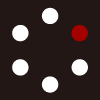
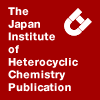
HETEROCYCLES
An International Journal for Reviews and Communications in Heterocyclic ChemistryWeb Edition ISSN: 1881-0942
Published online by The Japan Institute of Heterocyclic Chemistry
e-Journal
Full Text HTML
Received, 21st December, 2013, Accepted, 20th January, 2014, Published online, 28th January, 2014.
DOI: 10.3987/COM-13-12921
■ Enantioselective Total Synthesis of (–)-Scabronine D
Yu Kobayakawa and Masahisa Nakada*
Department of Chemistry and Biochemistry, School of Advanced Science and Engineering, Waseda University, 3-4-1 Okubo, Shinjuku-ku, Tokyo 169-8555, Japan
Abstract
The first enantioselective total synthesis of (–)-scabronine D comprising a unique bridged hemiacetal is described. The total synthesis is successfully accomplished using an enantiopure intermediate prepared in the total synthesis of (–)-scabronines G and A, and (–)-episcabronine A. Because the advanced intermediate was found to be sensitive to basic reaction conditions, the C14 keto and C11 hydroxy groups had to be co-protected as methyl acetal. In addition, both the C15 hydroxy and C17 carboxyl groups were too reactive to be chemically discriminated; hence, the carboxyl group once formed was protected as a tert-butyl ester for ensuring successful transformations.INTRODUCTION
Oshima and Ohta et al. isolated (–)-scabronine D (1) (Figure 1) as a component of various scabronines from Sarcodon scabrosus,1 a mushroom belonging to the Thelephoraceae having a strong bitter taste. (–)-Scabronine D (1) contains five stereogenic centers in its cyathane scaffold, which features a five–six–seven tricyclic ring system with trans-[6-7] ring fusion and two all-carbon quaternary stereogenic centers at the ring junctions. In addition, the unique oxygen-bridged hemiacetal structure formed between the C11 and C14 positions distinguishes it as the relatively complex structure in the scabronine family.
Certain scabronines demonstrate a potent induction effect upon the production and excretion of neurotrophic factors, including nerve growth factor (NGF) in 1321N1 human astroglial cells.1-3 NGF is a member of a family of small secreted proteins, which are important signaling molecules for the growth, maintenance, and survival of neural cells.4 NGF is naturally synthesized within the brain, indicating an endogenous mechanism for its production by small compounds that can cross the blood–brain barrier (BBB). Hence, research on compounds that stimulate endogenous NGF production has led to the isolation of natural products having this property.5 Consequently, the therapeutic relevance and synthetic challenges related to scabronines have evoked intense investigation by many research groups.
Although a number of synthetic studies on cyathane diterpenoids were conducted over the past decade,6-8 only three total syntheses of scabronines were reported.9,10 Recently, we reported the enantioselective total synthesis of (–)-scabronines G (2) and A (3), and (–)-episcabronine A (4) based on a biogenesis-inspired strategy.10 In the course of continued research on the collective and diverse total synthesis of scabronines for understanding their structure-activity relationships, we focused our interest on the structurally unique 1. We report herein the first enantioselective total syntheses of 1.
RESULTS AND DISCUSSION
Scheme 1 outlines our retrosynthetic analysis of 1 via 5, which can be prepared from 7 via 6 according to the synthetic route established in the total synthesis of 2–4.10
Pinnick oxidation of 6 (Scheme 2) and subsequent methyl ester formation afforded 8, followed by stereoselective dihydroxylation to give 9.11 The formation of 9’s diacetate failed, but the carbonate formation afforded 11 (α:β = 3.3:1), probably via the fast intramolecular reaction of 10. Treatment of 11 with DBU induced clean β-elimination to give 5, which was in equilibrium with 5’, but the 1H-NMR spectrum of 5 was time-dependent and varied gradually, indicating that the equilibrium was slow.
