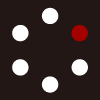
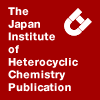
HETEROCYCLES
An International Journal for Reviews and Communications in Heterocyclic ChemistryWeb Edition ISSN: 1881-0942
Published online by The Japan Institute of Heterocyclic Chemistry
e-Journal
Full Text HTML
Received, 16th April, 2014, Accepted, 29th May, 2014, Published online, 3rd June, 2014.
DOI: 10.3987/COM-14-S(K)23
■ N-(2,3,4,5,6-Pentafluorophenyl)maleimide as a Powerful Dienophile in Dearomatizing Diels-Alder Reactions
Koichi Hagiwara, Masafumi Iwatsu, Daisuke Urabe, and Masayuki Inoue*
Graduate School of Pharmaceutical Sciences, The University of Tokyo, 7-3-1 Hongo, Bunkyo-ku, Tokyo 113-0033, Japan
Abstract
Here we demonstrate that N-(2,3,4,5,6-pentafluorophenyl)maleimide effectively promotes dearomatizing Diels-Alder reactions of 2,5-dimethylbenzene-1,4-diol and naphthalen-2-ol derivatives. The present reactions successfully converted the sp2-rich components to the desired products with multiple sp3-carbons in a single step. The adduct was further derivatized into the intermediate for our synthesis of 9-demethyl-10,15-dideoxyryanodol, an analogue of a sp3-rich natural product, in two steps.Pharmaceutically important natural products often contain a high proportion of sp3-hybridized carbon centers as exemplified by terpenoids, steroids, sugars, and macrolides. The three-dimensionality of these compounds that sp3-centers impart generally results in greater selectivity toward target proteins. Architectural complexity and the presence of stereogenic centers have also attracted the attention of medicinal chemists, since sp3-rich synthetic compounds have been correlated with higher success rates in various stages of the drug discovery process in comparison to the more traditional sp2-rich aromatic and heteroaromatic compounds.1 Therefore, development of efficient assembly methodologies for sp3-rich structures would contribute to both natural product synthesis and medicinal chemistry.
The Diels-Alder reaction using aromatic compounds as dienes is a powerful method for installing multiple sp3-carbons in a single step.2 Although dearomatization is an energetically disfavored process, phenol3 and naphthalenol derivatives4 have been shown to function as dienes (Scheme 1).5 Remarkably, upon heating to approximately 210 °C without solvent, maleic anhydride 1 and 2,5-dimethylbenzene-1,4-diol 2 were converted to the fused tricycle 4 with simultaneous introduction of two quaternary centers in 24% yield.6 Similarly, tetracyclic compound 8 was obtained from a reagent combination of 1 and naphthalene-2,7-diol 6c (12% yield) along with its stereoisomer (17% yield). In these reactions, formation of the more stable keto form 4/8 from the enol form 3/7 would be the decisive factor for preventing the retro-Diels-Alder reaction from 3/7 to aromatic 2/6c. Importantly, the four stereogenic sp3-centers were simultaneously introduced to 4 and 8 starting from flat molecules, and the resulting sp3-rich structures of 4 and 8 acted as useful intermediates for further transformations. We recently employed compound 5, which was prepared from 4 in one step, for synthesis of the densely functionalized natural product analogue, 9-demethyl-10,15-dideoxyryanodol,6 while Jackson et al. reported rapid access to a TNF-α signaling inhibitor (9) through a three-step sequence from 8.7
Despite the high versatility of the adducts, dearomatizing Diels-Alder reactions have rarely been used in organic synthesis due to their low yielding outcomes. We hypothesized that lowering the LUMO energy of the dienophile would enhance the LUMO/HOMO orbital interaction between the dienophile and the electron-rich aromatic ring, thereby improving the yield of the product. Here we report the highly efficient dearomatizing Diels-Alder reactions of 2 and various naphthalen-2-ol derivatives 6 by application of N-(2,3,4,5,6-pentafluorophenyl)maleimide 10c.
N-(Phenyl)maleimide derivatives were chosen as the alternative dienophiles to maleic anhydride 1 for the following two reasons.8 First, the LUMO energy would be effectively decreased by simply introducing the electron-withdrawing fluorides on the phenyl group.9 Second, the fact that the imide was chemically more stable than the corresponding acid anhydride was expected to favorably affect the product yield under the high-temperature reaction conditions. We thus investigated the dearomatizing Diels-Alder reaction of 2,5-dimethylbenzene-1,4-diol 2 to access the reactivity of N-(phenyl)maleimide and its fluorinated derivatives (Table 1). All the reactions were performed at high concentration (3.0 M) in 1,2-dichlorobenzene at 200 ºC under microwave irradiation for 11 h.10 The Diels-Alder reaction of N-(phenyl)maleimide 10a gave the adduct 11a in a slightly higher yield (23% yield, entry 2) than that of maleic anhydride 1 (16% yield, entry 1). As clearly shown in entries 3 and 4, the yields of the adducts were improved by attaching fluorides to the phenyl ring. Specifically, N-(2,4,6-trifluorophenyl)maleimide 10b (entry 3)11 and N-(2,3,4,5,6-pentafluorophenyl)maleimide 10c (entry 4)11,12 were transformed to the corresponding adducts 11b and 11c in 37% and 40% yields, respectively. Hence, a significantly higher yield was attained merely by switching the oxygen of 1 to the pentafluorophenyl nitrogen of 10c. These results together corroborated the importance of the low LUMO energy of the dienophile for efficient dearomatizing Diels-Alder reactions.
