with VBL. In the cases of MDA-MB-435 and SK-MEL-2 cell lines treated with cVCR (19) significant inhibiting effects were obtained. In the case of the SK-MEL-2 line the inhibiting effect was significantly stronger with cVCR (19) than with VCR.
With ovarian cancer cell lines cVCR (19) increased the inhibiting effect of vincristine only on the OVCAR-3 cell line, and in the case of renal cancer cyclopropanated vinblastine (18) caused moderate inhibition in the proliferation of cancer cells.
In the case of the prostate cancer, on cell line DU-145 cVCR (19) caused significant cell number decrease, but extra inhibiting effects were not detected. In the case of the breast cancer lines on BT-549 a significant decrease of cell numbers was measured.
According to Table 1 cyclopropano-vinblastine (18) has significant tumor cell inhibiting effect in leukemia, non-small-cell lung cancer, colon cancer, melanoma and breast cancer, cyclopropano-vincristine (19) has significant tumor cell inhibiting effect in colon cancer, melanoma, ovarian cancer and prostate cancer.
EXPERIMENTAL
General
Melting points were measured on VEB Analytik Dresden PHMK-77/1328 apparatus and are uncorrected. IR spectra were recorded on Zeiss IR 75 and 80 instruments. NMR measurements were performed on a Varian 800 MHz NMR spectrometer equipped with a 1H{13C/15N} Triple Resonance 13C Enhanced Salt Tolerant Cold Probe operating at 800 MHz for 1H and 201 MHz for 13C, and a Varian 500 MHz NMR spectrometer equipped with a 1H {13C/15N} 5 mm PFG Triple Resonance 13C Enhanced Cold Probe operating at 500 MHz for 1H and 125 MHz for 13C. Chemical shifts are given on the delta scale as parts per million (ppm) with tetramethylsilane (TMS) (1H) or dimethyl sulfoxide-d6 (13C) as the internal standard (0.00 ppm and 39.5 ppm, respectively). 1H-1H, direct 1H-13C, and long-range 1H-13C scalar spin-spin connectivities were established from 2D gDQFCOSY, zTOCSY, gHSQCAD, and gHMBCAD experiments, respectively. All pulse sequences were applied by using the standard spectrometer software package. All experiments were performed at 298 K. HRMS analyses were performed on an LTQ FT Ultra (Thermo Fisher Scientific, Bremen, Germany) system. The ionization method was ESI operated in positive ion mode. For the CID experiment helium was used as the collision gas, and normalized collision energy (expressed in percentage), which is a measure of the amplitude of the resonance excitation RF voltage applied to the endcaps of the linear ion trap, was used to induce fragmentation. The protonated molecular ion peaks were fragmented by CID at a normalized collision energy of 35–55%. The samples were dissolved in methanol. Data acquisition and analysis were accomplished with Xcalibur software version 2.0 (Thermo Fisher Scientific). TLC was carried out using Kieselgel 60F254 (Merck) glass plates.
Cyclopropanation reaction of vinblastine. Vinblastine (1) (406 mg, 0.5 mmol) was dissolved in CH2Cl2 (30 mL) and under Ar at 0 oC 1.4 mL (1.4 mmol in 1M hexane solution) of diethylzinc and then 0.09 mL (1.1 mmol) diiodomethane was injected to the solution. The reaction mixture was stirred for 30 min at 0 oC and then for 5 h at room temperature. After allowing the solution to stand overnight, the addition of diethylzinc and diiodomethane was repeated at room temperature and after stirring for 7 h the reaction mixture was filtered and the filtrate was evaporated to dryness. The crude product was separated by preparative thin-layer chromatography (CH2Cl2-MeOH 10:1) and 84 mg (18%) of quaternary salt (5) was isolated. One of the other fractions (27 mg) was the 2:1 mixture of vinamidine (7) and cyclovinblastine (9). Compounds 7 and 9 proved to be equivalent with the compound known in the literature by their NMR spectra. Quaternary salt, 5: mp 277–279 °C. IR (KBr) 3445, 2949, 1728, 1615, 1232, 1038, 742 cm-1. 