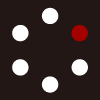
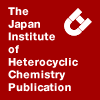
HETEROCYCLES
An International Journal for Reviews and Communications in Heterocyclic ChemistryWeb Edition ISSN: 1881-0942
Published online by The Japan Institute of Heterocyclic Chemistry
e-Journal
Full Text HTML
Received, 17th July, 2014, Accepted, 30th July, 2014, Published online, 6th August, 2014.
DOI: 10.3987/COM-14-S(K)85
■ Synthetic Studies toward Welwitindolinone Alkaloids. Tandem Aldol–Michael Reaction to Form the Carbocyclic Core of Welwitindolinones
Masato Shima and Masahiro Toyota*
Team TOYOTA, Graduate School of Science, Osaka Prefecture University, 1-1 Gakuencho, Sakai, Osaka 593-8531, Japan
Abstract
A tandem aldol ̶Michael reaction effectively constructed a bridged ketone, which is the carbocyclic core of the welwitindolinone family.In 1994, Moore and coworkers isolated a series of novel indole alkaloids 1-7 from the extracts of blue-green cyanobacteria Hapalosiphon welwitschii and Westiena intracta.1 Five years later, oxidized welwitindolinones 8-11 were isolated from the epilithic algae Fischerella muscicola and F. major.2 Inter alia, N-methylwelwitindolinone C isothiocyanate (7), which was later dubbed welwistatin, possesses the most relevant biological activity of the entire family (Figure 1).
The Moore group showed that welwistatin 7 attenuates the resistance of MCF-7/ADR cells to vinblastine and actinomycin D at doses as low as 1 μM.3 Additionally, a 36 kb welwitindolinone biosynthetic gene cluster in H. welwitschii was recently identified.4 Except for welwitindolinone A isonitrile (1), all welwitindolinones possess a 3,4-disubstituted oxindole with a bicyclo[4.3.1]decane ring system, numerous stereocenters, and many heteroatoms. Since their initial discovery, numerous approaches toward welwitindolinone alkaloids have been published.5
We have been interested in several chemical reactivities of bridged ketone 12 reported by the Simpkins group.6 They demonstrated that each bridgehead hydrogen in ketone 12, which bears the welwistatin skeleton, can be removed with a strong base and the corresponding anion can react with various electrophiles (Scheme 1).
We considered that bridged ketone 15 might have a similar reactivity as 12 and could be transformed into welwistatin 7 through functional group manipulation. Accordingly, 15 was adopted as our immediate target molecule. Herein we report an efficient synthesis of 15 employing a tandem aldol−Michael reaction.
Scheme 2 shows the retrosynthesis of bridged ketone 15. It was anticipated that bridged ketone 15 could be derived from intermediate 16 through an intramolecular Michael reaction at C-3 of the indole ring. Intermediate 17, which is a potential precursor of intermediate 16, could be fashioned from silyl enol ether 18 and acetone through a Mukaiyama cross aldol reaction. Finally, intermediate 18 could be formed by trapping the corresponding kinetic enolate, generated from ketone 19, with chlorotrimethylsilane.
Our approach began with the synthesis of indolylcyclohexanone 19 (Scheme 3). Using Rawal’s protocol,7 palladium-catalyzed coupling of (cyclohex-1-en-1-yloxy)trimethylsilane with 4-bromoindole 208 provided ketone 199 in 82% yield. After conversion of 19 to silyl enol ether 18 under kinetically controlled reaction conditions, a crucial intermolecular cross aldol reaction was performed.10 Although expected aldol product 17 was not isolated, cyclization product 15 was obtained in 29% yield along with ketone 19 (66%). The spectroscopic properties of synthetic 15 are identical to the previous report.11 It is unclear at present whether the cyclization reaction proceeded via a Michael reaction12 or an attack on the generated tertiary carbocation at the β-position of the carbonyl group in 17.
To accelerate the aldol reaction, different parameters (e.g., substrate concentrations, Lewis acids, and additives) were carefully screened.13 When the aldol reaction was conducted in the presence of 3.0 equivalent of Et3N, expected aldol product 17 was produced in 74% yield as a 4:1 mixture of diastereoisomers along with dehydrated product 21 (10%). The exact role of Et3N is unclear. The relative stereochemistry of the major aldol product 17-cis was determined by a NOE experiment (Scheme 4).