In contrast, the equilibrium of 12, which was obtained by the treatment of 5 with tetrabutylammonium fluoride (TBAF), was fast and the 12:12’ ratio was 1:1 in CDCl3 at 20 °C (Scheme 3). The difference between 5 and 12 could arise from the relatively rigid conformation of 5 with the pseudo-equatorial C11 hydroxy, which could be stabilized by the large C12 tert-butyldimethylsilyloxymethyl (TBDPSOCH2–) group (see the 3D structure of 12 which shows the same stable conformation as that of 5.). This implies that the conformation with the pseudo-axial C11 hydroxy would be energetically unfavorable but the conformation change of 12, which doesn’t have the large TBDPSOCH2– group at C12, could proceed smoothly when compared with 5, leading to the fast hemiacetal formation.
To accomplish the total synthesis of 1, the conversion of 12 to 1 was examined. Hydrolysis of the methyl ester did not proceed at room temperature under any condition. Therefore, heating was necessary for completion of the hydrolysis, but under the forced basic conditions resulted in the formation of only undesired products derived from the degradation of 12. For preventing the decomposition, the reactive C14 keto and C11 hydroxy groups in 12 were co-protected as the methyl acetal, i.e., 12 was converted to 13 using methanol-hydrogen chloride. Then, 13 was converted to the benzoate 14. Selective conversion of the methyl ester in 14 to the carboxyl group was examined under several conditions, i.e., AlCl3 and dimethylaniline,12 BCl3,13 Otera’s reagent,14 etc., but desired products were not obtained and debenzoylation occurred under all reaction conditions. In addition, selective benzoylation of the primary hydroxy group in 15 and subsequent hydrolysis of the methyl acetal was also examined, but the hydroxy group was not selectively benzoylated and instead, a mixed acid anhydride 16 was formed. The acid anhydride 16 was very stable during isolation and resisted hydrolysis, probably owing to the steric hindrance, and undesired debenzoylation occurred preferentially.
Consequently, we decided to protect the carboxyl group in 15 as a tert-butyl ester. Although the carboxyl group in 15 is highly hindered owing to the adjacent all-carbon quaternary center, the reaction of 15 with the reported reagent (N,N’-diisopropyl-O-tert-butyl isourea)15 (Scheme 4) successfully afforded 17. The reaction of 17 with benzoyl chloride under conventional conditions afforded 18 in 90% yield (three steps). The tert-butyl group in 18 was effectively removed by treatment with trifluoroacetic acid (TFA), but the methyl acetal remained intact. Therefore, after reaction completion, all volatile materials were removed under reduced pressure, and the residue was dissolved in THF and treated with 3N HCl at 60 °C. Fortunately, the methyl acetal in 19 was removed to afford a final product. Interestingly, the final product was obtained in only the hemiketal form, which spectroscopically matched the (–)-scabronine D reported by Oshima and Ohta et al.1 in all respects (i.e., 1H- and 13C-NMR, IR, HRMS, and [α]D). This confirms the formation of (–)-scabronine D (1). Oshima and Ohta et al. reported 1 as a yellowish oil, but the synthetic 1 solidified upon standing; hence, its melting point is reported accordingly.
In summary, the total synthesis of (–)-scabronine D was achieved for the first time. The methodology established in the total synthesis of (–)-scabronines G and A, and (–)-episcabronine A was successfully used for supplying the chiral intermediate to accomplish the enantioselective total synthesis of (–)-scabronine D, thus proving its robust utility for the collective, divergent, and enantioselective total syntheses of scabronines. The total synthesis herein reported was completed in 28 steps from 7, with 13% overall yield. Hence, this efficient total synthesis method would be useful for supplying (–)-scabronine D and congeners that have not been evaluated biologically, thereby promoting structure-activity relationship studies. Further synthetic studies and related biological studies are now underway, and the results will be reported in due course.