The Diels-Alder reactions of the six naphthalen-2-ol derivatives 6a-f were then explored using the optimal dienophile 10c (Table 2). As expected, the combined yields of the adduct 12 and its stereoisomer 13 from 10c were found to be uniformly higher than those of the corresponding adducts from maleic anhydride 1.4 Upon heating naphthalen-2-ol 6a and 10c to 200 °C in 1,2-dichlorobenzene for 3 h, the tetracyclic compound 12a was obtained along with the minor isomer 13a in 70% combined yield (entry 1). The cycloaddition of 10c reliably afforded the Diels-Alder products, even when the naphthalene ring had a second hydroxy group (entries 2-5). Naphthalene-1,7-diol 6b (entry 2), naphthalene-2,7-diol 6c (entry 3) and naphthalene-2,6-diol 6d (entry 4) were converted to the corresponding tetracyclic compounds 12b-d as the major products and 13b-d as the minor ones in 57%, 53% and 75% yields, respectively. The yield of the adduct toward 12 over 13 were somewhat lower when naphthalene-1,6-diol 6e was applied, yet the product 12e were formed in 36% yield as a single isomer (entry 5). As in entry 6, the additional bromide on the naphthalene ring was indifferent to the outcome of the Diels-Alder reaction, and the major adduct 12f with the bromobenzene moiety was generated from 6f along with the minor adduct 13f. The stereostructures of 12a-f were confirmed from the long range 1HA-1HB couplings.
All the Diels-Alder reactions in Table 2 selectively produced the 12 series over the 13 series. The stereoselective outcomes were rationalized by considering the two possible transition states, TS-1 and TS-2 (Scheme 2). Namely, the pentafluorophenyl group of 10c induces a more unfavorable steric interaction in TS-2 as compared to TS-1, resulting in selective formation of 12 via TS-1.
To demonstrate the utility of the adduct, 11c was converted to the C2-symmetric bicyclo[2.2.2]octene 5 (Scheme 3), which was the intermediate for the synthesis of 9-demethyl-10,15-dideoxyryanodol (see Scheme 1). Acidic hydrolysis of 11c with aqueous sulfuric acid provided trans-14 due to the acid-induced epimerization.13 Thus obtained 14 was converted into 5 by the Pb(OAc)4-promoted oxidative decarboxylation.
In summary, N-(2,3,4,5,6-pentafluorophenyl)maleimide 10c acted as a powerful dienophile in the dearomatizing Diels-Alder reactions of 2,5-dimethylbenzene-1,4-diol 2 and naphthalen-2-ol derivatives 6. The present operationally simple transformation enables the construction of tricycles 11a-c with two quaternary carbons and various tetracycles 12a-f, 13a-d, and 13f in a single step. The functionalized carbocyclic adducts obtained here would serve as versatile intermediates for quick access to structurally complex sp3-rich natural products and pharmaceuticals.
EXPERIMENTAL
All reactions sensitive to air or moisture were carried out under argon atmosphere in dry solvents under anhydrous conditions, unless otherwise noted. All reagents were used as supplied. The microwave irradiation experiments were performed using standard Pyrex vessels (capacity 0.5 mL or 2.0 mL) on Biotage Initiator. The temperature profiles of the solvents (power control) were monitored using calibrated infrared temperature control mounted underneath the reaction vessel. Analytical thin-layer chromatography (TLC) was performed using E. Merck Silica gel 60 F254 pre-coated plates. Flash column chromatography was performed using 50-60 μm Silica Gel 60 (Kanto Chemical Co., Inc.). Melting points were measured on a Yanaco MP-S3 micro melting point apparatus, and are uncorrected. Infrared (IR) spectra were recorded on JASCO FT/IR-4100 and Perkin Elmer Spectrum BX FT-IR spectrometers. 1H and 13C NMR spectra were recorded on JEOL JNM-ECX-500, JNM-ECA-500, or JNM-ECS-400 spectrometers. Chemical shifts were reported in ppm on the δ scale relative to CHCl3 (δ = 7.26 for 1H NMR), CDCl3 (δ = 77.0 for 13C NMR), (CD2H)CD3SO (δ = 2.50 for 1H NMR), (CD3)2SO (δ = 39.52 for 13C NMR), (CD2H)(CD3)CO (δ = 2.05 for 1H NMR), and (CD3)2CO (δ = 206.26 for 13C NMR) as internal references. Signal patterns are indicated as s, singlet; d, doublet; t, triplet; m, multiplet; br, broaden peak. High resolution mass spectra were measured on Bruker microTOFII and APEX III instruments.
N-(2,3,4,5,6-Pentafluorophenyl)maleimide 10c. A solution of 2,3,4,5,6-pentafluoroaniline (4.1 g, 22 mmol) in 1,2-dichloroethane (30 mL) was added dropwise to a solution of maleic anhydride (1.6 g, 16 mmol) in 1,2-dichloroethane (30 mL) at room temperature. The reaction mixture was stirred at 70 ˚C for 1 h, cooled to room temperature, and was concentrated. Then a mixture of the residue and NaOAc (707 mg, 8.62 mmol) in Ac2O (15 mL) was heated to 100 ºC. After being stirred for 1 h, the reaction mixture was cooled to room temperature. Ac2O was azeotropically removed with toluene in vacuo. The resultant residue was purified by crystallization from EtOAc/cyclohexane to afford N-(2,3,4,5,6-pentafluorophenyl)maleimide 10c (2.1 g, 8.1 mmol) in 51% yield over 2 steps: white solid; mp 107-108 ºC; IR (film) 3499, 3090, 1733, 1526, 1516, 1361, 1301, 1168, 1146, 1074 cm-1; 1H NMR (400 MHz, CDCl3) δ 6.98 (2H, s, COCH=CHCO); 13C NMR (100 MHz, CDCl3) δ 106.2 (m), 135.3, 137.9 (m), 142.1 (m), 144.1 (m), 166.8; HRMS (ESI) calcd for C11H6F5NO3Na [M+MeOH+Na]+ 318.0160, found 318.0173.
