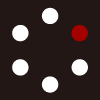
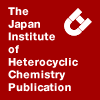
HETEROCYCLES
An International Journal for Reviews and Communications in Heterocyclic ChemistryWeb Edition ISSN: 1881-0942
Published online by The Japan Institute of Heterocyclic Chemistry
e-Journal
Full Text HTML
Received, 28th February, 2014, Accepted, 25th March, 2014, Published online, 26th March, 2014.
DOI: 10.3987/COM-14-S(K)15
■ Palladium-Catalyzed [3+2] Annulation of meso-Bromoporphyrin with Silylacetylenes and Desilylation of 8a-Silyl-7,8-dehydropurpurin
Norihito Fukui, Seiji Arai, Hiroshi Shinokubo, Hideki Yorimitsu,* and Atsuhiro Osuka*
Department of Chemistry, Graduate School of Science, Kyoto University, Sakyo, Kyoto 606-8502, Japan
Abstract
Palladium-catalyzed [3+2] annulation of meso-bromoporphyrins with trimethylsilyl-, tert-butyldimethylsilyl-, and triisopropylsilylacetylenes afforded 8a-silyl-7,8-dehydropurpurins. Protodesilylation of 8a-trimethylsilyl-7,8-de- hydropurpurin resulted in the first synthesis of an 8a,8b-unsubstituted 7,8-de hydropurpurin.INTRODUCTION
The electronic nature of a porphyrin ring is susceptible to modifications through fusion of π-conjugated segments at the periphery. Chemists have hence devoted much time to the design and synthesis of novel π-extended fused porphyrins.1,2 Among them, 7,8-dehydropurpurins are intriguing porphyrinoids that bear a fused five-membered ring that includes a vinylene moiety bridging across the meso and β positions.3–7 They exhibit largely altered absorption reaching out to the near infrared region due to their forbidden HOMO–LUMO transition with a narrow energy gap. These properties arise from the presence of dual 18π- and 20π-electronic circuits that resonate in the conjugated macrocycles.3 Today, a variety of 7,8-dehydropurpurin derivatives have been synthesized, which contain various substituents like aryl,4 alkynyl,5 and porphyrinyl5,6 groups at their 8a- and 8b-positions. In addition, fused π-segments such as phenylene7 and thienylene3 also behave as bridging vinylene moieties. However, 8a,8b-unsubstituted 7,8-dehydropurpurin has been unexplored. Our continuing interest in porphyrin π-systems encouraged us to envisage synthesizing a 7,8-dehydropurpurin having no substituent at the bridging vinylene moiety. Investigation into the 8a,8b-unsubstituted 7,8-dehydropurpurin should be helpful to elucidate the intrinsic nature of its attractive π-system.
Recently, we reported the facile synthesis of 7,8-dehydropurpurin derivatives via Pd-catalyzed [3+2] annulation of meso-bromoporphyrin with diphenylacetylene.4,8 According to this procedure, we have synthesized 8a-silyl-7,8-dehydropurpurins by replacement of diphenylacetylene with terminal trimethylsilyl- (TMS-), tert-butyldimethylsilyl- (TBS-), and triisopropylsilyl- (TIPS-) acetylenes. Desilylation reaction of 8a-TMS-7,8-dehydropurpurin led to the first synthesis of an 8a,8b-unsubstituted 7,8-dehydropurpurin.
RESULTS AND DISCUSSION
Initially, we performed Pd-catalyzed [3+2] annulation of NiII meso-bromoporphyrin 1Ni with TMS-, TBS-, and TIPS-acetylenes in the presence of catalytic amount of Pd2(dba)3 and PPh3 (Scheme 1). The reaction of 1Ni with TMS-acetylene afforded the expected 8a-TMS-7,8-dehydropurpurin 3a in low yield (29%) with some side products. In the case of a bulkier TBS group, Sonogashira coupling9 competed with the desired [3+2] annulation to provide TBS-ethynylporphyrin 2b and 8a-TBS-7,8-dehydropurpurin 3b in 23% and 41% yields, respectively. Such competitive Sonogashira coupling has been reported in the Pd-catalyzed [3+2] annulation reactions of 9-bromoanthracene with terminal acetylenes.10 The steric hinderance caused by the bulkiest TIPS group prevented the desired [3+2] annulation to afford a small amount of 8a-TIPS-7,8-dehydropurpurin 3c (9%) and a good yield of TIPS-ethynylporphyrin 2c (66%).