1H-NMR (800 MHz, D2O+CD3CN 1:1) δ (ppm) 1.00 (t br, J = 7.6 Hz, 3H, H3-18’); 1.08 (t br, J = 7.2 Hz, 3H, H3-18); 1.77 (m br, 1H, Hx-19); 1.88 (br, 1H, H2-19’); 1.89 (m, 1H, H-14’); 2.02 (m, 1H, Hy-19); 2.06 (m br, 2H, H2-15’); 2.31 (br, 1H, Hα-6); 2.44 (s, 3H, C(17)OCOCH3); 2.66 (br, 1H, Hβ-6); 2.79 (dd, J = 16.0 Hz, J = 4.3 Hz, 1H, Hα-17’); 3.10 (s, 3H, N(1)CH3); 3.25 (br, 1H, Hα-3’); 3.37 (br, 1H, Hα-5); 3.45 (s, 3H, N(4’)CH3); 3.55 (br, 1H, Hα-3); 3.56 (br, 1H, Hx-21’); 3.89 (br, 1H, Hα-6’); 3.80 (br, 1H, H-21); 3.91 (br, 1H, Hy-21’); 3.92 (br, 1H, Hx-5’); 3.98 (br, 1H, Hβ-5); 4.02 (br, 1H, Hβ-17’); 4.03 (s, 3H, C(16’)COOCH3); 4.07 (s, 1H, H-2); 4.10 (d, J = 12.7 Hz, 1H, Hβ-3’); 4.10 (s, 3H, C(11)OCH3); 4.15 (br, 1H, Hβ-3); 4.16 (s, 3H, C(16)COOCH3); 4.64 (br, 1H, Hβ-6’); 4.98 (dd, J = 16.4 Hz, J = 9.3 Hz, 1H, Hy-5’); 5.58 (s, 1H, H-17); 5.90 (d, J = 10.6 Hz, 1H, H-15); 6.29 (dd, J = 10.6 Hz, J = 5.1 Hz, 1H, H-14); 6.75 (s br, 1H, H-12); 7.13 (s, 1H, H-9); 7.50 (m, 1H, H-10’); 7.56 (m, 1H, H-11’); 7.70 (d, J = 8.1 Hz, 1H, H-12’); 7.98 (d, J = 8.1 Hz, 1H, H-9’). 13C-NMR (200 MHz, D2O+CD3CN 1:1) δ (ppm) 6.6 (C-18’); 7.7 (C-18); 19.0 (C-6’); 20.7 (C(17)OCOCH3); 28.9 (C-14’); 31.4 (C-19); 35.0 (C-19’); 36.4 (C-17’); 36.5 (C-15’); 38.1 (C-1); 43.0 (C-20); 44.1 br (C-6); 49.7 (C-5); 50.4 (C-3); 53.0 (C-7); 53.2 (C(16’)COOCH3); 53.6 (C(16)COOCH3); 55.6 (C-16’); 56.4 (C(11)OCH3); 57.6 (C-3’); 60.1 (N(4’)CH3); 65.9 (C-21); 66.3 (C-5’); 70.3 (C-20’); 72.1 (C-21’); 75.5 (C-17); 80.3 (C-16); 80.9 (C-2); 94.6 (C-12); 111.9 (C-12’); 112.5 (C-7’); 118.6 (C-9’); 120.0 (C-10’); 120.8 (C-10); 121.0 (C-8); 123.2 (C-14); 123.3 (C-11’); 124.2 (C-9); 129.6 (C-8’); 130.9 (C-15); 131.6 (C-2’); 136.0 (C-13’); 153.4 (C-13); 158.9 (C-11); 172.0 (C(17)OCOCH3); 172.1 (C(16)COOCH3); 175.7 (C(16’)COOCH3). HRMS: 825.44322 (C47H61O9N4; calc. 825.44331). ESI-MS-MS (rel. int. %): 807(1); 793(5); 765(7); 556(4); 541(17); 483(7); 481(10); 469(100).
Cyclopropanation reaction of vincristine. Vincristine (2) (176 mg, 0.213 mmol) was dissolved in CH2Cl2 (12 mL) and under Ar at 0 oC 0.56 mL (0.56 mmol in 1M hexane solution) of diethylzinc and then 0.04 mL (0.43 mmol) diiodomethane were injected to the solution. The reaction mixture was stirred for 30 min at 0 oC and then for 5 h at room temperature. After allowing the solution to stand overnight the addition of diethylzinc and diiodomethane was repeated at room temperature and after stirring for 8 h the reaction mixture was filtered and the filtrate was evaporated to dryness. The crude product was separated by preparative thin-layer chromatography (CH2Cl2-MeOH 9:1) and 15 mg (9%) of quaternary salt (6) was isolated. One of the other fractions (11 mg) was the 1:1 mixture of N-formylcatharinine (8) and cyclovincristine (10). Compounds 8 and 10 proved to be equivalent with the compound known in the literature on the basis of their NMR spectra. Quaternary salt, 6: mp >280 °C. IR (KBr) 3423, 2923, 1731, 1658, 1619, 1460, 1370, 1235, 1030, 744 cm-1. 