Cycloadduct 4. A vessel charged with 1 (903 mg, 9.21 mmol), 2 (415 mg, 3.01 mmol) and 1,2-dichlorobenzene (1.0 mL) was degassed by freeze-thaw procedure (x3), sealed, and irradiated with microwaves at normal absorption level at 100 °C for 30 min, 150 °C for 30 min, and then 200 °C for 11 h. The reaction mixture was cooled to room temperature, and was diluted with acetone. After the mixture was concentrated, remaining 1,2-dichlorobenzene was azeotropically removed with toluene (x3). Et2O (40 mL) was added to the residue, and the mixture was stirred under ultrasonic irradiation for 30 min. The generated slurry was filtered, and the cake was washed with Et2O. The brown solid was dissolved in acetone (30 mL), and then activated charcoal (powder, 1 g) was added. The suspension was vigorously stirred at room temperature for 12 h. Filtration and concentration of the solution afforded yellow solid, which was washed with a 1:1 mixture of hexane and Et2O to afford 4 (113 mg, 0.477 mmol) in 16% yield: white solid; mp 235 ºC; IR (film) 2946, 1777, 1724, 1448, 1388, 1255, 1215, 1122, 1078, 942 cm-1; 1H NMR (500 MHz, DMSO-d6) δ 1.189 (3H, s, CH3), 1.195 (3H, s, CH3), 2.37 (1H, d, J = 20.1 Hz, CHaHb), 2.53 (1H, d, J = 19.5 Hz, CHaHb), 2.63 (1H, dd, J = 20.1, 2.3 Hz, CHaHb), 2.76 (1H, d, J = 19.5 Hz, CHaHb), 3.42 (1H, dd, J = 9.8, 2.3 Hz, CHCH), 3.73 (1H, d, J = 9.8 Hz, CHCH); 13C NMR (100 MHz, CDCl3) δ 15.8, 16.0, 42.8, 44.3, 45.7, 47.2, 47.8, 48.4, 170.2, 170.3, 207.1, 207.5; HRMS (EI) calcd for C12H12O5 [M]+ 236.0679, found 236.0683.
General procedure A for the Diels-Alder reaction: Cycloadduct 11a. A vessel charged with 2 (418 mg, 3.03 mmol), 10a (1.6 g, 9.0 mmol), and 1,2-dichlorobenzene (1.0 mL) was degassed by freeze-thaw procedure (x3), sealed, and irradiated with microwaves at normal absorption level at 100 °C for 30 min, 150 °C for 30 min, and then 200 °C for 11 h. The reaction mixture was cooled to room temperature, and was diluted with acetone. After the mixture was concentrated, remaining 1,2-dichlorobenzene was azeotropically removed with toluene (x3). The residue was purified by flash column chromatography on silica gel (50 g, hexane/EtOAc 3:1 to 1:1 to 1:3) and recrystallization from hexane/EtOAc to afford 11a (135 mg, 0.434 mmol). The mother liquid was re-purified by flash column chromatography on silica gel (10 g, hexane/EtOAc 3:1 to 1:1 to 1:3) and recrystallization from hexane/EtOAc to afford 11a (78 mg, 0.25 mmol). The total yield of 11a was calculated to be 23%: white solid; mp 198-200 ºC; IR (film) 2935, 1728, 1712, 1496, 1383, 1184 cm-1; 1H NMR (400 MHz, CDCl3) δ 1.47 (3H, s, CH3), 1.49 (3H, s, CH3), 2.46 (1H, dd, J = 20.2, 1.8 Hz, CHaHb), 2.53 (1H, d, J = 19.7 Hz, CHaHb), 2.57 (1H, d, J = 20.2 Hz, CHaHb), 2.64 (1H, d, J = 19.7 Hz, CHaHb), 3.13 (1H, dd, J = 9.6, 1.8 Hz, CHCH), 3.20 (1H, d, J = 9.6 Hz, CHCH), 7.15-7.19 (2H, m, aromatic), 7.39-7.51 (3H, m, aromatic); 13C NMR (100 MHz, CDCl3) δ 16.4, 17.0, 43.2, 44.6, 46.1, 47.4, 48.3, 49.0, 126.4, 129.2, 129.3, 131.0, 173.0, 173.3, 206.9, 207.5; HRMS (ESI) calcd for C18H17NO4Na [M+Na]+ 334.1050, found 334.1051.
Cycloadduct 11b. According to the general procedure A, 11b (313 mg, 0.858 mmol) was synthesized by using 2 (312 mg, 2.26 mmol), 10b (1.5 g, 6.7 mmol), and 1,2-dichlorobenzene (750 µL) in 37% yield. The residue was purified by flash column chromatography on silica gel (25 g, hexane/EtOAc 10:1 to 3:1) and recrystallization from hexane/EtOAc: white solid; mp 229-231 ºC; IR (film) 3085, 2937, 1728, 1611, 1519, 1454, 1393, 1351, 1178, 1125 cm-1; 1H NMR (400 MHz, CDCl3) δ 1.47 (3H, s, CH3), 1.48 (3H, s, CH3), 2.47 (1H, dd, J = 20.6, 1.8 Hz, CHaHb), 2.52 (1H, d, J = 19.7 Hz, CHaHb), 2.62 (1H, d, J = 20.6 Hz, CHaHb), 2.65 (1H, d, J = 19.7 Hz, CHaHb), 3.22 (1H, dd, J = 9.6, 1.8 Hz, CHCH), 3.33 (1H, d, J = 9.6 Hz, CHCH), 6.84 (2H, t, J = 8.2 Hz, aromatic); 13C NMR (100 MHz, (CD3)2CO) δ 16.8, 17.2, 43.6, 46.1, 46.2, 48.7, 48.8, 49.8, 102.3 (dddd, J = 27.8, 24.0, 9.6, 3.8 Hz), 106.9 (td, J = 18.2, 5.8 Hz), 159.6 (m), 164.1 (dt, J = 252.9, 15.3 Hz), 173.6, 173.9, 207.2, 207.8; HRMS (ESI) calcd for C18H14F3NO4Na [M+Na]+ 388.0767, found 388.0764.