Based on the above results, we planned a tandem aldol−Michael reaction using silyl enol ether 18. To our delight, when the crude aldol product, obtained from 18 with acetone, was subjected to an acidic treatment with pyridinium p-toluenesulfonate, desired product 15 was isolated in 90% yield (Scheme 5). It is worth noting that the reaction described above provided 15 in excellent reproducibility in a high yield without depending upon a reaction scale.
In conclusion, we synthesized bridged ketone 15, which is the carbocyclic core of the welwitindolinone family, by a tandem aldol−Michael reaction as the key step in an overall yield of 66% starting from 4-bromoindole 20. Because the previous preparation of bridged ketone 15 requires more steps and has an overall yield of less than 10%,11 the present methodology should provide a useful knowledge regarding welwitindolinone synthesis.
EXPERIMENTAL
Unless otherwise noted, all reactions were performed in an oven-dried glassware, sealed with rubber septum under an atmosphere of argon. Anhydrous CH2Cl2 was purchased from Kanto Chemical Co., Inc. Et3N and TMSCl were distilled from CaH2 prior to use. Acetone was distilled from KMnO4. Toluene was distilled from P2O5. TiCl4 was distilled prior to use. Unless otherwise mentioned, materials were obtained from commercial suppliers and used without further purification. Flash column chromatography was carried out using Cica 60 N (spherical / 40-50 μm) silica gel. Compounds were visualized using an ultraviolet lamp (254 nm) and / or by staining with p-anisaldehyde (in EtOH) or ammonium molybdate (in 10% H2SO4). IR spectra were measured on a SHIMADZU FT-IR 8300 spectrophotometer. 1H NMR spectra were recorded on Varian 400 MR (400 MHz) spectrometer with CHCl3 and C6H6 as an internal standard. 13C NMR spectra were recorded on Varian 400 MR (100 MHz) spectrometer with CDCl3 as an internal standard. Mass spectra were recorded on Jeol JMS-AX 700 spectrometer.
2-(1-Methyl-1H-indol-4-yl)cyclohexan-1-one (19).9 To a mixture of (cyclohex-1-en-1-yloxy)trimethylsilane (1.62 g, 9.51 mmol), 20 (998 mg, 4.75 mmol), tris(dibenzylidenacetone)dipalladium(0) (109 mg, 0.119 mmol), and Bu3SnF (2.94 g, 9.51 mmol) was added a solution of t-Bu3P (69.0 μL, 0.284 mmol) in toluene (20 mL) at rt. The resulting mixture was heated under reflux for 24 h. After cooling to rt, the mixture was diluted with EtOAc, washed twice with 1N aqueous NaOH solution, brine, dried over Na2SO4, and concentrated to afford a crude product. The crude product was purified by flash column chromatography on silica gel with hexane-EtOAc (4:1 v/v) to produce 19 (885 mg, 82%) as a pale yellow oil. 1H NMR (400 MHz, CDCl3) δ 7.27 (1H, d, J = 6.8 Hz), 7.23 (1H, dd, J = 6.8 and 6.4 Hz), 7.05 (1H, d, J = 3.2 Hz), 6.97 (1H, dd, J = 6.4 and 1.4 Hz), 6.33 (1H, d, J = 3.2 Hz), 4.00 (1H, dd, J = 6.4 and 5.6 Hz), 3.79 (1H, s), 2.64−2.51 (2H, m), 2.42−2.28 (2H, m), 2.26−2.15 (1H, m), 2.13−2.04 (1H, m) and 1.97−1.84 (2H, m)