1H-NMR (800 MHz, D2O+CD3CN 1:1) δ (ppm) 0.90 (t, J = 7.2 Hz, 3H, H3-18); 1.12 (t, J = 7.6 Hz, 3H, H3-18’); 1.46 (m, 1H, Hx-19); 1.63 (m, 1H, H-14’); 1.68 (m, 1H, Hy-19); 1.71 (br, 1H, H2-19’); 1.82 (dd, J = 14.5 Hz, J = 7.0 Hz, 1H, Hα-15’); 1.92 (m, 1H, Hβ-15’); 1.97 (m, 1H, Hα-6); 2.24 (s, 3H, C(17)OCOCH3); 2.31 (br, 1H, Hβ-6); 2.71 (dd, J = 16.4 Hz, J = 4.8 Hz, 1H, Hα-17’); 2.80 (m, 1H, Hα-5); 2.96 (m, 1H, Hα-3); 2.99 (dd, J = 14.1 Hz, J = 6.0 Hz, 1H, Hα-3’); 3.27 (s, 3H, N(4’)CH3); 3.30 (s, 1H, H-21); 3.53 (m, 1H, Hβ-5); 3.61 (dd, J = 16.3 Hz, J = 5.1 Hz, 1H, Hβ-3); 3.71 (m, 1H, Hα-6’); 3.74 (d, J = 13.5 Hz, 1H, Hx-21’); 3.79 (m, 1H, Hx-5’); 3.81 (m, 1H, Hy-21’); 3.83 (s, 3H, C(16)COOCH3); 3.85 (m, 1H, Hβ-17’); 3.92 (s, 3H, C(16’)COOCH3); 4.12 (s, 3H, C(11)OCH3); 4.27 (d, J = 14.1 Hz, 1H, Hβ-3’); 4.58 (m, 1H, Hβ-6’); 4.69 (m, 1H, Hy-5’); 4.75 (s, 1H, H-2); 5.20 (s, 1H, H-17); 5.59 (d, J = 10.2 Hz, 1H, H-15); 6.13 (dd, J = 10.2 Hz, J = 5.1 Hz, 1H, H-14); 7.15 (s, 1H, H-9); 7.36 (m, 1H, H-10’); 7.39 (s, 1H, H-12); 7.42 (m, 1H, H-11’); 7.58 (d, J = 8.1 Hz, 1H, H-12’); 7.83 (d, J = 8.4 Hz, 1H, H-9’); 9.11 (s, 1H, N(1)CHO). 13C-NMR (200 MHz, D2O+CD3CN 1:1) δ (ppm) 6.2 (C-18’); 7.5 (C-18); 20.3 (C(17)OCOCH3); 21.3 (C-6’); 28.4 (C-14’); 30.6 (C-19); 34.5 (C-19’); 35.6 br (C-17’); 35.9 (C-15’); 40.9 (C-6); 42.4 (C-20); 48.4 (C-5); 49.8 (C-3); 52.9 (C-7); 53.1 (C(16’)COOCH3); 53.1 (C(16)COOCH3); 55.6 br (C-16’); 56.5 (C(11)OCH3); 56.8 (C-3’); 60.2 (N(4’)CH3); 63.3 (C-21); 66.6 (C-5’); 69.8 (C-20’); 71.4 (C-2); 71.8 (C-21’); 77.1 (C-17); 79.6 (C-16); 96.1 (C-12); 111.8 (C-12’); 113.0 (C-7’); 118.4 (C-9’); 119.8 (C-10’); 127.0 (C-10); 123.2 (C-11’); 124.7 (C-8); 125.2 (C-9); 125.6 (C-14); 127.0 (C-10); 129.2 (C-8’); 129.4 (C-15); 130.3 (C-2’); 135.8 (C-13’); 141.5 (C-13); 157.9 (C-11); 162.8 (C-1); 172.1 (C(17)OCOCH3); 172.4 (C(16)COOCH3); 175.3 (C(16’)COOCH3). 15N-NMR (81 MHz, D2O+CD3CN 1:1) δ -230 (N-1); -322 (N-4’). HRMS: 839.42275 (C47H59O10N4; calc. 839.42257). ESI-MS-MS (rel. int. %): 821(15); 807(30); 779(18); 761(13); 747(7); 729(6); 719(15); 704(6); 696(11); 678(25); 618(6); 555(100); 537(9); 513(9); 495(51); 455(7); 453(12); 435(19); 409(6); 395(4).
Reaction of vindoline (11) with diiodomethane. Vindoline (11) (228 mg, 0.5 mmol) was dissolved in CH2Cl2 (20 mL) and under Ar at 0 °C 1.28 mL (1.28 mmol in 1M hexane solution) of diethylzinc and then 0.08 mL (1.0 mmol) diiodomethane was injected to the solution. The reaction mixture was stirred for 30 min at 0 °C and then for 6 h at room temperature. After allowing the solution to stand overnight, the addition of diethylzinc and diiodomethane was repeated at room temperature and after stirring for 8 h the reaction mixture was filtered and the filtrate was evaporated to dryness. The crude product was separated by preparative thin-layer chromatography (CH2Cl2-MeOH 20:1) and 26 mg of the dimer (12) was isolated. Mp 119-121 °C. IR (KBr) 3445, 2996, 1743, 1615, 1372, 1249, 1011 cm-1. 1H-NMR (800 MHz, DMSO-d6) δ (ppm) 0.27 (m, 1H, H-15); 0.43 (t br, J = 7.3 Hz, 3H, H3-18); 0.60 (m, 1H, Hx-22); 0.73 (m, 1H, Hx-19); 0.77 (m, 1H, Hy-22); 1.12 (m, 1H, H-14); 1.61 (m, 1H, Hy-19); 1.96 (s, 3H, C(17)OCOCH3); 2.11 (m, 2H, H2-6); 2.12 (s, 1H, H-21); 2.23 (m, 1H, Hα-3); 2.29 (m, 1H, Hα-5); 2.52 (s, 3H, N(1)CH3); 3.17 (m, 1H, Hβ-5); 3.24 (m, 1H, Hβ-3); 3.32 (s, 1H, H-2); 3.60 (s, 2H, C(10)CH2); 3.66 (s, 3H, C(16)COOCH3); 3.76 (s, 3H, C(11)OCH3); 5.24 (s, 1H, H-17); 6.26 (s, 1H, H-12); 6.86 (s, 1H, H-9). 13C-NMR (200 MHz, DMSO-d6) δ (ppm) 7.9 (C-18); 8.6 (C-22); 15.0 (C-14); 15.8 (C-15); 21.0 (C(17)OCOCH3); 29.1 (C(10)CH2); 34.0 (C-19); 39.4 (C-1); 40.3 (C-20); 44.3 (C-6); 53.1 (C-5); 53.3 (C-3); 51.8 (C-7); 51.8 (C(16)COOCH3); 55.2 (C(11)OCH3); 70.0 (C-21); 76.5 (C-17); 78.1 (C-16); 83.3 (C-2); 93.7 (C-12); 120.7 (C-10); 124.9 (C-8); 123.6 (C-9); 151.4 (C-13); 157.7 (C-11); 169.9 (C(17)OCOCH3); 171.7 (C(16)COOCH3). HRMS: 953.48953 (C53H69O12N4; calc. 953.49065). ESI-MS-MS (rel. int. %): 893(100); 833(23); 670(46); 610(26); 483(7); 447(14); 423(16); 389(5).