EXPERIMENTAL
1H and 13C NMR spectra were recorded on 400 MHz spectrometer. 1H and 13C chemical shifts are reported in ppm downfield from tetramethylsilane (TMS, δ scale) with the solvent resonances as internal standards. The following abbreviations were used to explain the multiplicities: s, singlet; d, doublet; t, triplet; q, quartet; m, multiplet; band, several overlapping signals; brs, broad. IR spectra were recorded on a FT/IR spectrometer. Mass spectra were provided at the Materials Characterization Central Laboratory, Waseda University. All reactions were carried out under an argon atmosphere with dry, freshly distilled solvents under anhydrous conditions, unless otherwise noted. All reactions were monitored by thin-layer chromatography carried out on 0.25 mm silica gel plates using UV light as visualizing agent and phosphomolybdic acid and heat as developing agents. Silica gel (60, particle size 0.040-0.063 mm) was used for flash chromatography. Preparative thin-layer chromatography (PTLC) separations were carried out on self-made 0.3 mm silica gel plates. THF was distilled from sodium/benzophenone ketyl. Toluene was distilled from sodium. MeOH was distilled with a small amount of magnesium and I2. All commercially available reagents were used without further purification. Optical rotations were measured on a polarimeterat a wavelength of 589 nm. High resolution mass spectra (HRMS) were obtained by either an electronspray ionization (ESI) or a fast atom bombardment (FAB), and theoretical monoisotopic molecular masses were typically ≤5 ppm. Melting point was uncorrected. TLC Rfs of purified compounds were included.
(–)-(3aS,5aR,7Z,9R,10aR)-2,3,3a,4,5,5a,6,9,10,10a-decahydro-9-hydroxy-8-hydroxymethyl-1-isopropyl-5a-methyl-6-oxocyclohepta[e]indene-3a-carboxylic acid methyl ester (12). To a stirred solution of 5 (146.9 mg, 0.244 mmol) and NH4Cl (65.3 mg, 1.22 mmol, 5.0 equiv) in THF (4.0 mL) was added TBAF (1.0 M in THF, 0.73 mL, 0.73 mmol, 3.0 equiv) dropwise at 0 °C, and the reaction mixture was stirred at room temperature for 30 min. After the reaction was completed, the reaction mixture was quenched by adding saturated aqueous NH4Cl solution (5 mL), and the aqueous layer was extracted with EtOAc (20 mL × 4). The combined organic layer was washed with water (20 mL), brine (20 mL), dried over Na2SO4, and evaporated. The residue was purified by flash silica gel chromatography (hexane/EtOAc = 4/1 to 1/2) to afford 12 (78.7 mg, 99%) as a colorless oil: Rf = 0.31 (hexane/EtOAc = 1/3); 1H NMR (400 MHz, CDCl3) δ 5.94 (0.5H, s), 5.91 (0.5H, s), 4.71 (0.5H, s), 4.64 (0.5H, br), 4.42 (0.5H, d, J = 15.6 Hz), 4.28-4.12 (2H, m), 3.65 (1.5H, s), 3.60 (1.5H, s), 2.96 (1H, sept, J = 6.9 Hz), 2.62 (0.5H, dd, J = 13.3, 5.5 Hz), 2.49-2.18 (4.5H, m), 2.13-1.85 (1.5H, m), 1.71-1.37 (4H, m), 1.32-1.22 (1H, m), 1.10 (1.5H, s), 1.06 (1.5H, d, J = 6.9 Hz), 1.04 (1.5H, s), 1.02 (1.5H, d, J = 6.9 Hz), 1.00 (1.5H, d, J = 6.9 Hz), 0.94 (1.5H, d, J = 6.9 Hz); 13C NMR (100 MHz, CDCl3) δ 209.7, 178.0, 177.5, 153.3, 147.6, 144.6, 144.2, 131.7, 130.8, 126.0, 123.0, 110.4, 78.5, 72.0, 65.1, 61.6, 60.4, 58.4, 53.7, 52.0, 51.8, 41.6, 41.5, 40.7, 36.1, 35.7, 35.5, 34.0, 33.2, 32.0, 31.6, 30.5, 30.0, 27.0, 26.5, 26.2, 22.1, 21.7, 21.3, 21.1, 15.4, 11.9; IR (ATR) νmax 3396(br), 2948, 2866, 1722, 1438, 1163, 977, 735 cm-1; HRMS (ESI) [M+Na]+ calcd for C21H30NaO5: 385.1985, found: 385.1986; [α]D22 –66.2 (c 0.12, CHCl3).