1-Methyl-4-(2-((trimethylsilyl)oxy)cyclohex-2-en-1-yl)-1H-indole (18). To a solution of diisopropylamine (0.270 mL, 1.93 mmol) in THF (5.0 mL) was added dropwise BuLi (1.10 mL 1.63 M in hexane 1.79 mmol) at –78 °C. After 15 min, ketone 19 (206 mg, 0.906 mmol) in THF (3.0 mL) was added at –78 °C. After stirring for 30 min, TMSCl (0.350 mmol, 2.76 mmol) was added at –78 °C. The mixture was stirred at the same temperature for 15 min, and allowed to warm to rt over a period of 1 h. To the mixture was added a saturated aqueous NaHCO3 solution at 0 °C, and the resulting mixture was extracted three times with hexane−EtOAc (1:1 v/v). The combined organic layers were washed with brine, dried over K2CO3 and evaporated to afford a crude product. The crude product was purified by flash column chromatography on silica gel with hexane−EtOAc (20:1 v/v) to lead to 18 (240 mg, 89%) as a pale yellow oil. IR (neat) 1662 cm–1; 1H NMR (400 MHz, C6D6) δ 7.33−7.26 (2H, m), 6.96 (1H, ddd, J = 7.6, 1.2 and 0.8 Hz), 6.68 (1H, dd, J = 2.8 and 0.8 Hz), 6.57 (1H, d, J = 3.2 Hz), 5.26 (1H, ddd, J = 4.0, 1.2 and 0.8 Hz), 4.09 (1H, dd, J = 5.6 and 5.2 Hz), 2.93 (3H, s), 2.24−2.04 (4H, m), 1.73−1.63 (1H, m), 1.47−1.39 (1H, m) and 0.07 (9H, s); 13C NMR (100 MHz, C6D6) δ 151.8, 137.5, 136.6, 128.2, 127.9, 121.6, 119.3, 107.7, 105.5, 99.7, 44.2, 32.1, 31.6, 24.7, 20.0 and 0.49; LRMS m/z 299 (M)+; HRMS calcd for C18H25ONSi (M)+ 299.1705, found 299.1704.
(6S*,10R*)-2,11,11-Trimethyl-6,7,8,9,10,11-hexahydro-2H-6,10-methanocyclonona[cd]indol-12-one (15).11 To a mixture of 18 (240 mg, 0.801 mmol) and acetone (65.0 μL, 0.882 mmol) in CH2Cl2 (8.0 mL) was added titanium tetrachloride (97.0 μL, 0.885 mmol) at –78 °C. After stirring for 15 min, the mixture was allowed to warm to 0 °C. To the mixture was added a saturated aqueous NaHCO3 solution at 0 °C. The resulting mixture was extracted three times with EtOAc. The combined organic layers were washed with brine, dried over Na2SO4 and evaporated to yield a crude product. The crude product was purified by flash column chromatography on silica gel with hexane−EtOAc (3:1 v/v) to give rise to 15 (62.1 mg, 29%) as a dark red oil and 19 (120 mg, 66%) as a pale yellow oil. 1H NMR (400 MHz, CDCl3) δ 7.23−7.15 (2H, m), 6.94 (1H, s), 6.85−6.83 (1H, m), 4.06 (1H, dd, J = 2.8 and 2.4 Hz), 3.75 (3H, s), 2.59 (1H, d, J = 7.2 Hz), 2.31−2.18 (2H, m), 1.97−1.83 (2H, m), 1.55 (3H, s), 1.46−1.34 (1H, m), 1.26−1.18 (1H, m) and 1.14 (3H, s).
(2S*,6R*)-2-(2-Hydroxypropan-2-yl)-6-(1-methyl-1H-indol-4-yl)cyclohexan-1-one (17-cis). To a mixture of 18 (198 mg, 0.661 mmol) and acetone (54.0 μL, 0.733 mmol) in CH2Cl2 (6.6 mL) was added titanium tetrachloride (81.0 μL, 0.739 mmol) at –78 °C. After stirring for 15 min, to the mixture was adeed Et3N (0.280 mL, 2.01 mmol) at –78 °C and stirred for 1 h. The mixture was allowed to warm to 0 °C. To the mixture was added a saturated aqueous NaHCO3 solution at 0 °C. The resulting mixture was extracted three times with EtOAc. The combined organic layers were washed with brine, dried over Na2SO4 and evaporated to