14,15-Cyclopropanovindoline, 15. 10-Bromo-14,15-cyclopropanovindoline (14) (1.341 g; 2.441 mmol) was dissolved in MeOH (67 mL), the mixture was cooled to 10 °C, then under Ar palladium on charcoal (2.012 g) and sodium borohydride (1.385 g; 36.615 mmol) was added in portions. After 30 min another 1.385 g (36.615 mmol) of sodium borohydride was added in portions and after 30 min some drops of acetic acid were added to the reaction mixture. After filtration of the catalyst the filtrate was evaporated, dissolved in CH2Cl2 (150 mL) and was washed with 10% of aqueous sodium carbonate (100 mL). The water phase was washed with CH2Cl2 (50 mL) and the combined organic phase was extracted with water (100 mL), dried with magnesium sulfate and the solvent was evaporated. The crude product was purified by preparative TLC (CH2Cl2-MeOH 20:1) and 707 mg (62%) white crystals of 15 were obtained. Mp 89-91 °C. TLC (CH2Cl2-MeOH 20:1), Rf = 0.52. [α]29D -34.4 (c 1, CH2Cl2). IR (KBr) 3440, 2962, 1741, 1613, 1598, 1371, 1250, 1039 cm-1. 1H-NMR (800 MHz, DMSO-d6) δ (ppm) 0.29 (m, 1H, Hα-15); 0.60 (m, 1H, Hx-22); 0.65 (t, J = 7.3 Hz, 3H, H3-18); 0.81 (ABq, J = 14.0 Hz, J = 7.3 Hz, 1H, Hx-19); 0.82 (m, 1H, Hy-22); 1.13 (m, 1H, Hα-14); 1.69 (ABq, J = 14.0 Hz, J = 7.3 Hz, 1H, Hy-19); 1.97 (s, 3H, C(17)OCOCH3); 2.10 (dt, J = 13.5 Hz, J = 9.2 Hz, 1H, Hß-6); 2.17 (ddd, J = 13.5 Hz, J = 10.0 Hz, J = 3.4 Hz, 1H, Hα-6); 2.31 (s, 1H, H-21); 2.34 (dd, J = 10.9 Hz, J = 3.7 Hz, 1H, Hα-3); 2.39 (dt, J = 10.0 Hz, J = 9.2 Hz, 1H, Hα-5); 2.54 (s, 3H, N(1)CH3); 3.14 (td, J = 9.2 Hz, J = 3.4 Hz, 1H, Hß-5); 3.19 (d br, J = 10.9 Hz, 1H, Hß-3); 3.41 (s, 1H, H-2); 3.67 (s, 3H, C(16)COOCH3); 3.71 (s, 3H, C(11)OCH3); 5.23 (s, 1H, H-17); 6.21 (d, J = 2.3 Hz, 1H, H-12); 6.29 (dd, J = 8.3 Hz, J = 2.3 Hz, 1H, H-10); 7.36 (d, J = 8.3 Hz, 1H, H-9); 7.74 (s, 1H, OH). 13C-NMR (200 MHz, DMSO-d6) δ (ppm) 8.0 (C-18); 8.5 (C-22); 11.3 (C-14); 15.8 (C-15); 20.8 (C(17)OCOCH3); 34.1 (C-19); 38.5 (C-1); 40.3 (C-20); 44.5 (C-6); 51.6 (C-7); 51.8 (C(16)COOCH3); 52.69 (C-5); 52.74 (C-3); 55.1 (C(11)OCH3); 69.4 (C-21); 76.5 (C-17); 78.1 (C-16); 83.2 (C-2); 95.6 (C-12); 104.9 (C-10); 123.0 (C-9); 126.3 (C-8); 153.4 (C-13); 160.4 (C-11); 169.9 (C(17)OCOCH3); 171.7 (C(16)COOCH3). MS: 471 (C26H35O6N2/M+H/). ESI-MS-MS (CID = 35 %) (rel. int. %): 411(100); 188(28). HRMS: 471.24864 (C26H35O6N2; calc. 471.24896). ESI-MS-MS (rel. int. %): 411(100); 393(2); 351(3); 236(3); 188(14).
14,15-Cyclopropanoanhydrovinblastine, 17. Catharanthine sulfate (16.H2SO4) (238 mg) was added to a mixture of CH2Cl2 (30 mL) and distilled water (30 mL) and the mixture was basified to pH 8 with NH4OH. After extraction the water phase was washed with CH2Cl2 (20 mL) and the combined organic phase was dried with magnesium sulfate and the solvent was evaporated. 