After extensive screening of porphyrin substrates and reaction conditions, we found that utilization of free base meso-bromoporphyrin 1H2 as a starting material and (o-tol)3P as a ligand is more effective for annulation (Scheme 2). The Pd-catalyzed [3+2] annulation of 1H2 with TMS-acetylene followed by nickel metalation furnished 3a in 52% yield as a sole isomer without any alkynylporphyrins. By considering the previously reported reaction of 9-bromoanthracene with TMS-acetylene,10,11 the carbopalladation of porphyrinylpalladium to the C–C triple bond occurred to minimize steric hinderance, providing the 8a-silylated compound selectively. Even in the cases of bulkier silyl groups like TBS and TIPS groups, the [3+2] annulation reactions also proceeded smoothly and gave 3b and 3c in 75% and 66% yields, respectively.
Protodesilylation of 3a with hydrochloric acid in aqueous THF afforded unsubstituted 7,8-dehydropurpurin 4 in 45% yield (Scheme 3). The 1H NMR spectrum of 4 in CDCl3 showed two upfield-shifted doublet signals owing to the two protons at the bridging vinylene moiety at 6.80 and 5.96 ppm (J = 5.5 Hz). In the case of pyracylene, which had dual 10π- and 12π- electronic circuits, the paramagnetic ring current also resulted in relatively high field-shifted signals at 6.01 and 6.52 ppm in CCl4.12 The 13C–1H COSY measurement revealed the 13C signals of the fused five-membered ring at 139.35 and 126.18 ppm (Supporting Information, Figure S13). Unfortunately, compared with the previously reported 8a,8b-substituted 7,8-dehydropurpurin derivatives,3–7 4 was less stable in solution and gradually decomposed to a complicated mixture. Probably, the substituents at the fused five-membered rings kinetically supressed their oxidative decomposition processes despite its inherent instability caused by the contribution of 20π-antiaromatic conjugations.
The UV-visible absorption spectra of 3a–c, 4, and 8a,8b-diphenyl-7,8-dehydropurpurin 54,6 in CH2Cl2 are shown in Figure 1. These absorption spectra have characteristic split Soret bands located at 350–500 nm, almost featureless Q-like bands around 580 nm, and weak and extremely broad bands almost reaching 1000 nm. The absorption spectra of 3a–c and 4 are almost similar because of the exiguous perturbations of the silyl groups to 7,8-dehydropurpurin π-systems. Compared with 5, 4 has slightly blue-shifted absorption bands in the overall region. Although the phenyl groups at the 8a- and 8b- positions of 5 were twisted out of the porphyrin plane,4,6 they could contribute to the π-extension of the 7,8-dehydropurpurin π-system to a small degree.
The electrochemical properties of 3a–c, 4 and 5 were studied by cyclic voltammetry (CV) in CH2Cl2 (Table 1). 8a-Silyl-7,8-dehydropurpurins 3a–c exhibited two reversible oxidation waves and one reversible reduction wave. Unsubstituted 7,8-dehydropurpurin 4 displayed a similar reversible reduction wave, but its first oxidation wave became irreversible. Lack of kinetically protecting groups at fused five-membered ring destabilized the radical cation and triggered off the decomposition of 4. Compared with 3a–c and 4, 5 exhibited a slightly smaller electrochemical HOMO–LUMO gap because of the contribution of phenyl groups to the π-extension.