(–)-(3aS,5aR,6R,7Z,9R,10aR)-2,3,3a,4,5,5a,6,9,10,10a-decahydro-8-hydroxymethyl-1-isopropyl-6-methoxy-5a-methyl-6,9-epoxycyclohepta[e]indene-3a-carboxylic acid methyl ester (13). To a stirred solution of HCl in MeOH (8.0 mL, prepared by adding AcCl (2 drops) to MeOH (8 mL)) was added a solution of 12 (76.0 mg, 0.233 mmol) in MeOH (2.0 mL), and the resulting mixture was stirred at room temperature for 17 h. After the reaction was completed, the reaction was quenched by adding Et3N (0.5 mL) at 0 °C, and all the volatile materials were evaporated. The residue was purified by flash silica gel chromatography (hexane/EtOAc = 2/1) to afford 13 (85.9 mg, 98%) as a colorless oil: Rf = 0.83 (hexane/EtOAc = 1/3); 1H NMR (400 MHz, CDCl3) δ 6.00 (1H, s), 4.77 (1H, s), 4.29 (2H, s), 3.61 (3H, s), 3.30 (3H, s), 3.01 (1H, sept, J = 6.9 Hz), 2.45-2.23 (5H, m), 2.11 (1H, ddd, J = 12.8, 7.8, 2.3 Hz), 1.63-1.51 (4H, m), 1.45 (1H, ddd, J = 13.3, 4.6, 4.1 Hz), 1.23 (1H, ddd, J = 13.3, 4.6, 4.1 Hz), 1.03 (3H, d, J = 6.9 Hz), 1.02 (3H, s), 0.95 (3H, d, J = 6.9 Hz); 13C NMR (100 MHz, CDCl3) δ 177.9, 148.3, 144.5, 131.0, 124.2, 114.2, 78.3, 60.4, 59.0, 51.8, 51.1, 41.7, 41.6, 36.1, 33.4, 31.5, 30.5, 26.6, 26.5, 22.1, 21.2, 12.3; IR (ATR) νmax 3461(br), 2936, 2866, 1722, 1440, 989, 736, 667 cm-1; HRMS (ESI) [M+Na]+ calcd for C22H32NaO5: 399.2142, found: 399.2142; [α]D22 –62.0 (c 0.08, CHCl3).
(–)-(3aS,5aR,6R,7Z,9R,10aR)-8-(benzoyoxy)-2,3,3a,4,5,5a,6,9,10,10a-decahydro-1-isopropyl-6-methoxy-5a-methyl-6,9-epoxycyclohepta[e]indene-3a-carboxylic acid tert-butyl ester (18). To a stirred solution of 13 (39.2 mg, 0.104 mmol) in MeOH (1.0 mL) was added aqueous NaOH solution (2M, 1.0 mL), and the reaction mixture was stirred at 60 °C for 24 h. After the reaction was completed, to the reaction mixture was added aqueous HCl solution (2M, 1.02 mL) at 0 °C. After the addition was completed, the reaction mixture was extracted with CH2Cl2 (20 mL × 3). The combined organic layer was washed with brine (30 mL), dried over Na2SO4, and evaporated. The residue was filtered through a short plug of silica gel to afford crude acid, which was used for the next step without further purification. To a stirred solution of the crude acid in CH2Cl2 (5.0 mL) was added N,N’-diisopropyl-O-tert-butyl isourea (62.5 mg, 0.312 mmol, 3.0 equiv) at room temperature, and the reaction mixture was refluxed for 20 h. After the reaction was completed, all the volatile materials were evaporated. The residue was filtered through a short plug of silica gel to afford the crude tert-butyl ester, which was used for the next step without further purification. To a stirred solution of the crude tert-butyl ester in CH2Cl2 (1.0 mL) was added DMAP (25.4 mg, 0.208 mmol, 2.0 equiv) and BzCl (1.4×10-2 mL, 0.125 mmol, 1.2 equiv) at 0 °C, and