afford a crude product. The crude product was purified by flash column chromatography on silica gel with hexane−EtOAc (2:1 v/v) to furnish 12 (1.2 mg, trace) as a dark red oil, 17-cis (112 mg, 59%) as a colorless oil, 17-trans (28.2 mg, 15%) as a colorless oil and 21 (17.3 mg, 10%) as a brown oil. Data for 17-cis: IR (neat) 3510 and 1698 cm–1; 1H NMR (400 MHz, CDCl3) δ 7.28−7.21 (2H, m), 7.05 (1H, d, J = 3.2 Hz), 6.93 (1H, dd, J = 7.2 and 0.8 Hz), 6.26 (1H, dd, J = 3.2 and 0.8 Hz), 4.01 (1H, dd, J = 13.2 and 5.6 Hz), 3.79 (3H, s), 2.71 (1H, ddd, J = 13.2, 5.6 and 1.2 Hz), 2.45−2.25 (3H, m), 2.18−2.10 (1H, m), 2.02−1.90 (1H, m), 1.85−1.74 (1H, m) and 1.28 (6H, s); 13C NMR (100 MHz, CDCl3) δ 214.0, 136.7, 130.5, 128.8, 127.8, 121.7, 118.5, 108.6, 99.2, 71.6, 60.4, 56.4, 34.9, 33.1, 30.9, 28.8, 25.9 and 25.9; LRMS m/z 285 (M)+; HRMS calcd for C18H23O2N (M)+ 285.1729, found 285.1732. Data for 17-trans: IR (neat) 3502 and 1694 cm–1; 1H NMR (400 MHz, CDCl3) δ 7.28−7.22 (2H, m), 7.14 (1H, d, J = 6.8 Hz), 7.04 (1H, d, J = 3.2 Hz), 6.49 (1H, d, J = 3.2 Hz), 4.14 (1H, dd, J = 5.2 and 4.4 Hz), 3.79 (3H, s), 2.73−2.66 (1H, m), 2.51 (1H, dd, J = 12.4 and 5.2 Hz), 2.26−2.11 (3H, m), 1.96−1.76 (2H, m) and 1.12 (6H, s).; 13C NMR (100 MHz, CDCl3) δ 218.1, 136.9, 130.1, 128.9, 128.0, 121.7, 117.0, 108.6, 100.2, 71.4, 56.3, 55.0, 33.1, 30.1, 29.5, 28.9, 26.3 and 21.9; LRMS m/z 285 (M)+; HRMS calcd for C18H23O2N (M)+ 285.1729, found 285.1730. Data for 21: IR (neat) 1714 cm–1; 1H NMR (400 MHz, CDCl3) δ 6.35 (0.33H, d, J = 2.8 Hz) and 6.28 (1H, d, J = 2.8 Hz); LRMS m/z 267 (M)+; HRMS calcd for C18H21ON (M)+ 267.1623, found 267.1623.
Ketone 15 (Tandem aldol−Michael reaction). To a mixture of 18 (50.9 mg, 0.170 mmol) and acetone (14.0 μL, 0.190 mmol) in CH2Cl2 (1.7 mL) was added titanium tetrachloride (21.0 μL, 0.192 mmol) at –78 °C. After 15 min, Et3N (72.0 μL, 0.517 mmol) was added at –78 °C and the stirring of the resulting reaction mixture was continued for 1 h at –78 °C. The mixture was allowed to warm to 0 °C over a period of 30 min. To the mixture were added water and a saturated aqueous NaHCO3 solution at 0 °C. The resulting mixture was extracted three times with EtOAc. The combined organic layers were washed with brine, dried over Na2SO4 and evaporated to afford a crude product. The crude product was added to a benzene solution (5.7 mL) of PPTS (4.3 mg, 17.0 μmol), and the mixture was heated under reflux for 18 h. After cooling to rt, to the reaction mixture was added a saturated aqueous NaHCO3 solution. The resulting mixture was extracted three times with EtOAc. The combined organic layers were washed with brine, dried over Na2SO4 and evaporated to afford a crude product. The crude product was purified by flash column chromatography on silica gel with hexane−EtOAc (3:1 v/v) to provide 15 (40.8 mg, 90%) as a dark red oil.
References
1. K. Startmann, R. E. Moore, R. Bonjouklian, J. B. Deeter, G. M. L. Patterson, S. Shaffer, C. D. Smith, and T. A. Smikta, J. Am. Chem. Soc., 1994, 116, 9935. CrossRef