In conclusion, Pd-catalyzed [3+2] annulation of free base meso-bromoporphyrin 1H2 with three terminal silylacetylenes furnished 8a-silyl-7,8-dehydropurpurins 3a–c. Protodesilylation of 3a with hydrochloric acid resulted in the first synthesis of an unsubstituted 7,8-dehydropurpurin 4. By comparing the characters of unsubstituted 4 and substituted 5, the substituents at the fused five-membered rings of previously reported 7,8-dehydropurpurin derivatives have some contribution to their stability and π-extension.
EXPERIMENTAL
1H NMR (600 MHz), 13C NMR (151 MHz), and 13C–1H COSY spectra were taken on a JEOL ECA-600 spectrometer. Chemical shifts are reported on a delta scale in ppm relative to residual CHCl3 (δ = 7.26 ppm) for 1H NMR and to CDCl3 (δ = 77.16 ppm) for 13C NMR. Spectroscopic grade solvents were used for all spectroscopic studies without further purification. UV-visible absorption spectra were recorded on a Shimadzu UV-3600PC spectrometer. APCI-TOF-MS spectra were recorded on a Bruker micrOTOF instrument using a negative-ion mode. Redox potentials were measured by cyclic voltammetry on an ALS electrochemical analyzer model 660. Preparative separations were performed by silica gel chromatography (Wako gel C-200, C-300, or C-400). Toluene was distilled from CaH2. Unless otherwise noted, materials obtained from commercial suppliers were used without further purification.
Pd-Catalyzed [3+2] Annulation of NiII meso-Bromoporphyrins with Silylacetylenes: The reaction of NiII meso-bromoporphyrin 1Ni with TBS-acetylene is representative: NiII meso-bromoporphyrin 1Ni (30 μmol, 30 mg), Pd2(dba)3 (3.0 μmol, 2.8 mg), and PPh3 (12 μmol, 3.2 mg) were placed in a reaction flask under argon. Toluene (1.5 mL), TBS-acetylene (0.15 mmol, 28 μL), and N,N-dicyclohexylmethylamine (0.15 mmol, 32 μL) were added, and the whole mixture was heated at 110 ˚C for 12 h. The resulting mixture was allowed to cool to ambient temperature, diluted with CH2Cl2, and filtered through a small plug of silica gel with copious washings (CH2Cl2). After removal of the solvent in vacuo, the residue was purified by silica gel chromatography eluting with CH2Cl2/hexane. Recrystallization of the each separated solids from CH2Cl2/MeCN gave 2b (6.9 μmol, 7.4 mg, 23%) and 3b (12 μmol, 12.9 mg, 41%), respectively.
2b: 1H NMR (600 MHz, CDCl3) δ = 9.52 (d, 2H, J = 5.0 Hz, β), 8.83 (d, 2H, J = 5.0 Hz, β), 8.76–8.72 (m, 4H, β), 7.85 (d, 4H, J = 1.9 Hz, Ar-o), 7.84 (d, 2H, J = 1.9 Hz, Ar-o), 7.73 (t, 2H, J = 1.9 Hz, Ar-p), 7.71 (t, 1H, J = 1.9 Hz, Ar-p), 1.49 (s, 36H, tert-butyl), 1.46 (s, 18H, tert-butyl), 1.25 (s, 9H, TBS), 0.48 (s, 6H, TBS); 13C NMR (151 MHz, CDCl3): δ = 149.14, 149.09, 145.21, 143.49, 142.82, 142.75, 139.96, 139.87, 133.37, 132.66, 132.30, 131.43, 128.80, 128.70, 121.90, 121.36, 121.31, 121.10, 106.28, 100.34, 98.51, 35.14 (overlap), 31.84, 31.81, 26.67, 17.39, –4.09 ppm; UV-vis (CH2Cl2): λmax (ε [M−1cm−1]) = 426 (250000), 540 (17000), 573 nm (9600); MS (APCI, negative): m/z = 1068.5941. Calcd for C70H86N458NiSi: 1068.5981 [M]–.
