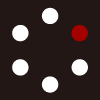
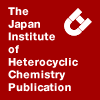
HETEROCYCLES
An International Journal for Reviews and Communications in Heterocyclic ChemistryWeb Edition ISSN: 1881-0942
Published online by The Japan Institute of Heterocyclic Chemistry
e-Journal
Full Text HTML
Received, 27th February, 2014, Accepted, 19th March, 2014, Published online, 2nd April, 2014.
DOI: 10.3987/COM-14-S(K)13
■ 2-Aryl-4-quinolone Synthesis Using the Thermal Rearrangement of Iminocyclobutenones
Iwao Hachiya, Keiichi Yokoyama, Akinori Ito, and Makoto Shimizu*
Department of Chemistry for Materials, Graduate School of Engineering, Mie University, 1577 Kurimamachiya-cho Tsu, Mie 514-8507, Japan
Abstract
2-Aryl-4-quinolone synthesis is developed using the thermal rearrangement of iminocyclobutenones formed by a conjugate addition reaction of ketene silyl acetals to alkynyl imines.Since the discovery of nalidixic acid as an antimicrobial agent in 1962, several 4-quinolone derivatives have been investigated as a chemotherapeutic agent. While 4-quinolone derivatives possessing a 3-carboxy group such as norfloxacin, ciprofloxacin, and levofloxacin have been introduced (Scheme 1),1 2-aryl-4-quinolones are also significant biologically active compounds because of antimitotic, antiplatelet, and antiviral activities. Therefore, numerous methods for the synthesis of 2-aryl-4-quinolone derivatives have been reported.2
Alkynyl imines are some of the most useful nitrogen-containing starting materials and extensively used in the synthesis of nitrogen-containing compounds including heterocycles.3 We have been interested in the reactivity at the β-position of alkynyl imines as a Michael acceptor and developed efficient synthetic methods for several nitrogen containing heterocycles such as 2-pyridones,4 bicyclo-pyridones,5 iminopyridines,6 and aminopyridines.6 During these investigations, we have found that aluminum chloride promoted conjugate addition reactions of alkynyl imines 1 with ketene silyl acetals 2 proceed to give the iminocyclobutenones 3 in good yields. Furthermore, it has been also developed that chemoselective reduction of iminocyclobutenones 3 proceeds to give the aminocyclobutenones 4 in high yields, and the subsequent thermal rearrangement of aminocyclobutenone 4 in the presence of an appropriate amine affords either cis- or trans-β-lactams 7 in good yields and with high diastereoselectivities (Scheme 2).7 We propose a plausible reaction mechanism of the thermal rearrangement of aminocyclobutenones 4 via aminoketene 5, which is considered to be one of the intermediates in the Kinugasa reaction.8 Aminoketene 5 would be generated by ring opening reaction of aminocyclobutenone 4 and undergoes a cyclization to give the enol 6. Protonation of the enol 6 and/or concomitant epimerization would occur in the presence of an appropriate amine to afford either cis- or trans-β-lactams 7 with high diastereoselectivities.
We next envisioned that a ring opening reaction of iminocyclobutenone 3 followed by a cyclization of iminoketene 8’ would give 4-quinolone 10 via an intermediate 9 (Scheme 3). Although several 4-quinolone syntheses via iminoketene intermediates have already been reported, there still remain some drawbacks such as a limited substrate scope and/or forcing cyclization conditions at high temperatures.9 Herein, this paper describes the 2-aryl-4-quinolone synthesis using the thermal rearrangement of iminocyclobutenones.10
We first examined reaction time in the thermal rearrangement of iminocyclobutenone 3a in toluene at reflux. When the reaction was carried out for 3 h, the desired 4-quinolone 4a was obtained in 94% yield (Table 1, entries 1-4).11 Several iminocyclobutenones 3b-h were subjected to the thermal rearrangement conditions. The reaction of diphenylated iminocyclobutenone 3b gave the 4-quinolone 10b in 95% yield (entry 5). The reaction of iminocyclobutenone 3c having a 2-furyl group as a hetero aromatic group also afforded 4-quinolone 10c in 91% yield (entry 6). When iminocyclobutenones 3d and 3e possessing a 2-naphthyl group at the R2 moiety were used, both yields slightly decreased probably due to the steric bulkiness (entries 7 and 8). The reactions of the iminocyclobutenones 3f and 3g possessing a 2-thienyl or a 2-naphthyl group at the R1 moiety gave 4-quinolones 10e and 10f in high yields, respectively (entries 9 and 10). When the iminocyclobutenone 3h having an ethyl group at the R3 moiety was carried out in toluene at reflux for 5 h, 4-quinolone 10h was obtained in 68% yield along with the recovered iminocyclobutenone 3h in 29% yield (entry 11). The reaction of 3h was next carried out in xylenes at reflux for 5 h to consume the remaining iminocyclobutenone 3h. The reaction proceeded to reach completion to afford 4-quinolone 10h in 86% yield (entry 12).