2. J. I. Jimenez, U. Huber, R. E. Moore, and G. M. L. Patterson, J. Nat. Prod., 1999, 62, 569. CrossRef
3. C. D. Smith, J. T. Zilfou, K. Startmann, G. M. L. Patterson, and R. E. Moore, Mol. Pharmacol., 1995, 47, 241.
4. M. L. Hillwig, H. A. Fuhrman, K. Ittaiamornkul, T. J. Sevco, D. H. Kwak, and X. Liu, ChemBioChem, 2014, 15, 665. CrossRef
5. For a review: A. D. Huters, E. D. Styduhar, and N. K. Garg, Angew. Chem. Int. Ed., 2012, 51, 3758; CrossRef A short review: J. L. Wood, Nature Chem., 2012, 4, 341; CrossRef Total Syntheses of (+)-welwitindolinone A isonitrile: J. M. Richter, Y. Ishihara, T. Matsuda, B. W. Whitefield, T. Llamas, A. Pohjakallio, and P. S. Baran, J. Am. Chem. Soc., 2008, 130, 17938; CrossRef P. S. Baran, T. J. Maimone, and J. M. Richter, Nature, 2007, 446, 404; CrossRef P. S. Baran and J. M. Richter, J. Am. Chem. Soc., 2005, 127, 15394; CrossRef Total Syntheses of (±)-welwitindolinone A isonitrile: S. E. Reisman, J. M. Ready, A. Hasuoka, C. J. Smith, and J. L. Wood, J. Am. Chem. Soc., 2006, 128, 1448; CrossRef Total Syntheseis of (±)-N-methylwelwitindolinone D isonitrile: V. Bhat, K. M. Allan, and V. H. Rawal, J. Am. Chem. Soc., 2011, 133, 5798; CrossRef Total Synthesis of ( ̶ )-N-methylwelwitindolinone C isothiocyanate: A. D. Huters, K. W. Quasdorf, E. D. Styduhar, and N. K. Garg, J. Am. Chem. Soc., 2011, 133, 15797; CrossRef K. M. Allan, K. Kobayashi, and V. H. Rawal, J. Am. Chem. Soc., 2012, 134, 1392; CrossRef Total syntheses of ( ̶ )-N-methylwelwitindolinone C isonitrile and ( ̶ )-3-hydroxy-N-methylwelwitindolinone C isothiocyanate: K. M. Allan, K. Kobayashi, and V. H. Rawal, J. Am. Chem. Soc., 2012, 134, 1392; CrossRef Formal synthesis of (±)-N-methylwelwitindolinone C isothiocyanate: T.-H. Fu, W. T. McElroy, M. Shamszad, R. W. Heidebrecht, Jr., B. Gulledge, and S. F. Martin, Tetrahedron, 2013, 69, 5588; CrossRef R. W. Heidebrecht, Jr., B. Gulledge, and S. F. Martin, Org. Lett., 2010, 12, 2492. CrossRef
6. V. Boissel, N. S. Simpkins, G. Bhalay, A. J. Blake, and W. Lewis, Chem. Commun., 2009, 1398. CrossRef
7. T. Iwata and V. H. Rawal, Org. Lett., 2006, 8, 5725. CrossRef
8. R. J. Rafferty and R. M. Williams, Tetrahedron Lett., 2011, 52, 2037. CrossRef
9. J. Baudoux, A. J. Blake, and N. S. Simpkins, Org. Lett., 2005, 7, 4087. CrossRef
10. T. Mukaiyama, K. Narasaka, and K. Banno, Chem. Lett., 1973, 1011; CrossRef T. Mukaiyama, K. Banno, and K. Narasaka, J. Am. Chem. Soc., 1974, 96, 7503; CrossRef Review, inter alia: T. Mukaiyama, Org. React., 1982, 28, 203.
11. B. R. Buckley and B. F. Diaz-Ropero, Tetrahedron Lett., 2013, 54, 843; CrossRef J. Szmuszkovicz, J. Am. Chem. Soc., 1957, 79, 2819. CrossRef
12. G. B. Jones and B. J. Chapman, ‘Comprehensive Heterocyclic Chemistry II,’ Vol. 2, ed. by C. W. Bird, Pergamon, 1996, pp. 169-170.
13. Unfortunately, attempts to effect the direct cross aldol reaction of ketone 19, using a variety of established procedures, met with failure. See, inter alia: R. Nagase, N. Matsumoto, K. Hosomi, T. Higashi, S. Funakoshi, T. Misaki, and Y. Tanabe, Org. Biomol. Chem., 2007, 5, 151; CrossRef N. Kagawa, M. Toyota, and M. Ihara, Aust. J. Chem., 2004, 57, 655; CrossRef D. A. Evans, J. S. Clark, R. Metternich, V. J. Novack, and G. S. Sheppard, J. Am. Chem. Soc., 1990, 112, 866. CrossRef