2c: 1H NMR (600 MHz, CDCl3) δ = 9.55 (d, 2H, J = 4.6 Hz, β), 8.82 (d, 2H, J = 4.6 Hz, β), 8.75–8.71 (m, 4H, β), 7.86–7.83 (m, 6H, Ar-o), 7.72 (d, 2H, J = 1.9 Hz, Ar-p), 7.70 (t, 1H, J = 1.9 Hz, Ar-p), 1.48 (s, 36H, tert-butyl), 1.46 (s, 18H, tert-butyl), 1.40–1.36 (m, 21H, TIPS); 13C NMR (151 MHz, CDCl3): δ = 149.12, 149.08, 145.31, 143.48, 142.87, 142.77, 140.01, 139.92, 133.39, 132.64, 132.27, 131.45, 128.71 (overlap), 121.87, 121.37, 121.30, 121.08, 107.51, 98.88, 98.55, 35.15 (overlap), 31.85, 31.82, 19.21, 11.96 ppm; UV-vis (CH2Cl2): λmax (ε [M−1cm−1]) = 427 (270000), 541 (17000), 573 nm (9800); MS (APCI, negative): m/z = 1110.6415. Calcd for C73H92N458NiSi: 1110.6450 [M]–.
Pd-Catalyzed [3+2] Annulation of Free Base meso-Bromoporphyrins with Silylacetylenes: The reaction of free base meso-bromoporphyrin 1H2 with TMS-acetylene is representative: Free base meso-bromoporphyrin 1H2 (30 μmol, 28.6 mg), Pd2(dba)3 (3.0 μmol, 2.8 mg), and (o-tol)3P (12 μmol, 3.7 mg) were placed in a reaction flask under argon. Toluene (1.5 mL), TMS-acetylene (0.15 mmol, 21 μL), and N,N-dicyclohexylmethylamine (0.15 mmol, 32 μL) were added, and the whole mixture was heated at 110 ˚C for 12 h. The resulting mixture was allowed to cool to ambient temperature, diluted with CH2Cl2, and filtered through a small plug of silica gel with copious washings (CH2Cl2). After removal of the solvent in vacuo, the residue was dissolved in toluene (10 mL). After addition of Ni(acac)2•2H2O (60 μmol, 15.4 mg), the mixture was stirred for 2 h at 110 ˚C. After removal of the solvent in vacuo, the residue was purified by silica gel chromatography eluting with CH2Cl2/hexane. Recrystallization of the separated solids from CH2Cl2/MeCN gave 3a (15.5 μmol, 15.9 mg, 52%) of high purity.
3a: 1H NMR (600 MHz, CDCl3) δ = 8.35 (d, 1H, J = 5.0 Hz, β), 8.33 (d, 1H, J = 5.0 Hz, β), 8.10 (d, 1H, J = 5.0 Hz, β), 8.07 (d, 1H, J = 5.0 Hz, β), 8.04 (d, 1H, J = 5.0 Hz, β), 8.01 (d, 1H, J = 5.0 Hz, β), 7.69–7.67 (m, 6H, Ar-o), 7.64 (t, 1H, J = 1.9 Hz, Ar-p), 7.63 (t, 1H, J = 1.9 Hz, Ar-p), 7.62 (t, 1H, J = 1.9 Hz, Ar-p), 7.13 (s, 1H, β), 7.05 (s, 1H, fused five-membered ring), 1.47 (s, 18H, tert-butyl), 1.45 (s, 18H, tert-butyl), 1.43 (s, 18H, tert-butyl), 0.35 (s, 9H, TMS) ppm; 13C NMR (151 MHz, CDCl3): δ = 158.29, 154.73, 149.24, 149.19, 149.17, 148.35, 147.41 (overlap), 146.89, 145.10, 143.79, 143.52, 142.55, 140.11, 139.92, 139.76, 138.18, 135.17, 133.09, 130.93, 129.85, 129.54, 128.93, 128.33, 127.81, 127.26, 126.71, 126.37, 126.02, 121.27, 121.11 (overlap), 120.29, 115.53, 35.11, 35.08 (overlap), 31.80, 31.77 (overlap), –0.92 ppm; UV-vis (CH2Cl2): λmax (ε [M−1cm−1]) = 380 (52000), 427 (61000), 583 nm (4600); MS (APCI, negative): m/z = 1026.5488. Calcd for C67H80N458NiSi: 1026.5511 [M]–.