We next examined the effects of the substituents on a phenyl group at the nitrogen atom, and Table 2 summarizes the results. The reactions of N-phenylated iminocyclobutenones 11a and 11b gave 4-quinolones 12a and 12b in 93% and 98% yields, respectively (entries 1 and 2). When para-halophenylated iminocyclobutenones 11c-e were used, 4-quinolones 12c-e were obtained in high yields irrespective of the kind of the halogen (entries 3-5). The reaction of ortho-methoxyphenylated iminocyclobutenone 11f gave 4-quinolone 12f in quantitatively yield (entry 6), whereas use of 2,4-disubstituted iminocyclobutenone 11g decreased the yield (entry 7).
We next examined the thermal rearrangement of iminocyclobutenones 13 having meta-substituents on a phenyl group at the nitrogen atom. These iminocyclobutenones have two reaction sites on the phenyl moiety to form 4-quinolones 14 or 15 (Scheme 4).
Table 3 summarizes the results. The reaction of iminocyclobutenones 13a and 13b having an electron-withdrawing group such as fluoro or bromo group afforded a mixture of 4-quinolones 14 and 15 in high yields and with a moderate preference of 7-substituted-4-quinolone 14 (entries 1 and 2). On the other hand, use of iminocyclobutenone 13c having an electron-donating group such as a methyl group gave a mixture of 4-quinolones 14c and 15c in high yields and with a slight 5-substituted-4-quinolone 15 selectivity (entry 3). The reason of the reversal of the selectivity is not yet clear at the present stage.
In conclusion, we have developed a 2-aryl-4-quinolone synthesis using the thermal rearrangement of iminocyclobutenones. The present 2-aryl-4-quinolone synthesis is an attractive alternative method because iminocyclobutenones are readily available using a conjugate addition reaction of ketene silyl acetals to alkynyl imines and thermal rearrangements occur under mild reaction conditions to afford the corresponding 2-aryl-4-quinolones in high yields.
ACKNOWLEDGEMENTS
This work was supported by Grants-in Aid for Scientific Research (B), (C) and Innovative Areas “Organic Synthesis Based on Reaction Integration. Development of New Methods and Creation of New Substances” from JSPS and MEXT.
References
1. For reviews on 4-quinolone agents and synthesis, see: (a) L. A. Mitscher, Chem. Rev., 2005, 105, 559; CrossRef (b) A. A. Beteva and O. P. Krasnykh, Chem. Heterocycl. Comp., 2009, 45, 757. CrossRef
2. For recent examples of the 2-aryl-4-quinolone synthesis, see: (a) Y. J. Song, J. S. Choi, and J. I. Lee, Bull. Korean Chem. Soc., 2013, 34, 3117; CrossRef (b) W. Yang, L. Xu, Z. Chen, L. Zhang, M. Miao, and H. Ren, Org. Lett., 2013, 15, 1282; CrossRef (c) L. Klier, T. Bresser, T. A. Nigst, K. Karaghiosoff, and P. Knochel, J. Am. Chem. Soc., 2012, 134, 13584; CrossRef (d) F. Sun, X. Zhao, and D. Shi, Tetrahedron Lett., 2011, 52, 5633; CrossRef (e) T. Zhao and B. Xu, Org. Lett., 2010, 12, 212; CrossRef (f) A. Romek and T. Opatz, Eur. J. Org. Chem., 2010, 5841; CrossRef (g) Y. Yoshino, T. Kurahashi, and S. Matsubara, J. Am. Chem. Soc., 2009, 131, 7494; CrossRef (h) J. Huang, Y. Chen, A. O. King, M. Dilmeghani, R. D. Larsen, and M. M. Faul, Org. Lett., 2008, 10, 2609; CrossRef (i) P. Hradil, M. Grepl, J. Hlavác, M. Soural, M. Malon, and V. Bertolasi, J. Org. Chem., 2007, 72, 7968; CrossRef (j) C. P. Jones, K. W. Anderson, and S. L. Buchwald, J. Org. Chem., 2007, 72, 7968 and references therein. CrossRef
3. For recent examples of alkynyl imines, see: (a) T. Kano, T. Yurino, and K. Maruoka, Angew. Chem. Int. Ed., 2013, 52, 11509; CrossRef (b) G. Cheng and X. Cui, Org. Lett., 2013, 15, 1480; CrossRef (c) F. Sha, L. Wu, and X. Huang, J. Org. Chem., 2012, 77, 3754; CrossRef (d) C. Olier, N. Azzi, G. Gil, S. Gastaldi, and M. P. Bertrand, J. Org. Chem., 2008, 73, 8469 and references therein. CrossRef
4. (a) I. Hachiya, K. Ogura, and M. Shimizu, Org. Lett., 2002, 4, 2755; CrossRef (b) I. Hachiya, K. Ogura, and M. Shimizu, Synthesis, 2004, 1349; (c) I. Hachiya, S. Fukushima, and M. Shimizu, Heterocycles, 2006, 69, 43. CrossRef
5. (a) I. Hachiya, M. Atarashi, and M. Shimizu, Heterocycles, 2006, 67, 523; CrossRef (b) I. Hachiya, W. Maehara, Y. Yamada, T. Kamiki, and M. Shimizu, Synlett, 2006, 3271.