the reaction mixture was stirred at 0 °C for 2 h. After the reaction was completed, the reaction mixture was quenched by adding saturated aqueous NH4Cl solution (2 mL), and the aqueous layer was extracted with EtOAc (5 mL × 2). The combined organic layer was washed with water (5 mL), brine (5 mL), dried over Na2SO4, and evaporated. The residue was purified by flash silica gel chromatography (hexane/EtOAc = 20/1) to afford 18 (49.0 mg, 90% (3 steps)) as a colorless oil: Rf = 0.85 (hexane/EtOAc = 1/1); 1H NMR (400 MHz, CDCl3) δ 8.04 (2H, d, J = 8.2 Hz), 7.58 (1H, t, J = 7.3 Hz), 7.45 (2H, dd, J = 8.2, 7.3 Hz), 6.16 (1H, s), 4.99 (1H, d, J = 14.7 Hz), 4.89 (1H, d, J = 14.7 Hz), 4.82 (1H, s), 3.32 (3H, s), 3.02 (1H, sept, J = 6.9 Hz), 2.47-2.22 (5H, m), 2.04 (1H, ddd, J = 13.3, 8.7, 2.3 Hz), 1.68-1.42 (3H, m), 1.41-1.24 (2H, m), 1.30 (9H, s), 1.03 (3H, d, J = 6.9 Hz), 1.02 (3H, s), 0.94 (3H, d, J = 6.9 Hz); 13C NMR (100 MHz, CDCl3) δ 176.3, 166.1, 144.1, 142.9, 133.3, 131.5, 129.7, 129.5, 128.5, 126.9, 114.3, 79.3, 78.5, 60.9, 60.5, 51.3, 41.9, 41.5, 36.0, 32.8, 31.5, 30.6, 27.8, 26.5, 26.5, 21.9, 21.4, 12.2; IR (ATR) νmax 2936, 2867, 1716, 1451, 1150, 990, 850, 710, 616 cm-1; HRMS (ESI) [M+Na]+ calcd for C32H42NaO6: 545.2874, found: 545.2863; [α]D22 –34.3 (c 0.50, CHCl3).
(–)-scabronine D (1). To a stirred solution of 18 (35.0 mg, 6.70×10-2 mmol) in CH2Cl2 (3.0 mL) was added TFA (1.5 mL) at 0 °C, and the reaction mixture was stirred at room temperature for 1 h. After the reaction was completed, the solvent was evaporated. To the residue was added THF (8 mL) and aqueous HCl solution (3M, 4.0 mL) at room temperature, and the reaction mixture was stirred at 60 °C for 24 h. After the reaction was completed, the reaction mixture was cooled to room temperature, and extracted with CH2Cl2 (10 mL × 3). The combined organic layer was washed with water (30 mL), brine (30 mL), dried over Na2SO4, and evaporated. The residue was purified by flash chromatography (CHCl3/MeOH = 100/1) to afford (–)-scabronine D (1) (25.2 mg, 83% (2 steps)) as a colorless solid: Rf = 0.44 (hexane/EtOAc = 1/1); 1H NMR (400 MHz, CDCl3) δ 8.00 (2H, d, J = 7.3 Hz), 7.55 (1H, t, J = 7.3 Hz), 7.42 (2H, t, J = 7.3 Hz), 6.09 (1H, s), 4.92 (2H, s), 4.77 (1H, s), 2.95 (1H, sept, J = 6.9 Hz), 2.52-2.36 (2H, m), 2.38-2.22 (3H, m), 2.19-2.05 (1H, m), 1.71-1.19 (5H, m), 1.07 (3H, s), 1.01 (3H, d, J = 6.9 Hz), 0.87 (3H, d, J = 6.9 Hz); 13C NMR (100 MHz, CDCl3) δ 181.9, 166.1, 145.6, 142.7, 133.3, 130.5, 129.7(2C), 129.5, 128.5, 110.1, 79.8, 60.3, 60.0, 41.7, 41.5, 36.5, 32.7, 31.6, 30.6, 26.7, 26.5, 22.0, 21.1, 11.8; IR (ATR) νmax 3362(br), 2927, 2854, 1718, 1695, 1451, 1267, 1109, 711 cm-1; HRMS (ESI) [M+Na]+ calcd for C27H32NaO6: 475.2091, found: 475.2092; [α]D22 –53.9 (c 0.37, MeOH); mp 79.2–81.4 °C.