Cycloadduct 11c. According to the general procedure A, 11c (412 mg, 1.03 mmol) was synthesized by using 2 (355 mg, 2.59 mmol), 10c (2.0 g, 7.7 mmol), and 1,2-dichlorobenzene (860 µL) in 40% yield. The residue was purified by flash column chromatography on silica gel (25 g, hexane/EtOAc 10:1 to 8:1 to 5:1 to 3:1 to 1:1) and recrystallization from hexane/EtOAc. The mother liquid was re-purified by flash column chromatography on silica gel (5 g, hexane/EtOAc 5:1 to 3:1) and recrystallization from hexane/EtOAc: white solid; mp 175-177 ºC; IR (film) 2939, 1732, 1521, 1362, 1304, 1179, 1140, 992 cm-1; 1H NMR (400 MHz, CDCl3) δ 1.47 (3H, s, CH3), 1.48 (3H, s, CH3), 2.49 (1H, dd, J = 19.7, 1.8 Hz, CHaHb), 2.54 (1H, d, J = 20.2 Hz, CHaHb), 2.59 (1H, d, J = 20.2 Hz, CHaHb), 2.66 (1H, d, J = 19.7 Hz, CHaHb), 3.26 (1H, dd, J = 9.6, 1.8 Hz, CHCH), 3.37 (1H, d, J = 9.6 Hz, CHCH); 13C NMR (100 MHz, CDCl3) δ 16.2, 16.9, 42.9, 45.2, 45.9, 47.9, 48.1, 49.1, 106.3 (td, J = 15.3, 3.8 Hz), 138.0 (m), 142.5 (m), 144.2 (m), 170.9, 171.3, 205.6, 206.6; HRMS (ESI) calcd for C18H12F5NO4Na [M+Na]+ 424.0579, found 424.0586.
General procedure B for the Diels-Alder reaction: Cycloadducts 12a and 13a. A vessel charged with 6a (72 mg, 0.50 mmol), 10c (400 mg, 1.52 mmol), and 1,2-dichlorobenzene (390 µL) was sealed, and irradiated with microwaves at normal absorption level at 200 °C for 3 h. The reaction mixture was cooled to room temperature, and was diluted with acetone. After the mixture was concentrated, remaining 1,2-dichlorobenzene was azeotropically removed with toluene (x3). The residue was purified by flash column chromatography on silica gel (5 g, CH2Cl2 only to CH2Cl2/EtOAc 30:1) to afford 12a (130 mg, 0.319 mmol) and 13a (13 mg, 0.032 mmol) in 64% and 6% yields, respectively. 12a: white solid; mp 235-237 °C; IR (film) 3512, 3027, 1798, 1735, 1658, 1522, 1478, 1362, 1299, 1173, 1141, 992 cm-1; 1H NMR (400 MHz, CDCl3) δ 2.38 (1H, ddd, J = 19.7, 2.8, 1.8 Hz, C(O)CHAH), 2.49 (1H, dd, J = 19.7, 2.8 Hz, C(O)CHAH), 3.45 (1H, ddd, J = 9.6, 3.6, 1.8 Hz, CH2CHCHB), 3.51 (1H, dd, J = 9.6, 3.6 Hz, CH2C(O)CHCH), 4.05 (1H, dt, J = 3.6, 2.8 Hz, CH2CH), 4.22 (1H, d, J = 3.6 Hz, CH2C(O)CH), 7.33-7.41 (4H, m, aromatic); 13C NMR (100 MHz, CDCl3) δ 38.0, 38.2, 45.1, 45.5, 53.5, 106.5 (m), 124.7, 126.6, 128.6, 128.9, 133.5, 138.0 (m), 140.1, 142.4 (m), 143.5 (m), 172.5, 173.5, 204.4; HRMS (ESI) calcd for C20H10F5NO3Na [M+Na]+ 430.0473, found 430.0464. 13a: yellow solid; mp 60-62 °C; IR (film) 1732, 1520, 1363, 1299, 1179, 1147, 994 cm-1; 1H NMR (400 MHz, CDCl3) δ 2.43 (1H, dd, J = 19.2, 3.7 Hz, C(O)CHaHb), 2.54 (1H, dd, J = 19.2, 2.8 Hz, CHaHb), 3.61 (1H, dd, J = 9.2, 3.2 Hz, CH2CHCH), 3.70 (1H, dd, J = 9.2, 3.2 Hz, CH2C(O)CHCH), 4.06 (1H, ddd, J = 3.7, 3.2, 2.8 Hz, CH2CH), 4.19 (1H, d, J = 3.2 Hz, CH2C(O)CH), 7.27-7.38 (4H, m, aromatic); 13C NMR (100 MHz, (CD3)2CO) δ 39.6, 40.2, 43.4, 46.2, 54.3, 107.9 (td, J = 15.3, 3.8 Hz), 126.6, 127.8, 129.3, 129.7, 133.1, 138.7 (m), 139.3, 143.0 (m), 143.9 (m), 174.0, 174.9; One 13C NMR signal was overlapped with that of deuterated acetone; HRMS (ESI) calcd for C20H10F5NO3Na [M+Na]+ 430.0473, found 430.0465.