3b: 1H NMR (600 MHz, CDCl3) δ = 8.37 (d, 1H, J = 5.0 Hz, β), 8.34 (d, 1H, J = 5.0 Hz, β), 8.12–8.09 (m, 2H, β), 8.05 (d, 1H, J = 5.0 Hz, β), 8.02 (d, 1H, J = 5.0 Hz, β), 7.69–7.67 (m, 6H, Ar-o), 7.64 (t, 1H, J = 1.9 Hz, Ar-p), 7.63–7.61 (m, 2H, Ar-p), 7.12 (s, 1H, β), 7.05 (s, 1H, fused five-membered ring), 1.46 (s, 18H, tert-butyl), 1.45 (s, 18H, tert-butyl), 1.42 (s, 18H, tert-butyl), 1.10 (s, 9H, TBS), 0.29 (s, 6H, TBS) ppm; 13C NMR (151 MHz, CDCl3): δ = 158.15, 155.49, 149.29, 149.25, 149.21, 148.94, 148.33, 147.40, 146.89, 145.18, 143.84, 143.55, 142.48, 139.97, 139.81, 138.15, 137.91, 135.15, 133.07, 130.91, 129.90, 129.58, 129.03, 128.35, 127.83, 127.64, 127.32, 126.40, 126.01, 121.30, 121.18, 121.13, 120.35, 115.53, 35.11 (overlap), 31.81, 31.78 (overlap), 26.99, 17.08, –5.63 ppm; UV-vis (CH2Cl2): λmax (ε [M−1cm−1]) = 381 (54000), 427 (63000), 583 nm (4700); MS (APCI, negative): m/z = 1068.5956. Calcd for C70H86N458NiSi: 1068.5981 [M]–.
3c: 1H NMR (600 MHz, CDCl3) δ = 8.38 (d, 1H, J = 5.0 Hz, β), 8.34 (d, 1H, J = 5.0 Hz, β), 8.12 (d, 1H, J = 5.0 Hz, β), 8.09 (d, 1H, J = 5.0 Hz, β), 8.06 (d, 1H, J = 5.0 Hz, β), 8.01 (d, 1H, J = 5.0 Hz, β), 7.69–7.66 (m, 6H, Ar-o), 7.65 (t, 1H, J = 1.9 Hz, Ar-p), 7.64–7.61 (m, 2H, Ar-p), 7.10 (s, 1H, β), 7.08 (s, 1H, fused five-membered ring), 1.46 (s, 18H, tert-butyl), 1.45 (s, 18H, tert-butyl), 1.42 (s, 18H, tert-butyl), 1.40-1.30 (m, 3H, TIPS), 1.28-1.24 (m, 18H, TIPS) ppm; 13C NMR (151 MHz, CDCl3): δ = 158.20, 155.99, 149.69, 149.24, 149.20, 149.15, 148.20, 147.35, 146.84, 145.16, 143.77, 143.48, 142.24, 139.93, 139.76, 138.11, 135.33, 135.12, 133.04, 130.89, 129.91, 129.56, 129.11, 128.35, 127.87, 127.82, 127.25, 126.33, 126.04, 121.27, 121.13, 121.10, 120.29, 115.72, 35.09 (overlap), 31.80, 31.78, 31.75, 19.08, 11.68 ppm; UV-vis (CH2Cl2): λmax (ε [M−1cm−1]) = 381 (57000), 428 (63000), 584 nm (4900); MS (APCI, negative): m/z = 1110.6405. Calcd for C73H92N458NiSi: 1110.6450 [M]–.