ACKNOWLEDGEMENTS
This work was financially supported in part by Waseda University Grant for Special Research Projects, The Grant-in-Aid for Scientific Research on Innovative Areas “Organic Synthesis based on Reaction Integration, Development of New Methods and Creation of New Substances” (No. 2105), grant for Scientific Research (B) (25293003), and Grants for Excellent Graduate Schools (Practical Chemical Wisdom), MEXT, Japan. The fellowship for young scientists to Y. K. from JSPS (5515) is also gratefully acknowledged.
References
1. Scabronines B-F: T. Kita, T. Y. Takaya, Y. Oshima, T. Ohta, K. Aizawa, T. Hirano, and T. Inakuma, Tetrahedron, 1998, 54, 11877.
2. Scabronine A: (a) T. Ohta, T. Kita, N. Kobayashi, Y. Obara, N. Nakahata, Y. Ohizumi, Y. Takaya, and Y. Oshima, Tetrahedron Lett., 1998, 39, 6229; CrossRef Scabronine G: (b) Y. Obara, H. Kobayashi, T. Ohta, Y. Ohizumi, and N. Nakahata, Mol. Pharmacol., 2001, 59, 1287; For episcabronine A: (c) T. Ohta, H. Kobayashi, S. Hosoi, F. Kiuchi, T. Kita, Y. Takaya, Y. Oshima, Y. Obara, N. Nakahata, and Y. Ohizumi, Tennen Yuki Kagobutsu Toronkai Koen Yoshishu, 1998, 40th, 341.
3. (a) Y. Obara, N. Nakahata, T. Kita, Y. Takaya, H. Kobayashi, S. Hosoi, F. Kiuchi, T. Ohta, Y. Oshima, and Y. Ohizumi, Eur. J. Pharmacol., 1999, 370, 79; CrossRef (b) X.-W. Shi, L. Liu, J.-M. Gao, and A.-L. Zhang, Eur. J. Med. Chem., 2011, 46, 3112. CrossRef
4. (a) X. He and K. C. Garcia, Science, 2004, 304, 870; CrossRef (b) M. V. Sofroniew, C. L. Howe, and W. C. Mobley, Annu. Rev. Neurosci., 2001, 24, 1217; CrossRef (c) R. M. Lindsay, J. Neurosci., 1988, 8, 2394.
5. S. J. Pollack and S. J. Harper, Curr. Drug Targets CNS & Neurol. Disord., 2002, 1, 59. CrossRef
6. For reviews see: (a) D. L. Wright and C. R. Whitehead, Org. Prep. Proced. Int., 2000, 32, 307; CrossRef (b) J. A. Enquist, Jr. and B. M. Stoltz, Nat. Prod. Rep., 2009, 26, 661; CrossRef (c) K. Kim and J. K. Cha, Angew. Chem. Int. Ed., 2009, 48, 5334; CrossRef (d) E. Elamparuthi, C. Fellay, M. Neuburger, and K. Gademann, Angew. Chem., Int. Ed., 2012, 51, 4071. CrossRef
7. Synthetic studies: (a) W. A. Ayer, D. E. Ward, L. M. Browne, L. T. J. Delbaere, and Y. Hoyano, Can. J. Chem., 1981, 59, 2665; CrossRef (b) D. E. Ward, Can. J. Chem., 1987, 65, 2380; CrossRef (c) K. R. Dahnke and L. A. Paquette, J. Org. Chem., 1994, 59, 885; CrossRef (d) E. Piers and K. L. Cook, Chem. Commun., 1996, 1879; CrossRef (e) P. Magnus and L. Shen, Tetrahedron, 1999, 55, 3553; CrossRef (f) D. L. Wright, C. R. Whitehead, E. H. Sessions, I. Ghiviriga, and D. A. Frey, Org. Lett., 1999, 1, 1535; CrossRef (g) K. Takeda, D. Nakane, and M. Takeda, Org. Lett., 2000, 2, 1903; CrossRef (h) P. A. Wender, F. C. Bi, M. A. Brodney, and F. Gosselin, Org. Lett., 2001, 3, 2105; CrossRef (i) C. Thominiaux, A. Chiaroni, and D. Desmaele, Tetrahedron Lett., 2002, 43, 4107; CrossRef (j) H. Watanabe and M. Nakada, Tetrahedron Lett., 2008, 49, 1518. CrossRef