Cycloadducts 12b and 13b. According to the general procedure B, 12b (100 mg, 0.236 mmol) and 13b (21 mg, 0.050 mmol) were synthesized by using 6b (80 mg, 0.50 mmol), 10c (400 mg, 1.52 mmol), and 1,2-dichlorobenzene (390 µL) under the microwave irradiation for 5 h in 47% and 10% yields, respectively. The residue was purified by flash chromatography on silica gel (15 g, CH2Cl2 only to CH2Cl2/EtOAc 10:1). 12b: yellow solid; mp 258-259 °C; IR (film) 3385, 1794, 1727, 1594, 1521, 1472, 1363, 1296, 1175, 1141, 989 cm-1; 1H NMR (400 MHz, CDCl3) δ 2.38 (1H, ddd, J = 19.7, 2.7, 1.8 Hz, C(O)CHAH), 2.47 (1H, dd, J = 19.7, 2.7 Hz, C(O)CHAH), 3.43 (1H, ddd, J = 9.6, 3.6, 1.8 Hz, CH2CHCHB), 3.51 (1H, dd, J = 9.6, 3.6 Hz, CH2C(O)CHCH), 4.01 (1H, dt, J = 3.6, 2.7 Hz, CH2CH), 4.67 (1H, m, CH2C(O)CH), 5.11-5.40 (1H, br s, OH), 6.79 (1H, d, J = 8.2 Hz, aromatic), 6.98 (1H, d, J = 7.8 Hz, aromatic), 7.21 (1H, dd, J = 8.2, 7.8 Hz, aromatic); 13C NMR (100 MHz, (CD3)2CO) δ 38.6, 38.7, 45.9, 46.1, 47.6, 107.9 (td, J = 15.8, 3.8 Hz), 115.8, 117.1, 120.4, 129.9, 138.7 (m), 143.0 (m), 143.5, 144.3 (m), 154.3, 174.1, 174.9, 205.3; HRMS (ESI) calcd for C20H10F5NO4Na [M+Na]+ 446.0422, found 446.0427. 13b: yellow solid; mp 120-122 °C; IR (film) 3441, 2959, 2928, 1796, 1730, 1595, 1520, 1470, 1366, 1295, 1180, 1148, 993 cm-1; 1H NMR (400 MHz, CDCl3) δ 2.43 (1H, dd, J = 18.8, 3.2 Hz, C(O)CHaHb), 2.52 (1H, dd, J = 18.8, 2.3 Hz, C(O)CHaHb), 3.58 (1H, dd, J = 8.7, 3.2 Hz, CH2CHCH), 3.72 (1H, dd, J = 8.7, 3.2 Hz, CH2C(O)CHCH), 4.02 (1H, td, J = 3.2, 2.3 Hz, CH2CH), 4.64 (1H, d, J = 3.2 Hz, CH2C(O)CH), 5.22 (1H, br s, OH), 6.78 (1H, d, J = 8.2 Hz, aromatic), 6.88 (1H, d, J = 7.9 Hz, aromatic), 7.19 (1H, dd, J = 8.2, 7.9 Hz, aromatic); 13C NMR (100 MHz, (CD3)2CO) δ 39.7, 40.1, 43.3, 46.0, 47.3, 108.0 (m), 115.9, 117.8, 118.5, 130.1, 138.7 (m), 140.9, 142.6 (m), 144.0 (m), 154.9, 174.0, 174.9; One 13C NMR signal was overlapped with that of deuterated acetone; HRMS (ESI) calcd for C20H10F5NO4Na [M+Na]+ 446.0422, found 446.0402.
Cycloadducts 12c and 13c. According to the general procedure B, a 3.0:1 mixture of 12c and 13c (67 mg, 0.16 mmol) were synthesized by using 6c (48 mg, 0.30 mmol), 10c (240 mg, 0.912 mmol), and 1,2-dichlorobenzene (230 µL) under the microwave irradiation for 2 h in 53% combined yield. The residue was purified by flash column chromatography on silica gel (10 g, CH2Cl2 only to CH2Cl2/EtOAc 3:1). Analytically pure 12c was obtained by recrystallization from EtOAc/hexane. 12c: white solid; mp 286-288 °C; IR (film) 3409, 1795, 1731, 1521, 1362, 1297, 1173, 1140, 990 cm-1; 1H NMR (400 MHz, CDCl3) δ 2.36 (1H, ddd, J = 20.2, 2.7, 1.8 Hz, C(O)CHAH), 2.47 (1H, dd, J = 20.2, 2.7 Hz, C(O)CHAH), 3.43 (1H, ddd, J = 9.6, 3.2, 1.8 Hz, CH2CHCHB), 3.49 (1H, dd, J = 9.6, 3.6 Hz, CH2C(O)CHCH), 3.99 (1H, dt, J = 3.2, 2.7 Hz, CH2CH), 4.14 (1H, d, J = 3.6 Hz, CH2C(O)CH), 4.90 (1H, br s, OH), 6.81 (1H, dd, J = 8.2, 2.8 Hz, aromatic), 6.84 (1H, d, J = 2.8 Hz, aromatic), 7.24 (1H, d, J = 8.2 Hz, aromatic); 13C NMR (125 MHz, (CD3)2CO) δ 38.0, 39.3, 46.4, 46.6, 54.7, 107.9 (m), 114.3, 115.7, 126.8, 132.7, 136.3, 138.9 (m), 143.1 (m), 144.3 (m), 158.1, 174.1, 174.9, 205.7; HRMS (ESI) calcd for C20H10F5NO4Na [M+Na]+ 446.0422, found 446.0401. Because of the inseparable nature of 12c and 13c by column chromatography and HPLC purification, 1H NMR data of 13c was analyzed by using the mixture. 13c: 1H NMR (400 MHz, CDCl3) δ 2.40 (1H, dd, J = 17.8, 2.8 Hz, C(O)CHaHb), 2.50 (1H, dd, J = 17.8, 2.3 Hz, C(O)CHaHb), 3.57 (1H, ddd, J = 9.2, 3.2 Hz, CH2CHCH), 3.66 (1H, dd, J = 9.2, 3.2 Hz, CH2C(O)CHCH), 3.96 (1H, ddd, J = 3.2, 2.8, 2.3 Hz, CH2CH), 4.08 (1H, d, J = 3.2 Hz, CH2C(O)CH), 6.75 (1H, d, J = 2.3 Hz, aromatic), 6.80 (1H, dd, J = 8.2, 2.3 Hz, aromatic), 7.11 (1H, d, J = 8.2 Hz, aromatic).