Protodesilylation of 8a-Trimethylsilyl-7,8-dehydropurpurin 3a: 8a-Trimethylsilyl-7,8-dehydropurpurin 3a (17.8 μmol, 16.5 mg) was placed in a reaction flask, and dissolved in THF (18 mL) and H2O (2.0 mL). After the addition of conc. HCl aqueous solution (12 M, 2.0 mL), the mixture was stirred at room temperature for 20 min. Then, the reaction was quenched by the addition of a saturated aqueous NaHCO3 aqueous solution, and the product was extracted with CH2Cl2 (3×25 mL). The combined organic layer was washed with brine and dried over Na2SO4. After removal of the solvent in vacuo, the residue was purified by silica gel chromatography eluting with CH2Cl2/hexane. Recrystallization of the separated solids from CH2Cl2/MeOH gave 4 (7.4 μmol, 7.1 mg, 45%) of high purity.
4: 1H NMR (600 MHz, CDCl3) δ = 8.33–8.28 (m, 2H, β), 8.09 (d, 1H, J = 4.8 Hz, β), 8.04–8.00 (m, 2H, β), 7.98 (d, 1H, J = 4.8 Hz, β), 7.67 (d, 2H, J = 1.9 Hz, Ar-o), 7.65 (d, 2H, J = 1.9 Hz, Ar-o), 7.65–7.62 (m, 3H, Ar-o+Ar-p), 7.63–7.60 (m, 2H, Ar-p), 7.08 (s, 1H, β), 6.80 (d, 1H, J = 5.5 Hz, fused five-membered ring), 5.96 (d, 1H, J = 5.5 Hz, fused five-membered ring), 1.44 (s, 36H, tert-butyl), 1.41 (s, 18H, tert-butyl) ppm; 13C NMR (151 MHz, CDCl3): δ = 156.48, 150.95, 149.27, 149.21, 149.11, 148.51, 147.09 (overlap), 144.99, 144.04, 143.63, 142.54, 139.87, 139.68, 139.35, 138.12, 137.64, 135.19, 133.19, 130.99, 129.96, 129.41, 128.31, 128.25, 127.78, 127.49, 126.55, 126.35, 126.18, 121.34, 121.28, 121.11, 120.04, 114.57, 35.07 (overlap), 31.79, 31.78, 31.76 ppm; UV-vis (CH2Cl2): λmax (ε [M−1cm−1]) = 379 (57000), 425 (63000), 581 nm (4700); MS (APCI, negative): m/z = 954.5075. Calcd for C64H72N458Ni: 954.5116 [M]–.
ACKNOWLEDGEMENTS
This work was supported by Grants-in-Aid from MEXT (Nos.: 24106721 “Reaction Integration” and 25107002 “Science of Atomic Layers”) and from JSPS (Nos.: 25220802 (Scientific Research (S)), 24685007 (Young Scientists (A)), 23655037 (Exploratory Research)).
References
1. N. Ono, H. Yamada, and T. Okujima, ‘Handbook of Porphyrin Science,’ Vol. 2, ed. by K. M. Kadish, K. M. Smith, and R. Guilard, World Scientific Singapore, Singapore, 2011, chap. 7, p. 1.
2. Recent reviews: M. G. H. Vicente, L. Jaquinod, and K. M. Smith, Chem. Commun., 1999, 1771; CrossRef S. Fox and R. W. Boyle, Tetrahedron, 2006, 62, 10039; CrossRef N. Aratani, D. Kim, and A. Osuka, Chem. Asian J., 2009, 4, 1172; CrossRef C. Jiao and J. Wu, Synlett, 2012, 171; J. P. Lewtak and D. T. Gryko, Chem. Commun., 2012, 48, 10069; CrossRef A. M. V. M. Pereira, S. Richeter, C. Jeandon, J.-P. Gisselbrecht, J. Wytko, and R. Ruppert, J. Porphyrins Phthalocyanines, 2012, 16, 464; CrossRef V. V. Roznyatovskiy, C.-H. Lee, and J. L. Sessler, Chem. Soc. Rev., 2013, 42, 1921; CrossRef H. Mori, T. Tanaka, and A. Osuka, J. Mater. Chem. C, 2013, 1, 2500. CrossRef
3. Y. Mitsushige, S. Yamaguchi, B. S. Lee, Y. M. Sung, S. Kuhri, C. A. Schierl, D. M. Guldi, D. Kim, and Y. Matsuo, J. Am. Chem. Soc., 2012, 134, 16540. CrossRef