200 mg (0.594 mmol) of catharanthine (16) base was obtained and it was dissolved together with 14,15-cyclopropanovindoline (15) (280 mg; 0.594 mmol) in a mixture of 0.1 M HCl (11.2 mL), distilled water (11.2 mL) and 2,2,2-trifluoroethanol (2.2 mL), then under Ar FeCl3x6H2O (802 mg; 2.97 mmol) was added. The reaction mixture was stirred at room temperature for 2 h and at 0 °C a solution of sodium borohydride (24 mg; 0.594 mmol) in distilled water (1.9 mL) was added. After 30 min stirring at 0 °C 25% NH4OH (12 mL) was added to the reaction mixture and filtrated. The filtrate was extracted with CH2Cl2 (3 x 60 mL), then the combined organic phase was washed with distilled water (100 mL), dried with magnesium sulfate and the solvent was evaporated. The crude product was purified by preparative TLC (EtOAc-MeOH 9:1) and 244 mg (51%) pale yellow crystals (17) were obtained. From the base (17) the sulfate salt was prepared. 41 mg (0.050 mmol) of 14,15-cyclopropanoanhydrovinblastine (17) was dissolved in a mixture of CH2Cl2 (0.4 mL) and EtOH (0.25 mL), then 0.28 ml of sulfuric acid-EtOH (from a mixture of 1 ml of cc. sulfuric acid and 100 mL of EtOH) was added and the mixture was evaporated to dryness. The solid product was treated with Et2O and after filtration 34 mg of 14,15-cyclopropanoanhydrovinblastine sulfate (17.H2SO4) was obtained. Mp (sulfate) 242-244 °C. TLC (base) (CH2Cl2-MeOH 10:1), Rf = 0.45. TLC (sulfate) (CH2Cl2-MeOH 10:1), Rf = 0.45. IR (sulfate) (KBr) 3416, 3088, 2963, 1736, 1614, 1503, 1434, 1373 cm-1. 1H-NMR (800 MHz, DMSO-d6+TFA) δ (ppm) 0.56 (br, 1H, Hα-15); 0.73 (br, 1H, Hx-22); 0.86 (br, 1H, Hy-22); 0.97 (t br, 3H, H3-18); 1.00 (t br, 3H, H3-18’); 1.37 (br, 1H, Hx-19); 1.38 (m, 1H, Hα-14); 1.87 (br, 1H, Hy-19); 1.95 (br, 1H, Hα-6); 2.05 (s, 3H, C(17)OCOCH3); 2.05 (br, 1H, H2-19’); 2.10 (br, 1H, Hα-17’); 2.25 (br, 1H, Hß-6); 2.67 (s, 3H, N(1)CH3); 2.75 (br, 1H, Hα-3’); 2.99 (br, 1H, Hß-17’); 3.21 (br, 2H, Hα-3, Hα-5); 3.36 (br, 1H, Hα-6’); 3.45 (br, 1H, H-21); 3.46 (br, 1H, Hx-5’); 3.52 (br, 1H, Hy-5’); 3.53 (br, 1H, Hß-5); 3.59 (s, 3H, C(16’)COOCH3); 3.61 (s, 1H, H-2); 3.67 (br, 1H, Hß-3); 3.69 (br, 1H, Hß-6’); 3.70 (br, 1H, Hα-21’); 3.79 (s, 3H, C(16)COOCH3); 3.80 (s, 3H, C(11)OCH3); 4.00 (br, 1H, Hy-21’); 4.10 (d, J = 12.7 Hz, 1H, Hß-3’); 5.23 (s, 1H, H-17); 5.75 (br, 1H, H-15’); 6.50 (s br, 1H, H-12); 7.04 (s br, 1H, H-9); 7.03 (br, 1H, H-10’); 7.11 (br, 1H, H-11’); 7.32 (br, 1H, H-12’); 7.57 (br, 1H, H-9’); 8.23 (br, 1H, H-4); 11.22 (br, 1H, H-4’). 13C-NMR (200 MHz, D2O+CD3CN 1:1) δ (ppm) 6.9 (C-22); 7.8 (C-18); 10.2 (C-14); 11.5 (C-18’); 15.4 (C-15); 19.0 (C-6’); 20.7 (C(17)OCOCH3); 27.4 (C-19’); 29.7 (C-14’); 34.3 br (C-17’); 35.9 (C-19); 38.2 (C-1); 40.0 (C-20); 44.4 (C-6); 45.3 (C-3’); 47.9 (C-21’); 50.4 (C-3); 50.6 (C-5); 51.5 (C-7); 52.4 (C(16’)COOCH3); 52.8 (C(16)COOCH3); 55.0 br (C-16’); 56.3 (C(11)OCH3); 53.6 (C-5’); 66.5 (C-21); 74.7 (C-17); 78.1 (C-16); 80.4 (C-2); 94.7 (C-12); 111.9 (C-7’); 112.1 (C-12’); 117.9 (C-9’); 118.8 (C-10’); 120.8 br (C-10); 121.9 (C-11’); 122.2 (C-8); 124.5 (C-15’); 124.9 (C-9); 128.6 (C-8’); 131.9 (C-2’); 135.8 (C-20’); 135.9 (C-13’); 152.5 (C-13); 157.9 (C-11); 169.9 (C(17)OCOCH3); 171.6 (C(16)COOCH3); 173.5 (C(16’)COOCH3). HRMS: 807.43268 (C47H59O8N4/Mbase+H/; calc. 807.43274). ESI-MS-MS (CID = 55%) (rel. int. %): 747(100); 577(49); 559(16); 522(45); 490(19); 379(8); 352(29); 337(10); 238(9).