Cycloadducts 12d and 13d. According to the general procedure B, 12d (140 mg, 0.331 mmol) and 13d (18 mg, 0.043 mmol) were synthesized by using 6d (80 mg, 0.50 mmol), 10c (400 mg, 1.52 mmol), and 1,2-dichlorobenzene (390 µL) under the microwave irradiation for 2 h in 66% and 9% yields, respectively. The residue was purified by flash column chromatography on silica gel (10 g, CH2Cl2 only to CH2Cl2/EtOAc 20:1). 12d: white solid; mp 228-230 °C; IR (film) 3430, 3027, 2966, 1795, 1732, 1617, 1522, 1362, 1300, 1175, 1144, 991 cm-1; 1H NMR (400 MHz, CDCl3) δ 2.37 (1H, ddd, J = 19.7, 2.8, 1.8 Hz, C(O)HAH), 2.47 (1H, dd, J = 19.7, 2.3 Hz, C(O)HAH), 3.43 (1H, ddd, J = 9.6, 3.2, 1.8 Hz, CH2CHCHB), 3.49 (1H, dd, J = 9.6, 3.2 Hz, CH2C(O)CHCH), 3.96 (1H, ddd, J = 3.2, 2.8, 2.3 Hz, CH2CH), 4.16 (1H, d, J = 3.2 Hz, CH2C(O)CH), 5.18 (1H, br s, OH), 6.78 (1H, dd, J = 8.2, 2.8 Hz, aromatic), 6.90 (1H, d, J = 2.8 Hz, aromatic), 7.20 (1H, d, J = 8.2 Hz, aromatic); 13C NMR (100 MHz, (CD3)2CO) δ 38.8, 38.9, 46.0, 46.8, 53.6, 108.0 (td, J = 15.3, 3.8 Hz), 113.2, 115.4, 125.5, 128.4, 138.9 (m), 143.1 (m), 143.3, 144.3 (m), 158.7, 174.2, 175.0, 205.4; HRMS (DART) calcd for C20H14F5N2O4 [M+NH4]+ 441.0868, found 441.0857. 13d: yellow solid; mp 235-238 °C; IR (film) 3439, 1795, 1729, 1522, 1366, 1299, 1179, 1146, 993 cm-1; 1H NMR (400 MHz, CDCl3) δ 2.42 (1H, dd, J = 18.8, 3.2 Hz, C(O)CHaHb), 2.52 (1H, dd, J = 18.8, 2.3 Hz, C(O)CHaHb), 3.58 (1H, dd, J = 8.7, 3.2 Hz, CH2CHCH), 3.66 (1H, dd, J = 8.7, 3.2 Hz, CH2C(O)CHCH), 3.96 (1H, td, J = 3.2, 2.3 Hz, CH2CH), 4.12 (1H, d, J = 3.2 Hz, CH2C(O)CH), 4.88 (1H, br s, OH), 6.78-6.80 (2H, m, aromatic), 7.12 (1H, d, J = 8.2 Hz, aromatic); 13C NMR (100 MHz, (CD3)2CO) δ 39.7, 40.2, 43.7, 46.1, 53.5, 108.0 (m), 113.8, 115.9, 123.3, 128.9, 138.8 (m), 140.6, 142.9 (m), 143.4 (m), 159.0, 174.3, 174.9; One 13C NMR signal was overlapped with that of deuterated acetone; HRMS (ESI) calcd for C20H10F5NO4Na [M+Na]+ 446.0422, found 446.0419.
Cycloadduct 12e. According to the general procedure B, 12e (76 mg, 0.18 mmol) was synthesized by using 6e (80 mg, 0.50 mmol), 10c (400 mg, 1.52 mmol), and 1,2-dichlorobenzene (390 µL) under the microwave irradiation for 3 h in 36% yield. The residue was purified by flash column chromatography on silica gel (15 g, CH2Cl2 only to CH2Cl2/EtOAc 10:1): orange solid; mp 268-271 °C; IR (film) 3437, 1795, 1731, 1593, 1521, 1468, 1362, 1289, 1172, 1140, 990 cm-1; 1H NMR (400 MHz, CDCl3) δ 2.36 (1H, ddd, J = 19.7, 2.8, 1.8 Hz, C(O)CHAH), 2.47 (1H, dd, J = 19.7, 2.3 Hz, C(O)CHAH), 3.43 (1H, ddd, J = 9.6, 3.6, 1.8 Hz, CH2CHCHB), 3.49 (1H, dd, J = 9.6, 3.7 Hz, CH2C(O)CHCH), 4.20 (1H, d, J = 3.7 Hz, CH2C(O)CH), 4.51 (1H, ddd, J = 3.6, 2.8, 2.3 Hz, CH2CH), 5.03 (1H, br s, OH), 6.81 (1H, d, J = 8.2 Hz, aromatic), 6.93 (1H, d, J = 7.8 Hz, aromatic), 7.19 (1H, dd, J = 8.2, 7.8 Hz, aromatic); 13C NMR (125 MHz, (CD3)2CO) δ 32.0, 38.3, 45.8, 46.4, 54.5, 108.0 (td, J = 13.3, 3.8 Hz), 116.3, 118.4, 127.5, 129.6, 136.8, 138.9 (m), 143.1 (m), 144.3 (m), 152.9, 174.1, 174.8, 205.7; HRMS (ESI) calcd for C20H10F5NO4Na [M+Na]+ 446.0422, found 446.0403.