6. I. Hachiya, Y. Minami, T. Aramaki, and M. Shimizu, Eur. J. Org. Chem., 2008, 1411. CrossRef
7. I. Hachiya, T. Yoshitomi, Y. Yamguchi, and M. Shimizu, Org. Lett., 2009, 11, 3266. CrossRef
8. (a) B. Baeza, L. Casarrubios, and M. A. Sierra, Chem. Eur. J., 2013, 19, 11536; CrossRef (b) X. Zhang, R. P. Hsung, H. Li, Y. Zhang, W. L. Johnson, and R. Figueroa, Org. Lett., 2008, 10, 3477; CrossRef (c) M.-C. Ye, J. Zhou, and Y. Tang, J. Org. Chem., 2006, 71, 3576; CrossRef (d) R. Shintani and G. C. Fu, Angew. Chem. Int. Ed., 2003, 42, 4082. CrossRef
9. For examples of the 4-quinolone synthesis via iminoketene intermediates, see: (a) K. T. Potts, R. Ehlinger, and W. M. Nichols, J. Org. Chem., 1975, 40, 2596; CrossRef (b) B. E. Fulloon and C. Wentrup, J. Org. Chem., 1996, 61, 1363; CrossRef (c) L. George, K.-P. Netsch, G. Penn, G. Kollenz, and C. Wentrup, Org. Biomol. Chem., 2006, 4, 558 and references therein. CrossRef
10. Iminocyclobutenones were prepared using conjugate addition reactions of alkynyl imines with ketene silyl acetals according to the literature method in reference 7.
11. Typical procedure (Table 1, entry 1): A solution of iminocyclobutenone 3a (38.7 mg, 0.0999 mmol) in toluene (1.0 mL) was stirred at reflux for 3 h. After cooling to room temperature, the solvent was removed in vacuo and then the residue was purified by preparative TLC on silica gel (CH2Cl2/MeOH = 19/1 as an eluent) to give 4-quinolone 10a (36.2 mg, 94%). Yellow white solid; Mp 279-280 °C; 1H NMR (500 MHz, CDCl3-(CD3)2SO (9:1)) δ 1.72 (s, 3H), 1.80 (s, 3H), 3.86 (s, 3H), 6.99-7.07 (m, 5H), 7.11 (dd, J = 3.1, 5.5 Hz, 1H), 7.19 (dd, J = 3.1, 9.2 Hz, 1H), 7.40 (d, J = 3.1 Hz, 1H), 7.58 (d, J = 5.5 Hz, 1H), 7.62 (d, J = 3.1 Hz, 1H), 7.66 (d, J = 9.2 Hz, 1H), 11.4 (s, 1H); 13C NMR (126 MHz, CDCl3-(CD3)2SO) δ 20.1, 21.1, 53.8, 102.9, 118.7, 120.3, 120.8, 124.0, 124.0, 125.1, 125.6, 127.4, 127.6, 128.0, 133.2, 133.9, 134.2, 139.2, 140.8, 154.2, 173.8; IR (KBr) 3160, 3060, 2997, 2966, 2927, 2848, 1614, 1571, 1540, 1491, 1436, 1369, 1342, 1291, 1258, 1228, 1164, 1113, 1029, 1053, 832, 794, 765, 735, 694 cm-1; HRMS (EI): Calcd for C24H21NO2S (M)+ 387.1293, found 387.1308.