14,15-Cyclopropanovinblastine, 18. 3.0 g (6.21 mmol) of Fe2(oxalate)3x6H2O was dissolved in distillated water (725 mL), then the solution was cooled at 0 oC and air was bubbled through the mixture for 10 min. Then 167 mg (0.207 mmol) of 14,15-cyclopropanoanhydrovinblastine (17) was added at 0 °C in a mixture of 0.1 M HCl (5.0 mL), distillated water (5.0 mL) and 2,2,2-trifluoroethanol (1.0 mL), and a solution of sodium borohydride (157 mg; 4.14 mmol) in distillated water (10 mL) was added to the reaction mixture. After stirring for 30 min, 25% NH4OH (23 mL) was added, and was extracted with a mixture of CH2Cl2-MeOH 9:1 (3 x 100 ml), the combined organic phase was washed with distillated water (100 mL), dried with magnesium sulfate and the solvent was evaporated. The crude product was purified by preparative TLC (CH2Cl2-MeOH 10:1) and 25 mg of the product (18) was obtained which was immediately transformed to the sulfate salt. The base was dissolved in a mixture of CH2Cl2 (0.24 mL) and EtOH (0.16 mL), then 0.16 mL of a mixture of sulfuric acid (0.030 mmol)-EtOH was added and the mixture was evaporated to dryness. The solid product obtained was treated with Et2O and after filtration 24 mg (13%) of 14,15-cyclopropanovinblastine (18) sulfate was obtained as pale yellow crystals. Mp (sulfate) >280 °C. TLC (base) (CH2Cl2-MeOH 10:1), Rf = 0.39. TLC (sulfate) (CH2Cl2-MeOH 10:1), Rf = 0.39. IR (sulfate) (KBr) 3672, 3432, 2951, 1736, 1649, 1614, 1460, 1337 cm-1. 1H-NMR (800 MHz, D2O+CD3CN 1:1) δ (ppm) 0.67 (m, 1H, Hα-15); 0.90 (m, 1H, H2-22); 0.91 (t, J = 7.5 Hz, 3H, H3-18’); 1.03 (t, J = 7.0 Hz, 3H, H3-18); 1.21 (ABq, J = 14.3 Hz, J = 7.0 Hz, 1H, Hx-19); 1.39 (m, 1H, H-14’); 1.41 (m, 1H, Hα-14); 1.50 (m, 1H, H2-19’); 1.60 (dd, J = 14.6 Hz, J = 7.0 Hz, 1H, Hα-15’); 1.65 (d, J = 14.6 Hz, 1H, Hß-15’); 1.95 (ABq, J = 14.0 Hz, J = 7.3 Hz, 1H, Hy-19); 2.08 (s, 3H, C(17)OCOCH3); 2.04 (ddd, J = 14.4 Hz, J = 10.6 Hz, J = 4.3 Hz, 1H, Hα-6); 2.26 (dt, J = 14.4 Hz, J = 8.7 Hz, 1H, Hß-6); 2.32 (dd, J = 15.7 Hz, J = 4.4 Hz, 1H, Hα-17’); 2.65 (s, 3H, N(1)CH3); 2.85 (dd, J = 14.5 Hz, J = 6.0 Hz, 1H, Hα-3’); 2.98 (dd, J = 11.9 Hz, J = 3.3 Hz, 1H, Hα-3); 3.12 (td, J = 11.2 Hz, J = 9.6 Hz, 1H, Hα-5); 3.16 (s, 1H, H-21); 3.18 (m, 2H, H2-21’); 3.35 (dd, J = 16.9 Hz, J = 6.3 Hz, 1H, Hα-6’); 3.55 (s, 1H, H-2); 3.60 (m, 1H, Hß-5’); 3.62 (m, 1H, Hα-5’); 3.64 (s, 3H, C(16’)COOCH3); 3.64 (m, 1H, Hß-5); 3.67 (d br, J = 11.9 Hz, 1H, Hß-3); 3.80 (dd, J = 15.7 Hz, J = 14.2 Hz, 1H, Hß-17’); 3.82 (s, 3H, C(11)OCH3); 3.83 (s, 3H, C(16)COOCH3); 3.92 (d, J = 14.5 Hz, 1H, Hß-3’); 4.42 (dd, J = 16.9 Hz, J = 11.3 Hz, 1H, Hß-6’); 5.27 (s, 1H, H-17); 6.40 (s, 1H, H-12); 6.76 (s, 1H, H-9); 7.16 (m, 1H, H-10’); 7.23 (m, 1H, H-11’); 7.31 (d, J = 8.0 Hz, 1H, H-12’); 7.61 (d, J=8.0 Hz, 1H, H-9’); 8.75 (s, 1H, H-1’). 13C-NMR (200 MHz, D2O+CD3CN 1:1) δ (ppm) 6.1 (C-18’); 7.0 (C-22); 7.8 (C-18); 10.4 (C-14); 15.3 (C-15); 20.2 (C(17)OCOCH3); 20.5 (C-6’); 26.3 (C-14’); 34.0 (C-19’); 35.3 (C-17’); 35.4 (C-19); 36.1 (C-15’); 38.5 (C-1); 40.3 (C-20); 44.0 (C-6); 44.8 (C-3’); 51.1(C-3); 51.5 (C-5); 51.7 (C-7); 52.8 (C(16’)COOCH3); 53.4 (C(16)COOCH3); 55.2 br (C-16’); 56.1 (C(11)OCH3); 56.6 (C-5’); 60.6 (C-21’); 67.8 (C-20’); 68.5 (C-21); 75.2 (C-17); 78.5 (C-16); 80.9 (C-2); 94.8 (C-12); 111.3 (C-12’); 113.8 (C-7’); 118.2 (C-9’); 119.8 (C-10’); 121.1 br (C-10); 122.3 (C-8); 123.2 (C-11’); 123.5 (C-9); 128.7 (C-8’); 131.0 (C-2’); 135.7 (C-13’); 153.0 (C-13); 158.7 (C-11); 171.9 (C(17)OCOCH3); 172.3 (C(16)COOCH3); 175.5 (C(16’)COOCH3). HRMS: 825.43996 (C47H61O9N4/Mbase+H/; calc. 825.44331). ESI-MS-MS (CID = 25 %) (rel. int. %): 807(6); 765(100); 747(30); 715(5); 540(7); 522(9).