Cycloadducts 12f and 13f. According to the general procedure B, 12f (130 mg, 0.267 mmol) and 13f (30 mg, 0.062 mmol) were synthesized by using 6f (110 mg, 0.493 mmol), 10c (400 mg, 1.52 mmol), and 1,2-dichlorobenzene (390 µL) under the microwave irradiation for 5 h in 53% and 12% yields, respectively. The residue was purified by flash chromatography on silica gel (15 g, CH2Cl2 only to CH2Cl2/EtOAc 50:1). 12f: white solid; mp 238-241 °C; IR (film) 3511, 3024, 2966, 1797, 1736, 1522, 1473, 1413, 1362, 1298, 1174, 1144, 1066, 991 cm-1; 1H NMR (400 MHz, CDCl3) δ 2.36 (1H, ddd, J = 19.7, 3.2, 1.4 Hz, C(O)CHAH), 2.48 (1H, dd, J = 19.7, 2.3 Hz, C(O)CHAH), 3.44 (1H, ddd, J = 10.0, 3.2, 1.4 Hz, CH2CHCHB), 3.48 (1H, dd, J = 10.0, 3.6 Hz, CH2C(O)CHCH), 4.01 (1H, dt, J = 3.2, 2.3 Hz, CH2CH), 4.20 (1H, d, J = 3.6 Hz, CH2C(O)CH), 7.23 (1H, d, J = 7.8 Hz, aromatic), 7.49 (1H, dd, J = 7.8, 2.3 Hz, aromatic), 7.57 (1H, d, J = 2.3 Hz, aromatic); 13C NMR (100 MHz, CDCl3) δ 37.7, 37.8, 44.7, 45.2, 53.0, 106.4 (m), 122.8, 128.1, 128.2, 131.6, 132.5, 138.0 (m), 142.0, 142.5 (m), 143.3 (m), 172.1, 173.1, 203.2; HRMS (ESI) calcd for C20H9BrF5NO3Na [M+Na]+ 507.9578, found 507.9572. 13f: orange solid; mp 189-191 °C; IR (film) 3025, 2962, 1798, 1733, 1523, 1472, 1414, 1364, 1301, 1180, 1148, 1066, 993 cm-1; 1H NMR (400 MHz, CDCl3) δ 2.40 (1H, dd, J = 19.2, 3.2 Hz, C(O)CHaHb), 2.54 (1H, dd, J = 19.2, 2.7 Hz, C(O)CHaHb), 3.61 (1H, dd, J = 9.2, 3.2 Hz, CH2CHCH), 3.70 (1H, dd, J = 9.2, 3.2 Hz, CH2C(O)CHCH), 4.02 (1H, td, J = 3.2, 2.7 Hz, CH2CH), 4.17 (1H, d, J = 3.2 Hz, CH2C(O)CH), 7.14 (1H, d, J = 7.8 Hz, aromatic), 7.47-7.51 (2H, m, aromatic); 13C NMR (100 MHz, (CD3)2CO) δ 39.3, 39.7, 43.3, 45.9, 53.8, 107.8 (m), 123.1, 129.6, 129.7, 132.2, 132.5, 138.8 (m), 141.7, 143.1 (m), 143.9 (m), 173.9, 174.7, 205.5; HRMS (ESI) calcd for C20H9BrF5NO3Na [M+Na]+ 507.9578, found 507.9596.
Carboxylic acid 14. A suspension of 11c (141 mg, 0.351 mmol) in a 1:1 mixture of concentrated H2SO4 and H2O (6 mL) was heated to 150 ºC for 24 h. After the solution was cooled to room temperature, 3M NaOH (30 mL) was added. The resultant mixture was extracted with EtOAc (40 mL x3), and the combined organic layers were dried over Na2SO4, filtered and concentrated. The residue was purified by flash column flash column chromatography on silica gel (10 g, hexane/EtOAc 5:1 to 2:1, EtOAc/AcOH 100:1, then CH2Cl2/MeOH 10:1) to afford carboxylic acid 14 (67 mg, 0.26 mmol) in 74% yield: brown solid; IR (film) 3413, 2921, 1720, 1597, 1512, 1395 cm-1; 1H NMR (400 MHz, (CD3)2CO) δ 1.14 (6H, s, CH3 x 2), 2.31 (2H, d, J = 18.8 Hz, C(O)CHaHb x 2), 2.67 (2H, d, J = 18.8 Hz, C(O)CHaHb x 2), 3.16 (2H, s, CHCOOH x 2); 13C NMR (100 MHz, (CD3)2CO) δ 17.2, 46.4, 48.3, 51.7, 174.1, 207.4; HRMS (ESI) calcd for C12H13O6Na2 [M-H+Na2]+ 299.0502, found 299.0507.