8. Total synthesis: (±)-Allocyathin B2: (a) B. B. Snider, N. H. Vo, S. V. O'Neil, and B. M. Foxman, J. Am. Chem. Soc., 1996, 118, 7644; CrossRef (b) B. B. Snider, N. H. Vo, and S. V. O'Neil, J. Org. Chem., 1998, 63, 4732; CrossRef (c) M. Tori, N. Toyoda, and M. Sono, J. Org. Chem., 1998, 63, 306; CrossRef (±)-Sarcodonin G: (d) E. Piers, M. Gilbert, and K. L. Cook, Org. Lett., 2000, 2, 1407; CrossRef (±)-Allocyathin B3: (e) D. E. Ward, Y. Gai, and Q. Qiao, Org. Lett., 2000, 2, 2125; CrossRef (f) D. E. Ward, Y. Gai, Q. Qiao, and J. Shen, Can. J. Chem., 2004, 82, 254; CrossRef (+)-Allocyathin B2: (g) M. Takano, A. Umino, and M. Nakada, Org. Lett., 2004, 6, 4897; CrossRef (h) B. M. Trost, L. Dong, and G. M. Schroeder, J. Am. Chem. Soc., 2005, 127, 2844; CrossRef (i) B. M. Trost, L. Dong, and G. M. Schroeder, J. Am. Chem. Soc., 2005, 127, 10259; CrossRef (+)-Cyanthiwigin U: (j) M. W. B. Pfeiffer, and A. J. Phillips, J. Am. Chem. Soc., 2005, 127, 5334; CrossRef (–)-Erinacine B: (k) H. Watanabe, M. Takano, A. Umino, T. Ito, H. Ishikawa, and M. Nakada, Org. Lett., 2007, 9, 359; CrossRef (–)-Cyathin A3: (l) D. E. Ward and J. Shen, Org. Lett., 2007, 9, 2843; CrossRef (–)-Erinacine E: (m) H. Watanabe and M. Nakada, J. Am. Chem. Soc., 2008, 130, 1150; CrossRef (–)-Cyanthiwigin F: (n) J. A. Enquist, Jr. and B. M. Stoltz, Nature, 2008, 453, 1228; CrossRef (±)-Cyathin A3 and B2: (o) K. Kim and J. K. Cha, Angew. Chem. Int. Ed., 2009, 48, 5334; CrossRef (+)-Cyrneine A: (p) E. Elamparuthi, C. Fellay, M. Neuburger, and K. Gademnn, Angew. Chem. Int. Ed., 2012, 51, 4071. CrossRef
9. (a) S. P. Waters, Y. Tian, Y.-M. Li, and S. J. Danishefsky, J. Am. Chem. Soc., 2005, 127, 13514; CrossRef (b) N. Kanoh, K. Sakanishi, E. Iimori, K. Nishimura, and Y. Iwabuchi, Org. Lett., 2011, 13, 2864. CrossRef
10. Y. Kobayakawa and M. Nakada, Angew. Chem. Int. Ed., 2013, 52, 7569. CrossRef
11. The relative stereochemistry of the major isomer was determined by NOESY analysis of its carbonate 11.
12. T. Akiyama, H. Hirofuji, A. Hirose, and S. Ozaki, Synth. Commun., 1994, 24, 2179. CrossRef
13. P. S. Manchand, J. Chem. Soc. D, 1971, 667. CrossRef
14. J. Otera, H. Danoh, and H. Nozaki, J. Org. Chem., 1991, 56, 5307. CrossRef
15. (a) E. Vowinkel, Chem. Ber., 1967, 100, 16; CrossRef (b) K. R. West, K. D. Bake, and S. Otto, Org. Lett., 2005, 7, 2615. CrossRef