14,15-Cyclopropanovincristine, 19. 14,15-Cyclopropanovinblastine sulfate (18) (64 mg; 0.069 mmol) was dissolved in a mixture of acetone (14 mL) and acetic acid (3.2 mL), and at -60 °C CrO3 (31 mg; 0.31 mmol) in acetic acid anhydride (11.7 mL) was added to the mixture. After stirring for 8 min, 25% NH4OH at -50 °C was added to pH 9 keeping the temperature of the reaction mixture below 50 °C, and distilled water (50 mL) was added. The mixture was extracted with CH2Cl2 (5 x 20 mL), the combined organic phase was washed with water (2 x 30 mL), dried with magnesium sulfate and evaporated to dryness. Formic acid (0.95 ml) and acetic acid anhydride (0.16 mL) was added to the residue and after allowing the solution to stand at room temperature for 5 min distilled water (20 mL) was added and basified with 25% NH4OH to pH 9. The solution was extracted with CH2Cl2 (5 x 10 mL), the combined organic phase was dried with magnesium sulfate and the solvent was evaporated. The crude product was purified by preparative TLC (CH2Cl2-MeOH10:1) and 35 mg (0.042 mmol) of product (19) was obtained, which was transformed immediately to the sulfate salt. The product was dissolved in a mixture of CH2Cl2 (0.33 mL) and EtOH (0.21mL), then 0.23 mL of a mixture of sulfuric acid (0.042 mmol)-EtOH was added. The solution was evaporated to dryness and after treating the residue with Et2O 34 mg (52%) 14,15-cyclopropanovincristine sulfate was obtained as pale yellow crystals, which contained 10% of starting material. Mp (sulfate) >280 °C. TLC (base) (CH2Cl2-MeOH 10:1), Rf = 0.36. TLC (sulfate) (CH2Cl2-MeOH 10:1), Rf = 0.36. IR (sulfate) (KBr) 3425, 3059, 2954, 1750, 1689, 1617, 1493, 1434, 1373 cm-1. 1H-NMR (800 MHz, D2O+CD3CN 1:1) δ (ppm) 0.67 (m, 1H, Hα-15); 0.84 (m, 1H, Hx-22); 0.90 (t, J = 7.5 Hz, 3H, H3-18’); 1.08 (t, J = 7.0 Hz, 3H, H3-18); 1.14 (m, 1H, Hy-22); 1.17 (ABq, J = 14.3 Hz, J = 7.0 Hz, 1H, Hx-19); 1.43 (m, 1H, H-14’); 1.41 (m, 1H, Hα-14); 1.49 (m, 1H, H2-19’); 1.54 (dd, J = 14.6 Hz, J = 7.3 Hz, 1H, Hα-15’); 1.66 (d, J = 14.6 Hz, 1H, Hß-15’); 1.82 (ABq, J = 14.4 Hz, J = 7.3 Hz, 1H, Hy-19); 1.92 (m, 1H, Hß-6); 2.04 (s, 3H, C(17)OCOCH3); 2.31 (ddd, J = 14.1 Hz, J = 9.0 Hz, J = 5.3 Hz, 1H, Hα-6); 2.38 (d br, J = 14.5 Hz, 1H, Hα-17’); 2.76 (dd, J = 14.5 Hz, J = 6.3 Hz, 1H, Hα-3’); 3.03 (d br, J = 11.9 Hz, 1H, Hα-3); 3.16 (m, 1H, Hα-5); 3.17 (m, 2H, H2-21’); 3.37 (dd, J = 17.4 Hz, J = 6.7 Hz, 1H, Hα-6’); 3.44 (s, 1H, H-21); 4.50 (s, 1H, H-2); 3.55 (dd, J = 14.2 Hz, J = 10.5 Hz, 1H, Hα-5’); 3.62 (dd, J = 14.2 Hz, J = 6.6 Hz, 1H, Hß-5’); 3.67 (s, 3H, C(16)COOCH3); 3.68 (s, 3H, C(16’)COOCH3); 3.71 (m, 1H, Hß-3); 3.72 (m, 1H, Hß-5); 3.80 (m, 1H, Hß-17’); 3.83 (m, Hß-3’); 3.90 (s, 3H, C(11)OCH3); 4.48 (dd, J = 17.4 Hz, J = 11.8 Hz, 1H, Hß-6’); 5.02 (s, 1H, H-17); 7.08 (s, 1H, H-9); 7.16 (m, 1H, H-10’); 7.17 (s, 1H, H-12); 7.24 (m, 1H, H-11’); 7.36 (d, J = 8.2 Hz, 1H, H-12’); 7.62 (d, J = 8.1 Hz, 1H, H-9’); 8.88 (s, 3H, N(1)CHO); 8.94 (s, 1H, H-1’). 13C-NMR (200 MHz, D2O+CD3CN 1:1) δ (ppm) 6.8 (C-18’); 7.8 (C-22); 8.4 (C-18); 11.3 (C-14); 16.1 (C-15); 20.7 (C(17)OCOCH3); 21.0 (C-6’); 26.7 (C-14’); 34.7 (C-19’); 35.5 (C-17’); 36.4 (C-19); 36.4 (C-15’); 41.0 (C-20); 41.5 (C-6); 45.3 (C-3’); 51.4 (C-5); 51.9 (C-7); 52.5 (C-3); 53.6 (C(16’)COOCH3); 54.4 (C(16)COOCH3); 56.1 (C-16’); 57.1 (C(11)OCH3); 57.4 (C-5’); 61.4 (C-21’); 67.6 (C-21); 68.2 (C-20’); 71.0 (C-2); 75.8 (C-17); 78.3 (C-16); 96.8 (C-12); 112.3 (C-12’); 114.9 (C-7’); 119.0 (C-9’); 120.5 (C-10’); 123.5 (C-8); 124.0 (C-11’); 125.4 (C-9); 128.2 (C-10); 129.5 (C-8’); 130.6 (C-2’); 136.6 (C-13’); 141.8 (C-13); 159.1 (C-11); 163.9 (C-1); 172.0 (C(17)OCOCH3); 171.3 (C(16)COOCH3); 175.3 (C(16’)COOCH3). HRMS: 839.42334 (C47H59O10N4/ Mbase+H/; calc. 839.42257). ESI-MS-MS (CID=65 %) (rel. int. %): 821(49); 779(100); 761(22); 737(12); 719(25); 701(6); 618(4); 353(7).