4. A. K. Sahoo, S. Mori, H. Shinokubo, and A. Osuka, Angew. Chem. Int. Ed., 2006, 45, 7972. CrossRef
5. N. Fukui, H. Yorimitsu, J.-M. Lim, D. Kim, and A. Osuka, Angew. Chem. Int. Ed., 2014, 53, 4395. CrossRef
6. A. Nakano, N. Aratani, H. Furuta, and A. Osuka, Chem. Commun., 2001, 1920.
7. S. Fox and R. W. Boyle, Chem. Commun., 2004, 1322; CrossRef D.-M. Shen, C. Liu, and Q.-Y. Chen, Chem. Commun., 2005, 4982; CrossRef D.-M. Shen, C. Liu, and Q.-Y. Chen, J. Org. Chem., 2006, 71, 6508; CrossRef S. Hayashi, Y. Matsubara, S. Eu, H. Hayashi, T. Umeyama, Y. Matano, and H. Imahori, Chem. Lett., 2008, 37, 846; CrossRef G. Bringmann, D. C. G. Götz, T. A. M. Gulder, T. H. Gehrke, T. Bruhn, T. Kupfer, K. Radacki, H. Braunschweig, A. Heckmann, and C. Lambert, J. Am. Chem. Soc., 2008, 130, 17812; CrossRef T. D. Lash, B. E. Smith, M. J. Melquist, and B. A. Godfrey, J. Org. Chem., 2011, 76, 5335; CrossRef A. M. V. M. Pereira, M. G. P. M. S. Neves, J. A. S. Cavaleiro, C. Jeandon, J.-P. Gisselbrecht, S. Choua, and R. Ruppert, Org. Lett., 2011, 13, 4742; CrossRef T. Ishizuka, Y. Saegusa, Y. Shiota, K. Ohtake, K. Yoshizawa, and T. Kojima, Chem. Commun., 2013, 49, 5939; CrossRef N. Fukui, W.-Y. Cha, S. Lee, S. Tokuji, D. Kim, H. Yorimitsu, and A. Osuka, Angew. Chem. Int. Ed., 2013, 52, 9728. CrossRef
8. Reviews: H. Shinokubo and A. Osuka, Chem. Commun., 2009, 1011; CrossRef S. Hiroto, S. Yamaguchi, H. Shinokubo, and A. Osuka, J. Synth. Org. Chem. Jpn., 2009, 67, 688; CrossRef H. Yorimitsu and A. Osuka, Asian J. Org. Chem., 2013, 2, 356.
9. K. Sonogashira, Y. Tohda, and N. Hagihara, Tetrahedron Lett., 1975, 50, 4467; CrossRef K. Sonogashira, J. Organomet. Chem., 2002, 653, 46; CrossRef E.-i. Negishi and L. Anastasia, Chem. Rev., 2003, 103, 1979; CrossRef R. Chinchilla and C. Najera, Chem. Rev., 2007, 107, 874. CrossRef
10. H. Dang and M. A. Garcia-Garibay, J. Am. Chem. Soc., 2001, 123, 355. CrossRef
11. J. D. Wood, J. L. Jellison, A. D. Finke, L. Wang, and K. N. Plunkett, J. Am. Chem. Soc., 2012, 134, 15783. CrossRef
12. B. M. Trost and G. M. Bright, J. Am. Chem. Soc., 1967, 89, 1967; CrossRef H. P. Diogo, T. Kiyobayashi, M. E. M. Piedade, N. Burlak, D. W. Rogers, D. McMasters, G. Persy, J. Wirz, and J. F. Liebman, J. Am. Chem. Soc., 2002, 124, 2065. CrossRef