Ketone 5. A suspension of 14 (258 mg, 1.01 mmol) and Pb(OAc)4 (2.0 g, 4.4 mmol) in a 1:1 mixture of 1,4-dioxane and pyridine (6 mL) was stirred at 60 ºC for 1 h. After the solution was cooled to room temperature, 2 M HNO3 (60 mL) and Et2O (30 mL) were successively added. The resultant solution was stirred at room temperature for 30 min. After the separation, the organic layer was washed with 2 M HNO3 (10 mL), H2O (10 mL), and brine (10 mL), dried over Na2SO4, filtered, and concentrated. The residue was purified by flash column chromatography on silica gel (5 g, petane/Et2O 10:1 to 5:1 to 3:1) to afford ketone 5 including solvents (167 mg, 5/pentane/Et2O 1.0:6.7:3.4, 0.18 mmol for 5). The yield of the highly volatile 5 was calculated to be 18% from the 1H NMR spectrum of the mixture. The 1H NMR of 5 was identical to those reported previously.14
ACKNOWLEDGEMENTS
This research was financially supported by the Funding Program for Next Generation World-Leading Researchers (JSPS) to M.I. and a Grant-in-Aid for Scientific Research (C) (JSPS) to D.U.
References
1. F. Lovering, J. Bikker, and C. Humblet, J. Med. Chem., 2009, 52, 6752; CrossRef F. Lovering, Med. Chem. Commun., 2013, 4, 515. CrossRef
2. S. Cossu, F. Fabris, and O. De Lucchi, Synlett, 1997, 1327; CrossRef J. C. C. Atherton and S. Jones, Tetrahedron, 2003, 59, 9039. CrossRef
3. R. C. Cookson and N. S. Wariyar, J. Chem. Soc., 1957, 327; CrossRef C. A. Grob and A. Weiss, Helv. Chim. Acta, 1960, 43, 1390; CrossRef M. Nakazaki, K. Naemura, and H. Yoshihara, Bull. Chem. Soc. Jpn., 1975, 48, 3278; CrossRef C. W. Jefford, T. W. Wallace, and M. Acar, J. Org. Chem., 1977, 42, 1654. CrossRef
4. K. Takeda, K. Kitahonoki, M. Sugiura, and Y. Takano, Chem. Ber., 1962, 95, 2344; CrossRef K. Takeda, S. Hagishita, M. Sugiura, K. Kitahonoki, I. Ban, S. Miyazaki, and K. Kuriyama, Tetrahedron, 1970, 26, 1435. CrossRef
5. For other representative examples of the Diels-Alder reactions of aromatic compounds, see: M. D. Chordia, P. L. Smith, S. H. Meiere, M. Sabat, and W. D. Harman, J. Am. Chem. Soc., 2001, 123, 10756; CrossRef T. Murase, S. Horiuchi, and M. Fujita, J. Am. Chem. Soc., 2010, 132, 2866; CrossRef T. Murase and M. Fujita, Chem. Rec., 2010, 10, 342. CrossRef
6. K. Hagiwara, M. Himuro, M. Hirama, and M. Inoue, Tetrahedron Lett., 2009, 50, 1035; CrossRef D. Urabe, M. Nagatomo, K. Hagiwara, K. Masuda, and M. Inoue, Chem. Sci., 2013, 4, 1615. CrossRef
7. R. W. Jackson, R. Gelinas, T. A. Baughman, T. Cox, J. J. Howbert, K. A. Kucera, J. A. Latham, F. Ramsdell, D. Singh, and I. S. Darwish, Bioorg. Med. Chem. Lett., 2002, 12, 1093. CrossRef
8. For selected reactions of various N-(phenyl)maleimide derivatives, see; K. Uemae, S. Masuda, and Y. Yamamoto, J. Chem. Soc., Perkin Trans. 1, 2001, 1002; CrossRef X. Li, S. Hu, Z. Xi, L. Zhang, S. Luo, and J.-P. Cheng, J. Org. Chem., 2010, 75, 8697; CrossRef J.-J. Wang, X.-J. Dong, W.-T. Wei, and M. Yan, Tetrahedron: Asymmetry, 2011, 22, 690; CrossRef L. Yasmin, K. A. Stubbs, and C. L. Raston, Tetrahedron Lett., 2014, 55, 2246; CrossRef For a review on asymmetric organocatalytic addition reactions of maleimides, see: P. Chauhan, J. Kaur, and S. S. Chimni, Chem. Asian J., 2013, 8, 328. CrossRef
9. For related reactions of pentafluorophenyl derivatives, see: T. Kan and Y. Ohfune, Tetrahedron Lett., 1995, 36, 943; CrossRef Y. Ohfune, T. Kan, and T. Nakajima, Tetrahedron, 1998, 54, 5207; CrossRef M. Inoue, K. Miyazaki, Y. Ishihara, A. Tatami, Y. Ohnuma, Y. Kawada, K. Komano, S. Yamashita, N. Lee, and M. Hirama, J. Am. Chem. Soc., 2006, 128, 9352. CrossRef
10. A. Loupy, Microwaves in Organic Synthesis; Wiley-VCH: Weinheim, 2003; C. O. Kappe, D. Dallinger, and S. S. Murphree, Practical Microwave Synthesis for Organic Chemists: Strategies, Instruments, and Protocols; Wiley-VCH: Weinheim, 2009.
11. A. Schwarzer and E. Weber, Cryst. Growth Des., 2008, 8, 2862. CrossRef
12. K. Bynum, R. Prip, R. Callahan, and R. Rothchild, J. Fluorine Chem., 1998, 90, 39. CrossRef
13. Hydrolysis of 4 occurred in neutral water at 80 °C (Scheme 1), while the acid was required to hydrolyze 11c (Scheme 3), proving the chemical robustness of the imide structure in comparison to the anhydride structure.
14. M. Iwatsu, D. Urabe, and M. Inoue, Heterocycles, 2010, 82, 491. CrossRef