ACKNOWLEDGEMENT
The authors are grateful to Gedeon Richter Plc for financial assistance.
References
1. A. Brossi and M. Suffness, Eds. “The Alkaloids”, Vol. 37, Academic Press Inc., New York, USA, 1990, pp. 1-240.
2. P. S. Sisodiya, Int. J. Res. Dev. Phar. L. Sci., 2013, 2, 293.
3. H. Bölcskei, L. Szabó, and Cs. Szántay, Frontiers Nat. Prod. Chem., 2005, 1, 43. CrossRef
4. P. Keglevich, L. Hazai, Gy. Kalaus, and Cs. Szántay, Molecules, 2012, 17, 5893. CrossRef
5. R. L. Noble, T. Beer, and R. W. McIntyre, Cancer, 1967, 20, 885. CrossRef
6. F. J. Weigert and J. D. Roberts, J. Am. Chem. Soc., 1967, 89, 5962. CrossRef
7. K. B. Wiberg, Acc. Chem. Res., 1996, 29, 229. CrossRef
8. W. A. Donaldson, Tetrahedron, 2001, 57, 8589. CrossRef
9. M. Tori, T. Hamaguchi, M. Aoki, M. Sono, and Y. Asakawa, Can. J. Chem., 1997, 75, 634. CrossRef
10. J. C. Lorenz, J. Long, Z. Yang, S. Yue, Y. Xie, and Y. Shi, J. Org. Chem., 2004, 69, 327. CrossRef
11. G. Brunner, S. Elmer, and F. Schröder, Eur. J. Org. Chem., 2011, 4623. CrossRef
12. M. Incze, G. Dörnyei, I. Moldvai, E. Temesvári-Major, O. Egyed, and Cs. Szántay, Tetrahedron, 2008, 64, 2924. CrossRef
13. C.-H. Wang, G.-C. Wang, Y. Wang, X.-Q. Zhang, X.-J. Huang, D.-M. Zhang, M.-F. Chen, and W.-C. Ye, Fitoterapia, 2012, 83, 765. CrossRef
14. J. P. Kutney, C. A. Boullet, L. S. L. Choi, W. Gustowski, M. McHugh, J. Nakano, T. Nikaido, H. Tsukamoto, G. M. Hewitt, and R. Suen, Heterocycles, 1988, 27, 613. CrossRef
15. J. P. Kutney, B. Botta, C. A. Boullet, C. A. Buschi, L. S. L. Choi, J. Golinski, M. Gomulka, G. M. Hewitt, G. Lee, M. McHugh, J. Nakano, T. Nikaido, J.-I. Onodera, I. Perez, P. Salisbury, M. Singh, R. Suen, and H. Tsukamoto, Heterocycles, 1988, 27, 629. CrossRef
16. J. P. Kutney, L. S. L. Choi, J. Nakano, and H. Tsukamoto, Heterocycles, 1988, 27, 1837. CrossRef
17. K. Honty, Á. Demeter, Cs. Szántay, Jr., M. Hollósi, P. Kolonits, and Cs. Szántay, Heterocycles, 1999, 50, 169. CrossRef
18. S. H. Ahn, M. W. Duffel, and J. P. N. Rosazza, J. Nat. Prod., 1997, 60, 1125. CrossRef
19. P. Keglevich, L. Á. Gorka-Kereskényi, L. Szabó, L. Hazai, M. Lengyel, Cs. Szántay, Jr., Zs. Sánta, Gy. Kalaus, and Cs. Szántay, Heterocycles, 2007, 71, 1553Hazai, Á. Gorka-Kereskényi, L. Péter, J. Gyenese, Zs. Lengyel, Gy. Kalaus, Zs. Dubrovay, M. Dékány, E. Orbán, I. Szabó, Z. Bánóczi, Cs. Szántay, Jr., and Cs. Szántay, Heterocycles, 2013, 87, 2299. CrossRef
20. Á. Gorka-Kereskényi, L. Szabó, L. Hazai, M. Lengyel, Cs. Szántay, Jr., Zs. Sánta, Gy. Kalaus, and Cs. Szántay, Heterocycles, 2007, 71, 1553. CrossRef
21. D. L. Boger, WO Patent 2011/103007, 2011 (Chem. Abstr., 2011, 155, 328440).
22. H. Ishikawa, D. A. Colby, S. Seto, P. Va, A. Tam, H. Kakei, T. J. Rayl, I. Hwang, and L. Boger, J. Am. Chem. Soc., 2009, 131, 4904. CrossRef
23. K. Jovanovics, K. Szász, Gy. Fekete, E. Bittner, E. Dezséri, and J. Éles, US Patent 3,899,493, 1975 (Chem. Abstr., 1975, 83, 179360).
24. A. Monks, D. A. Scudiero, P. Skehan, R. H. Shoemaker, K. Paull, D. Vistica, C. Hose, J. Lamgley, P. Cronise, A. Waigro-Wolff, M. Grog-Goodrich, H. Campbell, J. G. Mayo, and M. R. Boyd, J. Nat. Cancer Inst., 1991, 83, 757. CrossRef
25. M. C. Alley, D. A. Scudiero, A. Monks, M. L. Hursey, M. J. Czerwinski, D. L. Fine, B. J. Abbott, J. G. Mayo, R. H. Shoemaker, and M. R. Boyd, Cancer Res., 1988, 48, 589.
26. R. H. Shomaker, A. Monks, M. C. Alley, D. A. Scudiero, D. L. Fine, T. L. McLemore, B. J. Abbott, K. D. Paull, J. G. Mayo, and M. R. Boyd, Prog. Clin. Biol. Res., 1988, 276, 265.